Zooplankton, the Floating Animals
The benthic and nektonic groups were the first to be observed or studied by man. But as systematic investigations of the sea progressed, with the improvement of collecting methods and the use of the microscope, it became obvious that another ecological group existed, which for convenience of study should be considered distinct from the bottom-dwelling and the fast-swimming forms to which it holds such vital relationships. This group is now called the plankton, a term first used by Victor Hensen in 1887 to distinguish the vast assemblage of feebly swimming or floating organisms, both plants and animals, that drift about with little or no resistance to water movements. To the plankton belong not only by far the greatest number of marine organisms, but also those of widest dispersal.
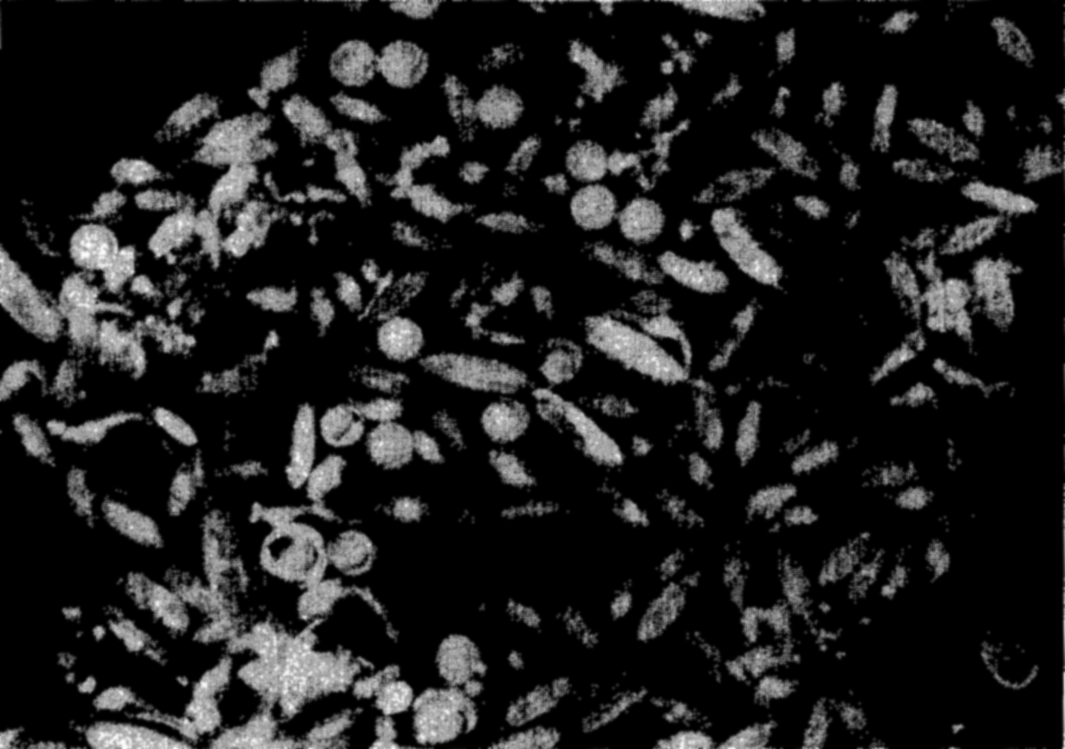
Photomicrograph of a plankton community containing copepods (Calanus and Metridia), young euphausiids, and fish eggs.
[Full Size]
The general plankton is divided into two large groups: Phytoplankton and Zooplankton. To the phytoplankton belong most of the diatoms, dinoflagellates, and other unicellular plants or animal-like plants that are capable of synthesizing food. These, in contrast to the consumers making up the zooplankton, are the chief producers of the primary food of the sea. They are more fully discussed in chapters XVI and XIX.
In the zooplankton are included many of the protozoa, especially tintinnids, radiolarians, and foraminifera, a large number of the small crustaceans such as copepods, ostracods, euphausiids, amphiopods; the jellyfishes and siphonophores; many worms; a number of molluscs, such as the pteropods and heteropods; and the eggs and larval stages of most of the benthic and nektonic animals of all kinds. Figures 222 and 223 are photomicrographs of typical zooplankton catches from coastal waters.
