Ocean Currents
If the waters of the oceans were not kept in vigorous circulation by the various forces discussed elsewhere, they could support only a small fraction of the marine life that actually now exists. First of all, the amount of primary food produced by plants would be so curtailed by exhaustion of the essential nutrients in the euphotic zone that only a few animals could find nourishment. The slow diffusion of gases and toxic wastes that could take place would set shallow vertical limits of distribution to all forms of life except certain anaerobic bacteria. The northern and southern boundaries of faunal zones dependent on temperature or light would coincide with parallels of latitude.
But the ocean waters are not stagnant. Vertical circulation extending to great depths occurs, and greater or smaller masses of water flow continuously in systems extending in diverse directions over vast areas, irrespective of latitude. Far to the north along western continental shores in the Northern Hemisphere the currents convey heat that has been stored in the waters during their passage through warm, tropical regions. Similarly, along the eastern continental shores cold water masses are carried to the south by currents of arctic or subarctic origin, causing isotherms to streak obliquely across the oceans in a general northeasterly direction. Systems of similar character and magnitude exist in the Southern Hemisphere (Charts II and III).
Water movements provide a means of transportation for organisms which, when combined with temperature and other factors, may control the limits of propagative and sterile distribution of both pelagic and benthic life. The character of the fauna and flora is thus governed by the nature of the currents—namely, their origin, direction of flow, magnitude, coldness or warmth, degree of salinity or density, and their character as regards other attributes such as relative richness in nutrients.
We have just reviewed some of the influences of temperature on animal life. Some of these influences are inseparable from ocean currents, for temperature is usually an index of flow. With this in mind, we shall now endeavor to examine more closely some features wherein water movements are of far-reaching biological significance.
Significance of Currents in Dispersal and Maintenance of Populations. Perhaps the most obvious importance of currents on marine life results from its direct influence on dispersal. It has already been pointed out (p. 315) that nearly all benthic animals in the littoral zone possess free-swimming or floating eggs and larvae. The significance attached to this type of life history in any given species must be that it provides a means of taking advantage of water movements to bring
about and maintain as wide distribution as possible, so that all environmental facies favorable to the life requirements of the adult both for spawning and vegetative growth can be inhabited. During this dispersal, countless thousands of larvae are carried into areas where they are unable to live or where at desertion of the pelagic life the sessile stages find conditions unsuitable with respect to depth of water, substratum, chemical-physical character of the water, or such other factors as food and enemies. This, however, is the price paid for the widest possible distribution.
Vast numbers of young are accordingly produced by each individual in order to overcome the hazards of the temporary pelagic existence (see p. 316). By far the largest number of larvae of benthic forms are eaten by predacious animals or succumb as a result of settling in inimical environments at the time of metamorphosis and desertion of the pelagic habit. That great numbers, however, do find favorable settlement is witnessed by extensive populations of benthic animals. Certain areas may be quite suitable to maintain a population but this population may be unable to restock to any appreciable extent from its own spawning, owing to the prevailing currents carrying the eggs and larvae completely away from the area and into other situations where settlement may or may not occur. These areas cannot be considered as areas of sterile distribution for reproduction does take place and larvae can survive, but because of circumstances associated with currents the areas must be regularly restocked by incoming larvae from elsewhere. Most benthic populations, perhaps, experience to some degree such renewal of stock by larvae produced elsewhere, and in turn they contribute their own larvae to restock other areas. Oscillating currents related to tides within a bay or fjord function to reduce loss of larvae and to maintain a degree of independence for its population.
When the larvae of benthic forms have survived to settle in a favorable habitat, the new stage of life is still dependent upon currents to maintain tolerable living conditions with respect to aeration, dispersal of toxic wastes, and supply of planktonic food. To coastal animal communities where the temporary plankton element is conspicuous, coastal circulation resulting from more or less local conditions such as tides and winds is of especial importance; but we shall see that larger and more permanent current systems also affect the lives of members of the temporary plankton and their adult stages.
