BIOLOGICAL FACTORS INFLUENCING MOVEMENTS AND CONCENTRATION OF ORGANISMS
From what has just been said concerning the interrelations of organisms, it is obvious that in a study of the combination of factors controlling the whole or a given portion of the population it is necessary to consider seriously not only physical and chemical factors but also biological factors that have direct bearing on the lives of the individuals of the population. These factors are associated chiefly with feeding but there may be other less obvious influences. We have already alluded to the abundance of plant production in the coastal waters and to the inanimate factors making for high productivity. The direct corollary is the abundant animal life of the coastal regions as opposed to the more sparse population of the waters far from shore.
Phytoplankton—Zooplankton
In attempting to evaluate the importance of the ecological relationships of natural biological groups in the sea as a whole, we are forced to
Organisms making up the plankton are usually short-lived, particularly the photosynthetic organisms since these are notably sensitive to changes in the physical-chemical living conditions. Seasonal and sometimes interseasonal periods prevail, therefore, when but little phytoplankton can be found; but, at least in temperate regions, a vernal production followed by subsidiary increases of phytoplankton can be depended upon each year. This vernal production of phytoplankton must be considered as an event of great significance, for it comes at a time coinciding with abundant production of pelagic larvae, especially of invertebrates, that feed upon it (see below). Any factor, whether it be in reference to phytoplankton food or to inorganic living conditions, that hampers the success of these swarms of larvae will function immediately to the disadvantage of higher plankton feeders that utilize the larvae directly or the adult population resulting therefrom. Studies have indicated that among these feeders may be placed many commercial fishes, especially the young, but also the adults. Any degree of failure of the spawning or development of larvae of the permanent plankton (of which copepods may be considered typical) must also be reflected only a few weeks later in the adult stock available in any area, for the adults are short-lived and apparently die after the spring and summer propagative periods and therefore depend upon the success of these broods to maintain the adult stock at a high numerical level. Copepods, we know, feed upon the phytoplankton and in turn constitute an important item in the diet of many fishes. Assuming that phytoplankton production may be correlated with abundance of light, an example of the far-reaching effect of phytoplankton production on the mackerel is offered in a study by Allen (1909), which shows a direct correlation between the abundance of fish caught during May and the total hours of sunshine for the preceding February and March, over a period of seven years (fig. 243). Official figures for the mackerel fisheries as a whole show a marked drop in 1906, and Bullen (1909) has shown a
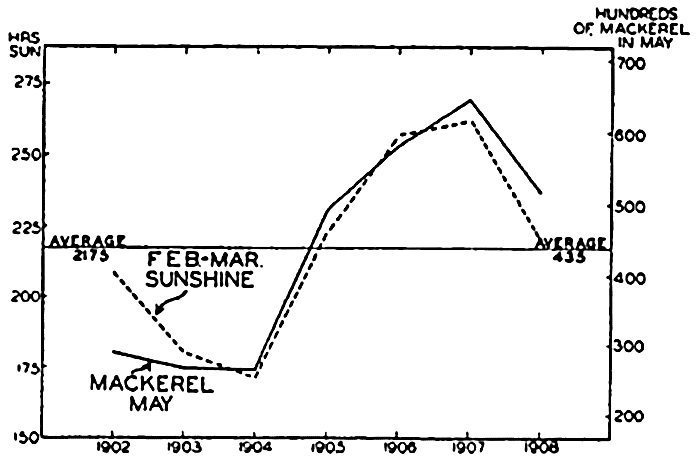
Correlation between sunshine and the abundance of mackerel. (From Allen).
Many investigators have observed that during the growing season when diatoms occur in abundance there frequently appears to be a scarcity of zooplankton. A combination of causes doubtless operates to produce this effect. Two main hypotheses have been advanced in explanation of the phenomenon of inverse relations. The least complicated explanation is found in the hypothesis of grazing (Harvey, 1934), which holds that diatom numbers are controlled through consumption by animals. The alternate hypothesis is that of animal exclusion, advanced by Hardy (Hardy and Gunther, 1935; Hardy 1936).
Animal Exclusion. According to this view, which involves a consideration of the regular vertical diurnal migrations of the zooplankton, certain of the animals during a part of the day swim upward into the layer of water where diatoms are being produced. The duration of their sojourn in the upper layer is inversely related to the concentration of the phytoplankton. Thus they are excluded vertically for a considerable period of time when diatom production has resulted in a dense swarm of diatoms. It should be noted that this exclusion is primarily a vertical one; nevertheless it may result in lateral exclusion when there is a difference in the speed or direction of flow of the upper euphotic layer and of the deeper layer into which the animals have descended and in which, when the diatoms are very abundant, they may spend a disproportionately long time. A lateral displacement may therefore be more or less complete and the greatest concentration of animals will finally occur in areas where diatoms are relatively scarce. The implications of this hypothesis on the habit of diurnal migrations should be noted (p. 836).