As illustrative of the role of currents in the life of coastal benthic animals we refer to the study of Emerita analoga (Johnson, 1939b), the sand crab which in the benthic state inhabits sandy beaches in the intertidal zone. The female incubates the eggs, which remain attached to body appendages until the first zoeal larval stage. Then the larvae are freed to seek food and to face the regular hazards of enemies and possibly
The distance to which these or other temporary larvae can be transported depends of course upon the speed of the currents, the adaptability of the larvae, and especially upon the normal length of pelagic larval existence. In Emerita the larval stage is of long duration, being a matter of about four to five months and resulting in transport seaward at least 200 miles and doubtless much greater distances in coastwise currents. In the oyster the larval stage is shorter, being only about two to four weeks, depending upon temperatures. Yet oysters are known to extend their range of distribution at least 30 miles in one season through drift of the pelagic larval stage (Elsey and Quayle, 1939).
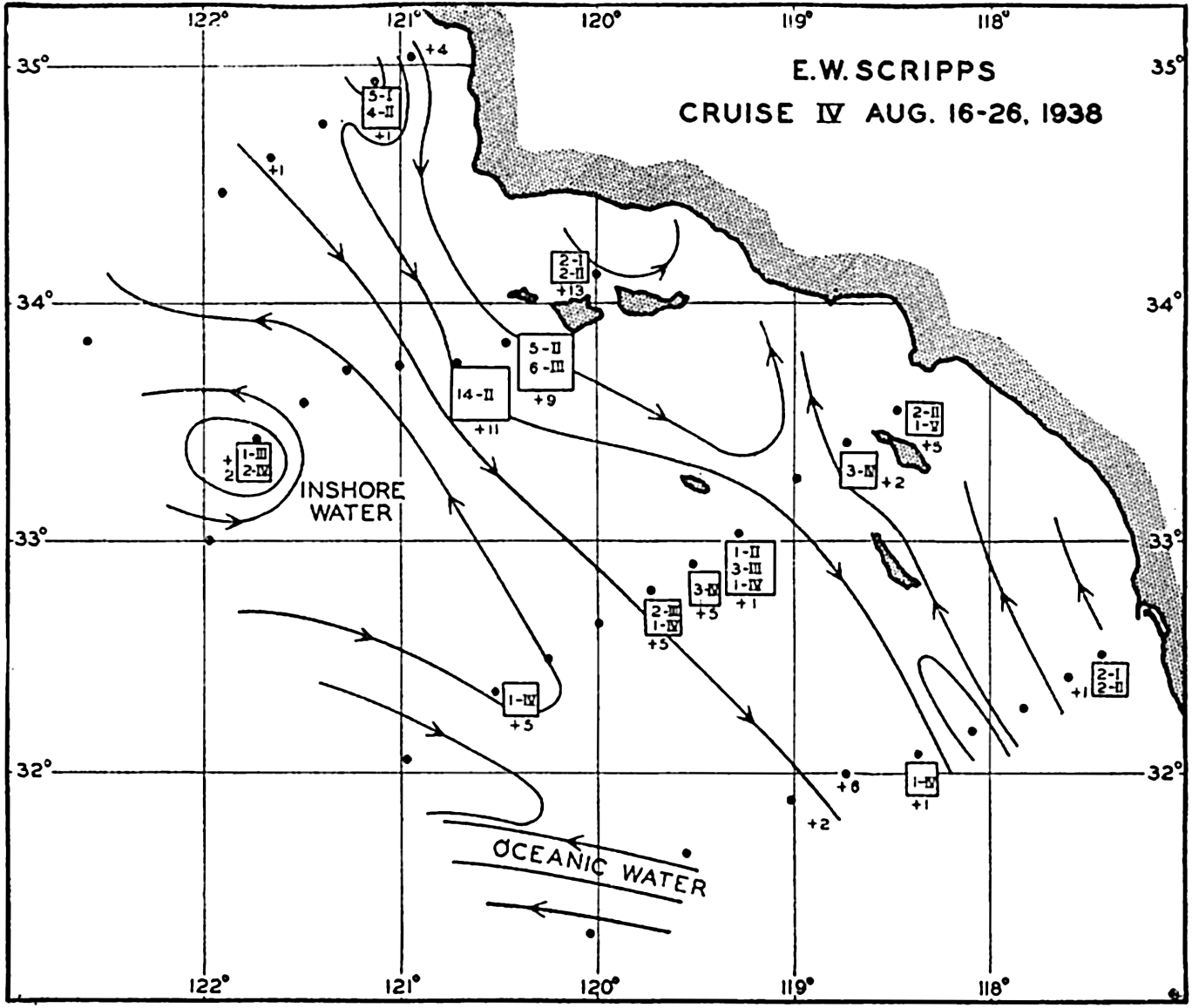
The dispersal of larval stages of Emerita analoga and other littoral crustacean larvae. Figures within the squares indicate the number and zoeal stages of Emerita; the symbol + followed by a number indicates the number of unidentified crab larvae. The character of the currents is indicated by the lines with arrows. (From Johnson.)
The great stretch of open water between the west coast of Central America and the easternmost Polynesian Islands is considered an effective
The depth at which passive eggs of the temporary plankton are carried by water currents depends to a great extent on their specific gravity in relation to that of the waters wherein they were spawned or to which they may rise (Walford, 1938). It was found (Thompson and Van Cleve, 1936) that halibut eggs occur in the denser waters some distance offshore at the outer edge of the spawning banks in depths of 100 to 200 m, though occasionally at only 40 m and at other times as deep as 935 m. The eggs were completely absent in shallower net hauls near the surface in waters with a low salinity and low specific gravity. From these facts it appears that they drift with the slowly moving deep currents. The newly hatched halibut larvae are found outside the continental shelf and at depths over 200 m. As the larvae develop, however, they rise to the upper layers until at the age of three to five months all of them are found at depths of 100 m or less. With the rise to the surface they are carried by inshore currents into shallow Alaskan bays, where they are found on the bottom. The cycle requires about six or seven months for completion. In the southern range of the northern halibut the powers of recuperation of the overfished stock are apparently less than in the stock of the Alaskan area, owing to a more unfavorable dispersal of the pelagic eggs by offshore or southward-moving currents in the southern area (Thompson, 1936).
Perhaps the best known example of transoceanic transportation of organisms is found in the investigations of Johannes Schmidt (1925a), dealing with the life history of the European eel Anguilla vulgaris. Full-grown and submature eels inhabit fresh-water streams and lakes, but upon approach of complete sexual maturity in autumn they desert these waters and disappear into the sea to spawn. The destination of the eels during these spawning journeys was for centuries one of the deepest mysteries of the sea. The young, recently metamorphosed eels, known as elvers, (about 6 or 7 cm long) have long been known to occur in the European coastal waters where in spring they enter fresh water in great numbers, but it was not known where in the sea they had originated. Strange theories were advanced; for example, Pliny and Aristotle believed they arose from mud of the sea bottom. Only in the last few decades have these mysteries been solved. Not until 1896 was it shown (Grassi, 1896) that a strange ribbonlike little fish, considered since 1856 as a separate species called Leptocephalus brevirostris, was in reality an eel developmental stage which through metamorphosis becomes the elver.
With this information at hand Johannes Schmidt in 1904 began investigations which extended over many years, involving examination
The American eel Anguilla rostrata breeds in the same or adjacent waters. Its life history requires only two years of larval life, for although the bulk of larvae migrate for a period in the same current as the European larvae, upon nearing the North American coast they become segregated from the European larvae, which are destined to continue slowly onward to the east.