As evidence in support of this hypothesis, it was found during the Discovery investigations in the Antarctic that the greatest concentration of zooplankton occurs in areas low in phytoplankton content and relatively high in phosphate content, which indicated that phytoplankton production had been low for some time. Correlated with this disparity was the fact that blue and fin whales, which are known to seek out and feed upon zooplankton, were present in greatest numbers in the areas rich in phosphates. Some support is also given through experiments which indicate that some animals are more strongly negatively phototropic in the presence of many diatoms (Lucas, 1936). Plankton animals exhibiting the most pronounced activity in diurnal vertical migrations are the ones most likely to be excluded according to the hypothesis. Young stages are believed to show less tendency to exclusion. In the hypothesis of animal exclusion, which is considered as a tentative one, the emphasis is placed on the inimical effect (presumably chemical) of plants on the animals.
Grazing. The concept in this hypothesis shifts the emphasis to the effect of the grazing zooplankton as a control of phytoplankton production. That grazing is a highly important factor in the control of phytoplankton is given much support by various investigators (Harvey et al, 1935, Fuller, 1937).
According to this point of view, large phytoplankton and zooplankton populations cannot exist simultaneously in the same area for long, since the extensive grazing by the zooplankton prevents the phytoplankton from building up or maintaining dense growth. As a result of investigations of Harvey et al (1935) it is indicated that in general “a change in diatom population is brought about by a change in one or both of two opposing factors—the rate of growth of the diatoms (depending upon illumination and probably on concentration of nutrient salts) and the rate at which the diatoms are eaten (depending upon the number and kind of herbivorous animals).” Therefore, in any area a dense phytoplankton population is the result of optimum growing conditions combined with a relative scarcity of grazing animals, the yield of plant cells having been greater per unit time than the consumption by animals plus the loss that may result through other causes. Since dense plant growth is favored in the absence of grazers it appears as if the animals have been excluded because they avoid a dense diatom population. Evidence of grazing is found in the fact that phytoplankton may “disappear” when nutrients and other growing conditions are good.
In this connection it is significant to note that computations indicate that during maximum grazing plankton animals may consume somewhat less than half their own weight per day. The copepod Eurytemora hirundoides is said to eat as many as 120,000 small diatoms (Nitzschia closterium) in a day (Harvey et al, 1935). Lohmann (1908), in an exhaustive study of plankton at Kiel, assumed that each metazoan animal requires a daily ration of one tenth its own volume. A 30 per cent daily increase in plants was assumed to take place, and only this much could then be removed without reducing the initial plant stock by overgrazing. As stated elsewhere (p. 885), calculations on this basis showed a plant deficiency during the seasons of low plant production. As a matter of fact, the rate of increase in numbers of plants cannot be so simply stated. It varies greatly and so also does the rate of feeding in grazing animals, and widely different combinations of plants and grazers must occur.
Evidence has been brought to indicate that some plankton filter-feeding animals, that is, copepods and mysids, filter the water at a rather constant rate regardless of the concentration of the microscopic particulate food that is present (Lucas, 1936, Fuller and Clarke, 1936, Fuller, 1937, Fleming, 1939). Other factors, such as light, temperature, and size of particles, function to vary the filtering rate (Fuller, 1937). This
Time in days | Population (cells per liter) | ||
---|---|---|---|
Grazing element doubled | Grazing element increased fivefold | ||
0 | 1,000,000 | 1,000,000 | |
1 | 487,000 | 62,000 | |
2 | 237,000 | 3,900 | |
3 | 106,000 | 240 | |
4 | 56,000 | 15 | |
5 | 27,000 | <1 |
When plants have been reduced through grazing or when conditions for growth of the plants become less favorable and the division rate diminishes to a point where production of new cells is less than the number consumed by the grazers, animals again dominate the field. The progressively diminishing efficiency in catching plants as they become scarce is important to the survival of the diatoms since as a result there is likely always to be some “seed” left in the water. It is important to note that observations taken at fixed places, in areas where an exchange of water masses occurs, may show an apparent succession of phyto- and zooplankton populations. However, under such conditions no true alternation has occurred but only an apparent one owing to the exchange of water within the area under observation. Investigations of food relations in the plankton can, of course, be most reliably carried on in bodies of water such as bays or other closed systems that are sufficiently isolated to experience little or no influence from inflow or outflow from adjacent waters and therefore support an endemic self-contained population. In open coastal areas where much exchange of water occurs, the details
Some investigations do, nevertheless, show a clear relation between phytoplankton increases and the dependent plankton animals. For example, in Loch Striven, a semienclosed area, Marshall, Nicholls, and Orr (1934) were able to correlate directly three main successful broods of Calanus finmarchicus with diatom increases in (1) March-April, (2) May, and (3) July and August-September, the main spawning having occurred in February-March, May, and July. A fourth period of spawning occurred but was abortive owing to scarcity of food for the early stages of development. There were also indications that a storage of fat can take place during periods of plentiful phytoplankton.