The animals belonging to the permanent plankton may also be transported by currents over great distances, and this is particularly true with respect to the main oceanic currents. If the courses be sufficiently long or circular, the animals will successfully propagate while en route. Successful propagation is indicated when all stages of development of a given species are found in the transporting waters. Usually, however, the waters change their character as regards temperature, salinity, and so forth, or they may merge with other waters and lose their identity entirely or in part. In this case many of the animals that are being transported may die. Some of the hardier species frequently survive for some time and may even spawn, but the young are unable to withstand the environment unnatural to them (see p. 863). In this event the plankton catches will show only adult or submature stages, sometimes eggs and a few early stages, but few if any of the intermediate ones. Restocking must then take place regularly or periodically in successive invasions from the outside. The presence of these exotic animals is living evidence of the source of the water, provided the normal habitat of the species is known. Gulf Stream species are classical examples of planktonic animals that appear as visitors in localities where they do not normally breed. Illustrative of this are certain salps and the tropical copepod Rhincalanus nasutus occurring off the coast of Norway. Other examples are found in the northern penetration of R. nasutus and also
On the west Atlantic coast a number of Gulf Stream forms are swept by offshoots of the main current into the Gulf of Maine. They may survive there for varying periods of time, although the temperature and salinity are much reduced by mixture with coastal waters. Bigelow (1926), by a study of plankton catches, has determined the point of entrance of these immigrant forms and has traced their course after entering the Gulf and mingling with the endemic plankton community. They enter the Gulf at three levels and show varying degrees of success in establishing themselves, depending upon their species and adaptability. It is found, for example, that Sagitta serratodentata is a very hardy warmwater visitor that survives long enough to complete a good portion of the large counterclockwise gyral within the Gulf, and it may even grow to a larger size than in its native Gulf Stream habitat, although it cannot reproduce with sufficient success to establish itself as endemic. Adults may live for a time in waters with temperatures as low even as 3.9°C but do not survive the winter in the invaded area. Redfield and Beale (1940) have verified Bigelow's conclusion (1926) that this species does not reproduce in the Gulf of Maine. Because of this sterile distribution the animals are known as terminal immigrants. Their periodic occurrence depends upon periodic changes in the flow of outside water into the area. Sagitta maxima enters the Gulf in the deep layers and becomes dispersed in the deeper part where salinity is highest. Rhincalanus cornutus and R. nasutus are also stray tropical and oceanic visitors in the Gulf.
Arctic forms are also found in the Gulf of Maine, giving evidence of the penetration of the southward-flowing cold Nova Scotian water past Cape Sable into the Gulf. Among these cold-water strays are included Calanus hyperboreus, the pteropod Limacina helicina, and the appendicularian Oikopleura vanhoffeni.
The pattern of horizontal flow is a determining factor not only in the widespread dispersal of exotic plankton elements from their centers of production, but also in maintaining an endemic population. The effect of oscillating tidal currents with a minimum residual flow was mentioned above as contributing to the maintenance of an endemic stock in restricted areas. Many partially landlocked bays maintain a characteristic population through such action. The semiclosed lochs of the Clyde Sea area, for example, have proved favorable regions for scientific study of local population cycles without too great an exchange with biological elements produced elsewhere and carried into the lochs (p. 903). But certain types of nontidal current systems are also effective in supporting a self-sustaining population of specific forms. Such are the eddies of
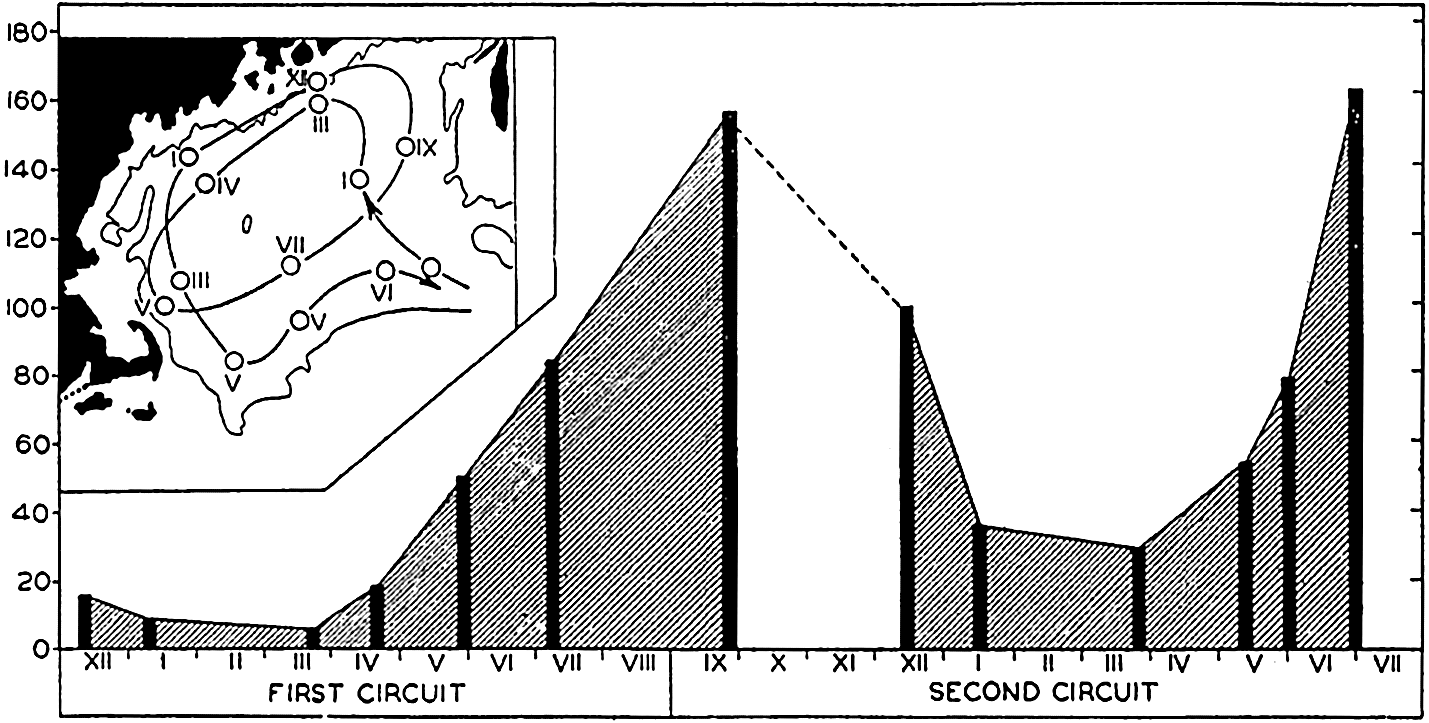
The growth of population in a mass of water assumed to move along the course indicated in inset. Ordinate: volume of zooplankton in cubic centimeters per square meter of sea surface; abscissa, time in months. The black bars indicate the volumes caught at the selected stations. The position of these stations and the month of collection are indicated on the inserted chart. (From Redfield.)
Damas (1905) and Sömme (1934) have discussed the importance of such currents in maintaining a breeding stock for pelagic populations in the Norwegian Sea. The Gulf of Maine on the American coast offers an excellent example of the biological effect of this type of circulation. Redfield (1941), carrying forward the earlier analyses of Bigelow and of Huntsman, concludes that the great cyclonic eddy of the basin of the Gulf is a vital factor in maintaining sufficient breeding stock to perpetuate an endemic calanoid population (Calanus finmarchicus and related forms) in that area and also to contribute to other neighboring waters. The regular recurrence of a closed eddy during the summer provides a tolerably uniform environment permitting the endemic species to complete their whole life cycle from egg to spawning adult while being swept involuntarily along in the moving mass of surface water wherein the bulk of the animals occur. Figure 236, from Redfield, shows how the shift of population center was followed in its drift around the Gulf. In chapter IX we learned that the rate of breeding of C. finmarchicus permits of two or three generations in a year. The rate of flow of water within the Gulf is about seven miles per day and requires about three months to make the complete circuit, thus allowing considerable time for development. In early winter there is an indraft of relatively barren water from the northeast, and also during this time the center of the endemic population is being forced gradually to the southwest, but in
A cyclic circulation resulting in a flow of water around an island may also lead to maintenance of an endemic stock. A good example depicting this is the investigation of the drift of eggs and larvae of gadoid fishes around Iceland (Schmidt, 1909). Hydrographic investigations show a clockwise current which flows around the island at a speed just sufficient to transport the developing eggs and larvae passively from the spawning ground, located on the south and west coasts, to the north and east coasts where the advanced larvae seek the bottom to continue growing after their coastwise journey of several hundred miles in the plankton. The motility of the older fish is also a factor in maintaining the local stock, but any circuit of this nature must be a particularly significant factor in maintaining an endemic population of benthic life and of the general plankton as well.
Finally, the biological implications resulting from a difference in rate of flow of surface and subsurface water masses in any given area should be mentioned. Planktonic animals that inhabit deep water and that perform no vertical migrations must cross into other water masses less frequently than those making vertical diurnal migrations. Periodically the latter will become segregated horizontally from the former when their journey takes them into waters with different rates of flow. Different stages of development or even the sexes may experience some degree of segregation from this cause, since it is known that the adults and juveniles, as well as the sexes of some species, do not all migrate at the same rate (p. 837). Hardy (1935) has shown how vertical migrations and the dynamics of distribution may function to segregate phytoplankton and zooplankton swarms (p. 900).