Nielsen (1937) found that in the open coastal waters of Iceland the phytoplankton maximum occurred in May. In these waters the zooplankton was poor and was represented mainly by juvenile individuals, while at the same time in the protected fjords, where diatom maximum came a month earlier, there was an abundance of animal plankton with numerous full-grown individuals.
Mention should here be made of the mutually beneficial relationship derived by the phytoplankton and the zooplankton through an exchange of oxygen and carbon dioxide in solution. We know that during photosynthesis by the plants much oxygen is produced in the waters of the euphotic zone. It is not clear, however, to what extent this means of aeration is a necessary supplement to that which results from diffusion at the contact zone with the atmosphere. In isolated quiet waters it must be an important item. Waters of great depths have a sufficient supply of dissolved oxygen to support their characteristic types of animal life and this must have been transported directly from the surface or euphotic zone through diffusion and the action of water currents. It is probable, however, that a greater rate of metabolism in the more abundant animals of shallower depths, where temperatures are higher and food more abundant, sets up a requirement which could not readily be met by diffusion of oxygen from the surface alone. Plankton animals do occur in the oxygen minimum layer below the euphotic zone of the Pacific, and in the Gulf of California they are found at mid-depth layers where oxygen is near zero. The numbers of animals in the oxygen minimum layer are nevertheless small, and this may have resulted in part from the low oxygen content in the absence of photosynthesis or rapid diffusion from better aerated waters.
Plants in the lighted zone must derive some benefit through the carbon dioxide produced by animals living in the same waters; but here also the significance of this source, though certainly not negligible, has not been established.
The interrelations of the plankton animals have not been studied in such detail as those between the plants and animals. That many of them are carnivorous or omnivorous has been mentioned, and among these the medusae and ctenophores are notably influential in sweeping the waters clean of other planktonic animals upon which they feed (p. 890).
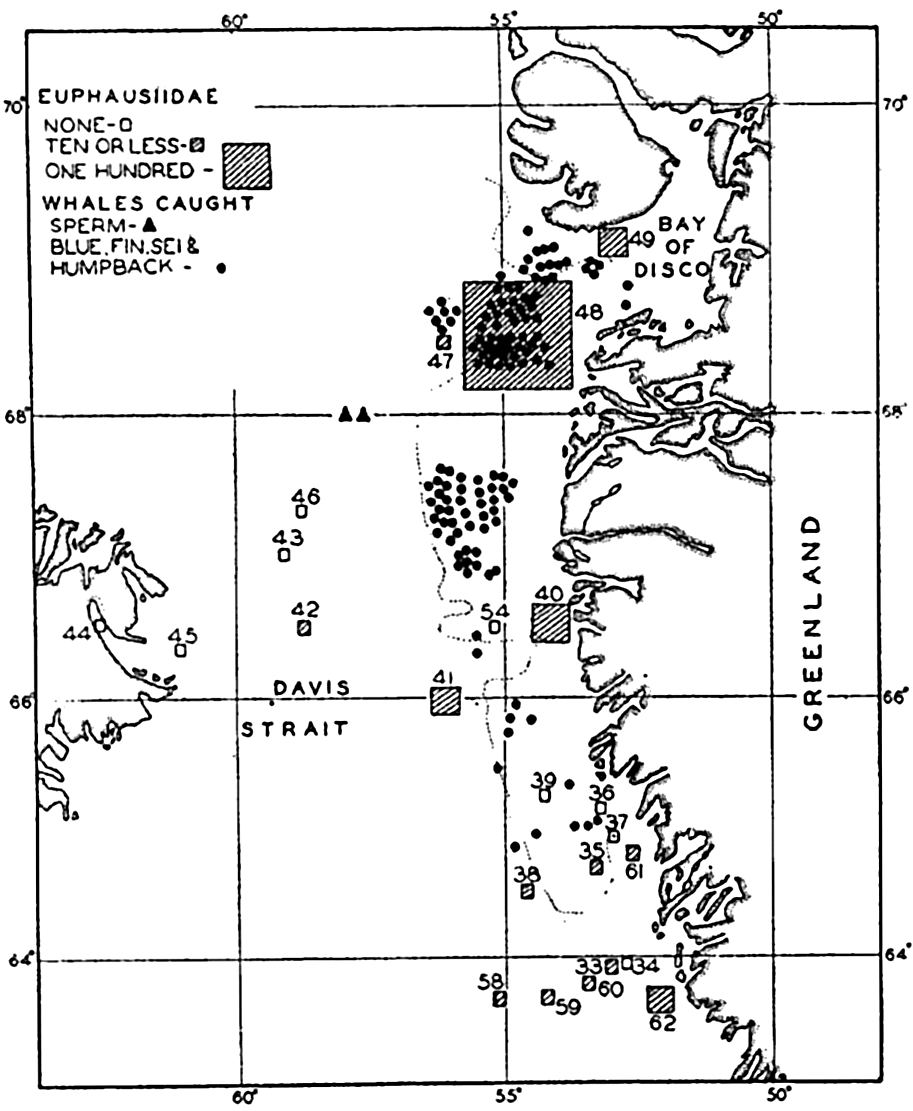
Correlation of the catches of whales with abundance of euphausiids in Davis Strait. (After Hjort and Ruud.)