Certain areas of slowly moving water at depths of 300 m or more are held responsible for the maintenance of a stationary winter population of Calanus finmarchicus in the Norwegian Sea (Sömme, 1934). If these animals were to winter in the surface waters instead of descending to greater depths, they would become dispersed by the more rapid surface currents and thus dilute the breeding stock for spring production.
Indicator Species. From what has been said of the far-reaching biological influences of water movements, it becomes obvious that the intelligent interpretations in any marine ecological investigation must be based in part on data which provide a reliable picture of ocean currents both locally and in general. Descriptions of current systems and discussions of methods for observing or computing currents are given elsewhere,
We have seen that many animals are carried passively by currents into areas other than those in which they normally live and breed. If now we consider the problem conversely, these exotic forms whose developmental history, habits, and source are known become invaluable indicators of the source of the currents or water masses in which they are found.
A review of the voluminous literature bearing upon this subject is best obtained in recent special publications (Russell 1935a, 1935b, 1939). As examples of indicators we need here only refer back to the forms used in illustrating the dispersal of animals by currents. It must be born in mind that for most reliable use of indicator species the following pre-requisites are necessary:
Exact identification of the species or varieties of organisms involved must be possible.
Their propagative and sterile distribution must be satisfactorily known.
The morphological stages of development in the life history must be known for the species or must be deducible from what is known of the group to which they belong. In this connection the time and depth at which spawning occurs and the duration of the various stages are important. Passive eggs and larvae may be adjusted in specific gravity to heavy or light water layers and therefore transported mainly in layers of corresponding density (p. 861).
It should be known to what extent ecological adjustment can be made by migrations into deeper or shallower water in the extended geographical distribution. For example, Calanus hyperboreus and Parathemisto oblivia, arctic species, may live in deep cold water in lower latitudes and by vertical migration may appear also in surface layers (Gran, 1902).
A clear distinction must be made between animals brought in from outside geographical areas and those of biological groups that develop locally when conditions simulate those of the outside geographical area, especially with respect to temperature (see p. 793).
Associations of organisms transported from a common center of distribution are of more value than occasional strays. Such associations may be expected to be found especially among the euryhaline-stenohaline neritic forms occurring in offshore situations, indicating seaward drift of coastal waters. Pelagic larvae of intertidal benthic species may be of special value if the duration of the various larval stages is sufficiently well known to supply information not only on distance of drift but also
― 867 ―on the time involved (fig. 235). These larvae thus serve as myriads of tiny drift bottles released along the coast.
Species Development. Indirectly, currents must have played an important role in the development of species. Through the ages, the currents have persisted in the dispersal of marine animals and many euryhaline and eurythermic species have become cosmopolitan. However, distributional limits are usually much more narrow for most animals since the scattering effect of currents must constantly work in opposition to the limiting effects of other factors and in particular to those of temperature, salinity, and light. It appears that the persistent pressing by currents of both the holo- and meroplanktonic forms into regions deviating from the optimal living conditions has had profound effects on the character of the fauna established in the more adverse regions. The less tolerant species are eliminated and certain genetic strains adapted to the more selective conditions are developed. It is generally believed that species-producing centers occur in the temperate or warm stable waters of the tropical belt. Strongly indicative of this is the correlation between temperature and the number of species of Tintinnoinea (Kofoid, 1930). Of the 705 known species of the suborder, 8 are restricted to fresh water, 59 to the Arctic seas, 42 to the Antarctic, and 515 to the warm temperate and tropical oceans. Though the polar faunas are not so fully known as the tropical, the ratio of cold- to warm-water species appears to be one to five.
Not merely species but many genera and even higher systematic groups are confined entirely, or nearly so, to the tropical region (Ekman, 1935). From these rich centers temperate and cold-water species have seemingly evolved through the process of selection at border regions where conditions become farther and farther removed from the optimum for stenothermic warm-water forms. The more rigorous living conditions of bordering areas have allowed only relatively few species to become established compared to the abundant fauna of tropical waters. This relation is brought out also for certain of the higher pelagic animals in table 99, adapted with modifications from a summary of the better-known holoplanktonic groups which was compiled from various specialists by Russell (1935b). The numbers given are not to be considered final, for it is certain that many species have not yet been recorded or are not included, but they are sufficiently complete to illustrate the preponderance of species in warm areas and to indicate other relative features to which reference will be made later.