Nekton
The migrations and concentrations of nektonic animals are governed largely by two biologic factors, reproduction and search of food. Mention need only be made of such truly phenomenal alternating wanderings as are witnessed in the salmon and the eel. Many other fishes also migrate, although the reasons for the migration of some of them have not been ascertained even in a general way. As a matter of fact, even in the
Among the great wanderers of the seas are the marine mammals. The habits of the Alaskan fur seal in returning to the Bering Sea to breed are widely known, and some whales migrate regularly to warm waters for breeding and between breeding times to the feeding grounds of the polar regions for food.
It will be realized from the above that any study of migratory animals and their habits as related to these biologic factors is complicated to the extent to which breeding and feeding grounds do not coincide.
It is well known among whalers and scientists that blue, fin, sei, and humpback whales feed wholly or partly upon planktonic life. Scientific investigations carried on in cooperation with whaling companies have shown, as might be expected, that the occurrence of plankton-feeding whales is correlated with the distribution of the planktonic life upon which they are known to subsist. In Davis Strait it was shown by Hjort and Ruud (1929) that young euphausiid crustaceans (the young are considered to reflect numbers of old specimens not so readily caught with vertically hauled nets) had their maximum concentration over certain coastal banks, and it is precisely in these waters that the maximum numbers of whales also occurred (fig. 244). The euphausiids tend to collect in swarms during breeding and the appearance of blue whales coincides with the periods of spawning of the dominant species in the region. Sperm whales, which feed largely on squids and not directly on plankton, are found to be present farther offshore, as indicated in the figure. The same authors compare the numbers of sei whales (Balaneoptera borealis) with the concentration of Calanus finmarchicus during the same period from the coastal banks. Figure 245 shows a striking correlation in the abundance of these animals, with the increase in Calanus preceding somewhat the appearance of the whales. Data also
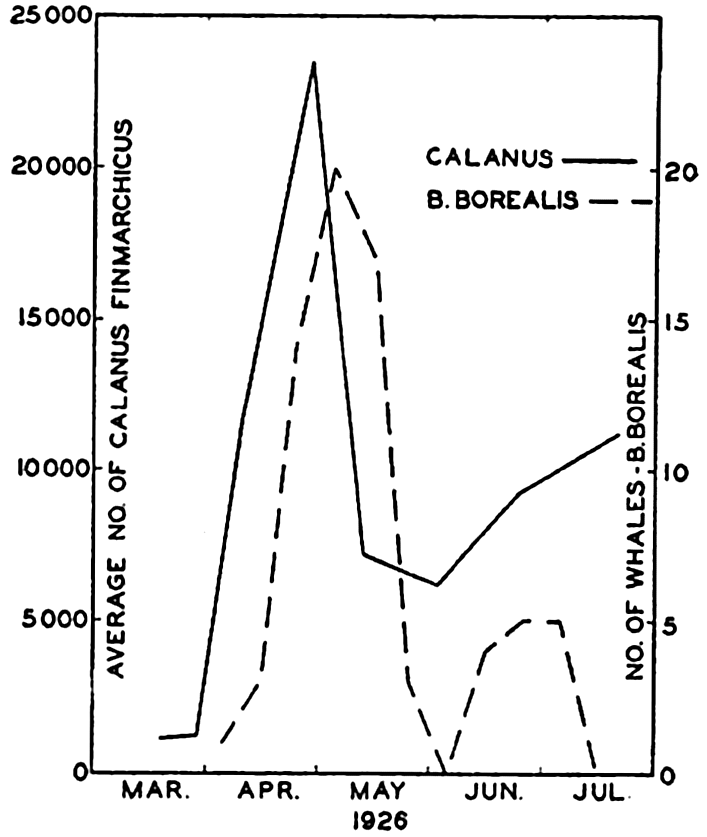
Correlation of the catch of sei whales (Balaneoptera borealis) with the number of copepods. (After Ruud.)
Investigations by the Discovery likewise showed a positive correlation between distribution of blue and fin whales and abundance of zooplankton, especially with their favorite food Euphausia superba (Mackintosh. 1934, Hardy and Gunther, 1935).
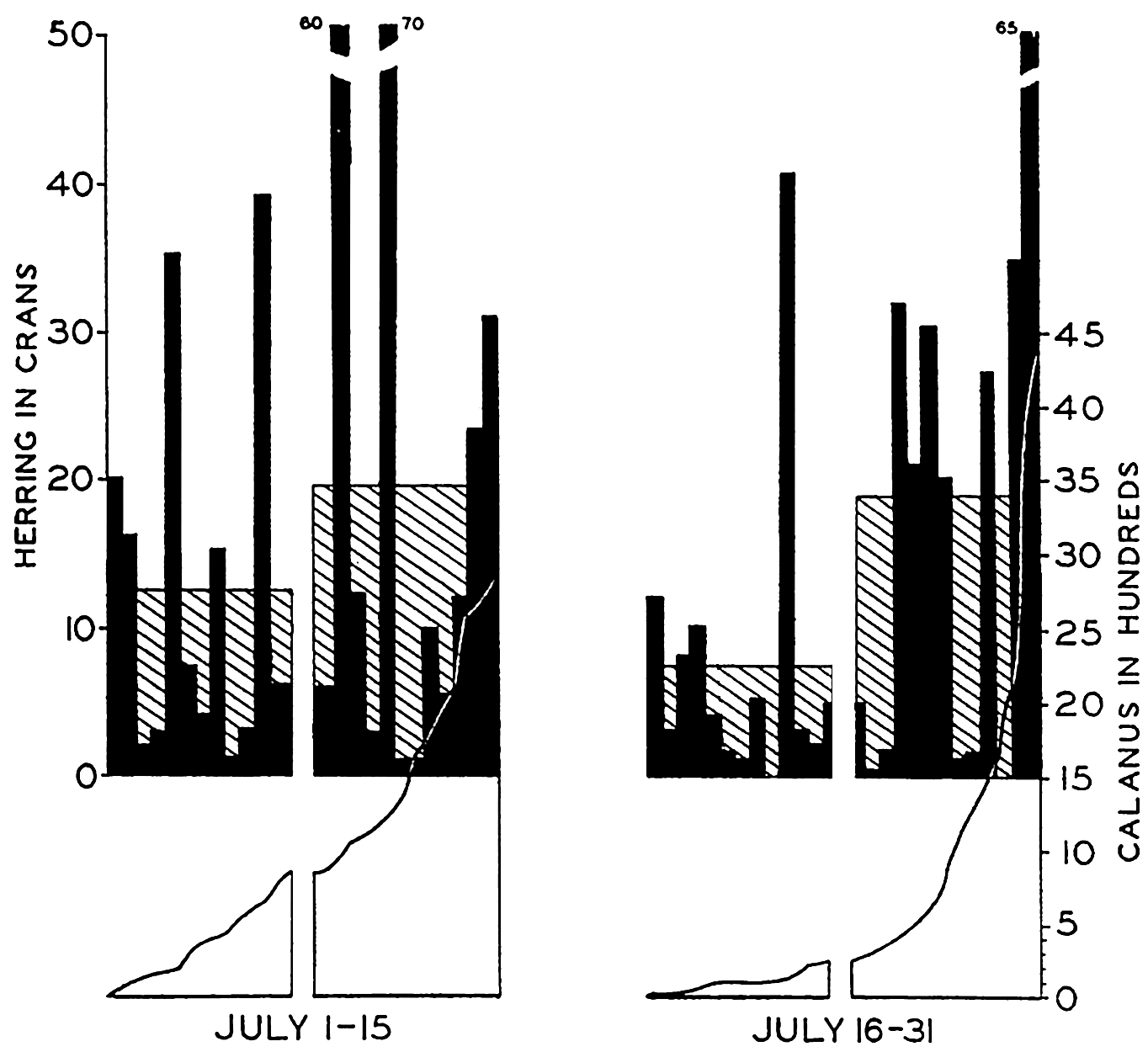
Histograms showing (in black) the individual catches of herring in half-monthly periods arranged in each period from left to right in the order of ascending values of Calanus in the associated plankton samples. The average catches of herring are shown as shaded histograms. The left half of each series represents catches in the poorer, and the right half in the richer Calanus water as indicated in the graph for associated Calanus values. (From Lucas.)