The greater number of planktonic species in the antarctic region than in the arctic may be associated with the far greater area of confluence between the warm and cold waters in the Southern Hemisphere but, in addition, the general oceanic currents of the Atlantic appear to play a part, especially for the cold-water copepods (Russell, 1935b).
Cold-water species evolving outward from warm-water surface species may do so by dispersal either in a northerly or southerly direction, and also by submergence into the deep cold water of the tropics where deepwater genera and species are formed. The deep-water current system in the Atlantic (p. 747) favors the carrying of these deep-water forms, as well as those of the Arctic, toward the antarctic surface region (p. 620). That the antarctic and subantarctic copepod population has a closer connection with deep-sea genera is shown by the fact that in the Antarctic and Subantarctic 18 species belong to deep-sea genera (that is, genera in which 50 per cent or more of the species in a genus may be regarded as belonging to the deep sea), as opposed to only 3 species belonging to deep-sea genera in the Arctic and in boreal waters. Species that are bipolar-epiplanktonic (p. 850) are representatives mainly of surface genera (that is, genera in which less than 50 per cent of the species in a genus can be regarded as deep-sea species). Judged by these standards, 5 species of bipolar-epiplanktonic copepods are among surface genera and only 1 species from deep-sea genera. The extent to which bipolar-epiplanktonic species can develop apparently depends largely on the ability of northern cold-water species to withstand tropical submergence.
Animals | Arctic | Arctic-Boreal and Boreal | Antarctic | Subantarctic | Bipolar-epiplanktonic | Cosmopolitan | Warm water | Deep sea | Total | |
---|---|---|---|---|---|---|---|---|---|---|
( ) = Bipolar subarctic and subantarctic. | ||||||||||
Coelenterata | ||||||||||
Trachy- and Narcomedusae | 4 | 10 | 3 | 6 | 90 | 21 | 134 | |||
Siphonophora: Calycophorae. | 2 | 1 | 64 | 5 | 72 | |||||
Physophorae. | 1? | 2 | 29 | 2 | 34 | |||||
Ctenophora. | 1 | 2 | 2 | 1 | 2 | 69? | 3 | 80? | ||
Nemertea | 52 | 52 | ||||||||
Polychaeta | ||||||||||
Tomopteridae | 1? | 4 | 1 | 38 | ? | 44 | ||||
Chaetognatha. | 2 | 2 | 2 | 18 | 6 | 30 | ||||
CRUSTACEA | ||||||||||
Cladocera. | 1 | (3) | 3 | 7 | ||||||
Copepoda. | 7 | 10 | 32 | 5 | 1(5) | 10 | 489 | 195 | 754 | |
Amphipoda | ||||||||||
Hyperiidea | 2 | 10 | 2 | 250 | 28 | 292 | ||||
Gammaridea. | 7 | 20 | 17 | 44 | ||||||
Euphausiacea | 6 | 2 | 7 | (1) | 46 | 23 | 85 | |||
Mollusca | ||||||||||
Pteropoda | ||||||||||
Thecosomata. | 2 | 1(1)? | 44 | 3 | 51 | |||||
Gymnosomata | 1 | 2 | (1)? | 37 | ? | 41 | ||||
Heteropoda. | 90 | 90 | ||||||||
Tunicata | ||||||||||
Appendicularia. | 2 | 1 | 6 | 1 | 48 | 3 | 61 | |||
Thaliacea | ||||||||||
Doliolidae | 1? | 11 | 12 | |||||||
Salpidae | 1 | 24 | 25 | |||||||
Pyrosomidae. | 8 | 8 | ||||||||
Total | 23 | 35 | 71 | 19 | 17 | 15 | 1378 | 358 | 1916 |
The greater number of species in the antarctic region over the arctic is also evidenced by littoral benthic life. But here the reason seems to be an historic one and not a matter of pelagic larvae or adults being transported thence by currents, for the Antarctic Continent is an isolated one and an extraordinarily large endemic population exists on its shores. Whatever the reason may be, it is of interest to note that, though warm or temperate waters appear favorable to species development, yet paradoxically the Antarctic possesses a littoral fauna richer in species than the Arctic shores of the Northern Hemisphere although the antarctic environment is colder than the arctic.