Numerous other examples might be given showing the concentration of whalebone whales in coastal areas rich in plankton food, and their instinctive migrations into these waters at the swarming season of such forms as euphausiids is truly phenomenal. One is impressed with the efficiency of the metabolism of some of the large animals which feed upon the animal plankton. For instance, at birth the blue whales are about 7 m long and weigh about 2000 kg (4400 lb), and at weaning, seven
Among the pelagic fishes the best material illustrative of correlation with plankton comes from studies of the herring. It has long been believed that the movements and concentrations of these fish are associated with the relative concentrations of the zooplankton upon which they feed. Hardy, Lucas, Henderson, and Fraser (1936) have recently investigated the plankton by means of the plankton indicator (a quick-sampling device) and, in correlating the numbers of Calanus copepods of the plankton with the amount of herring caught by fishermen in the same areas, they have shown in most instances that the greatest number of adult herring are caught in Calanus-rich waters. Theoretically the gains derived through fishing exclusively in Calanus-rich waters may be as high as 21 per cent. In Fig. 246, from Lucas, is shown the general trend of the relation that exists when the Calanus series are arranged in ascending order of numbers and each half of the series compared with the average catch of herring for the period.
A negative correlation is found between heavy phytoplankton and herring. North Sea fishermen have observed this in practice, and designate the heavily diatom-populated waters as “weedy water” or “stinking water” and consider it a bad omen to fishing.
Benthos
Among the benthic organisms the animate factors of the environment are manifest not only in predacity and competition for food but also in competition for favorable space for attachment or burrowing. The competition for space is most obvious in sessile forms such as barnacles and mussels of the intertidal zone, where overcrowding must lead to elimination as growth proceeds, and many pelagic larvae of sessile forms perish by failure to find attachment at all. A foremost problem in oyster culture is the curtailment of oyster production that results in beds in which the slipper shell Crepidula is a serious competitor with the oyster for food and space. In English waters as much as twenty tons of Crepidula have been removed from certain beds daily, and some beds there and elsewhere have been completely abandoned for culture because of this pest.
Many good examples of the influence of biological factors on the benthic populations are also offered by studies of other pests on oyster beds (Orton, 1937). Among these we may mention the common drill Urosalpinx cinerea, which may destroy 50 per cent of the young oysters settling on a bed. In Australia whole beds have been destroyed in a few days by yet another predator, the mangrove crab Scylla serrata. The extent to which sea stars are a factor in reducing the yield of oysters in Long Island Sound is indicated by reports that as much as 500,000 bushels are annually killed by these pests in that small area alone (Galtsoff and Loosanoff, 1939).
In the above, attention has been directed to the effect of predacity on the prey, but we may shift the emphasis and consider an instance wherein the predator is the object of economic interest.
According to quantitative investigations of benthic invertebrates with reference to their utilization as food for the bottom-feeding plaice, it has been calculated by Blegvad (1930) that over an area of 3445 square miles in the Kattegat there is produced, as a minimal figure, about 230,000 tons of “first-class” plaice food. (The food is considered first-class when it is of a type available to young as well as adult animals, and second-class when it can be eaten only by the grown animals.) This amount of food is believed sufficient to produce 12,800 tons of plaice. Animals known to compete for the food of the plaice take about one tenth of the total.
These few examples selected from a field of study intensively pursued for economic reasons are doubtless illustrative also of similar biological aspects that enter into the control of any population in the sea (see also fig. 249, p. 937).