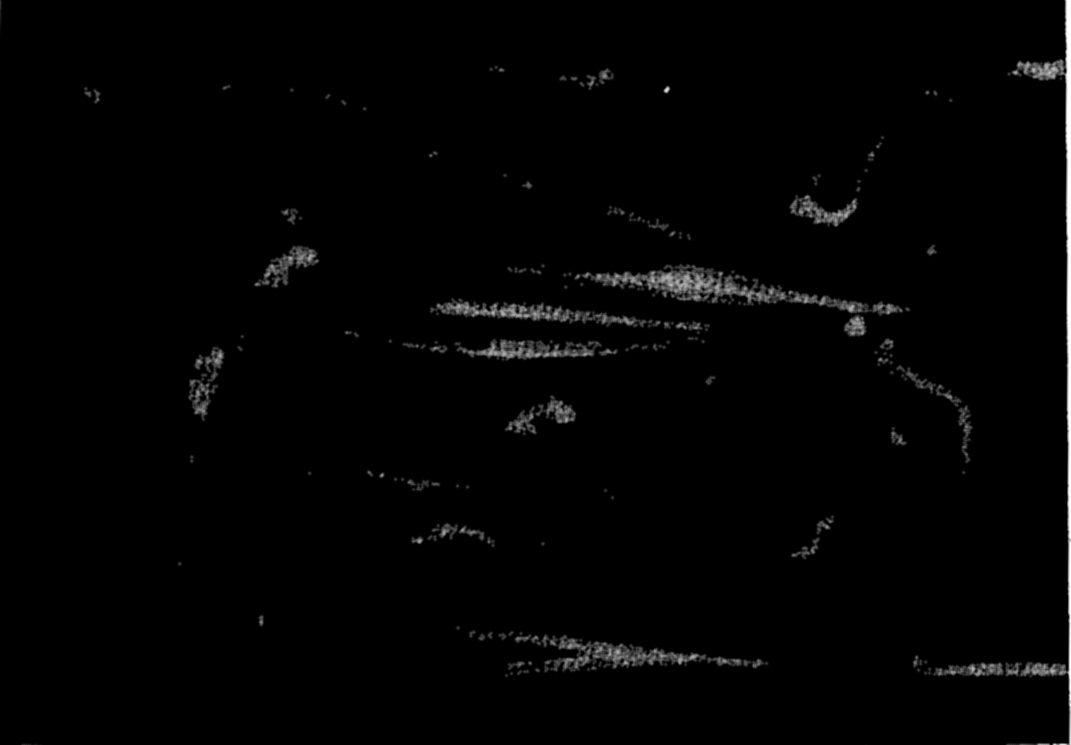
Photomicrograph of plankton dominated by the arrow worm, Sagitta, and including copepods, young euphausiids, and fish larvae.
[Full Size]
Undoubtedly the first notice taken of the general microscopic plankton was not of the individual organisms themselves, but rather of such phenomena as discoloration of the water, associated with their swarming. These observations, of course, could only increase the mysteries of the sea, for the causes were in most instances entirely unknown. Probably one of the earliest references applying to general plankton phenomena was made by Pytheas in the fourth century B.C. During a voyage in the North Atlantic he reported that the sea became sluggish and thick like a jellyfish (Herdman, 1923). There are records where the occurrence of the color phenomenon was accompanied by considerable destruction of marine life along the coasts, such as has been witnessed on the coasts of California, Japan, and elsewhere. The terms “red-water,” “red-tide,” and “sliming” have been applied to some of these displays. In other instances, the discoloring became associated with good or bad fishing conditions, and recent investigations (Hardy et al, 1936) have, indeed, shown that there is a correlation between herring catches and color of water resulting from microorganisms (p. 907). Perhaps the most
Among the first important typically planktonic organisms to be studied in detail were the boreal copepod Calanus finmarchicus (fig. 227-c), described by Gunnerus under the name Monaculus finmarchicus in 1765, and Ceratium tripos (fig. 74-a), a dinoflagellate described by O. F. Müller in 1777. Little was accomplished, however, until about 1846, when Johannes Müller introduced the plankton net for use in extensive studies. Since then the plankton has been the subject of a vast amount of investigation.
It will be noted that the three categories, benthos, nekton, and plankton, are not sharply separated. There are not only transitory stages but also many organisms of borderline habits. Most members of the nekton and benthos are for a period properly plankton. The swimming powers of many animals put them midway between the plankton and the nekton, and many forms, for example some mysids, amphipods, cumacids, and so forth, live both on or near the bottom and are sometimes called hypoplankton. Many of the planktonic animals do swim freely and may quickly move many times their body length, although, owing to their small size, little distance is covered. The direction of swimming is frequently haphazard and intermittent, resulting in little progress. However, under certain directing stimuli such as light and gravity, the resultant movements do bring about consistent, although restricted, migrations.
Categories of animal plankton are defined according to duration of the life cycle in the pelagic state, according to size, or according to habitat.
Temporary Plankton. The planktonic eggs and larvae of the benthos and nekton make up what is known collectively as the temporary plankton (fig. 224). This temporary element, or meroplankton as it is sometimes called, is especially abundant in the neritic waters and is composed mainly of developmental stages of the invertebrates, but includes also the young of the fishes.
The following examples give the computed maximum concentrations of various invertebrate larvae taken at separate stations by means of a No. 20 plankton net towed vertically from 25 to 0 m in neritic waters of the Bering Sea during August (Johnson, 1937):
Larvae | Number per cubic meter of water |
Ophiopluteus. | 1,235 |
Echinopluteus | 12,195 |
Bipinnarian | 837 |
Annelid | 8,130 |
Barnacle nauplius | 694 |
Pelecypod veliger | 17,073 |
Gastropod veliger | 7,883 |
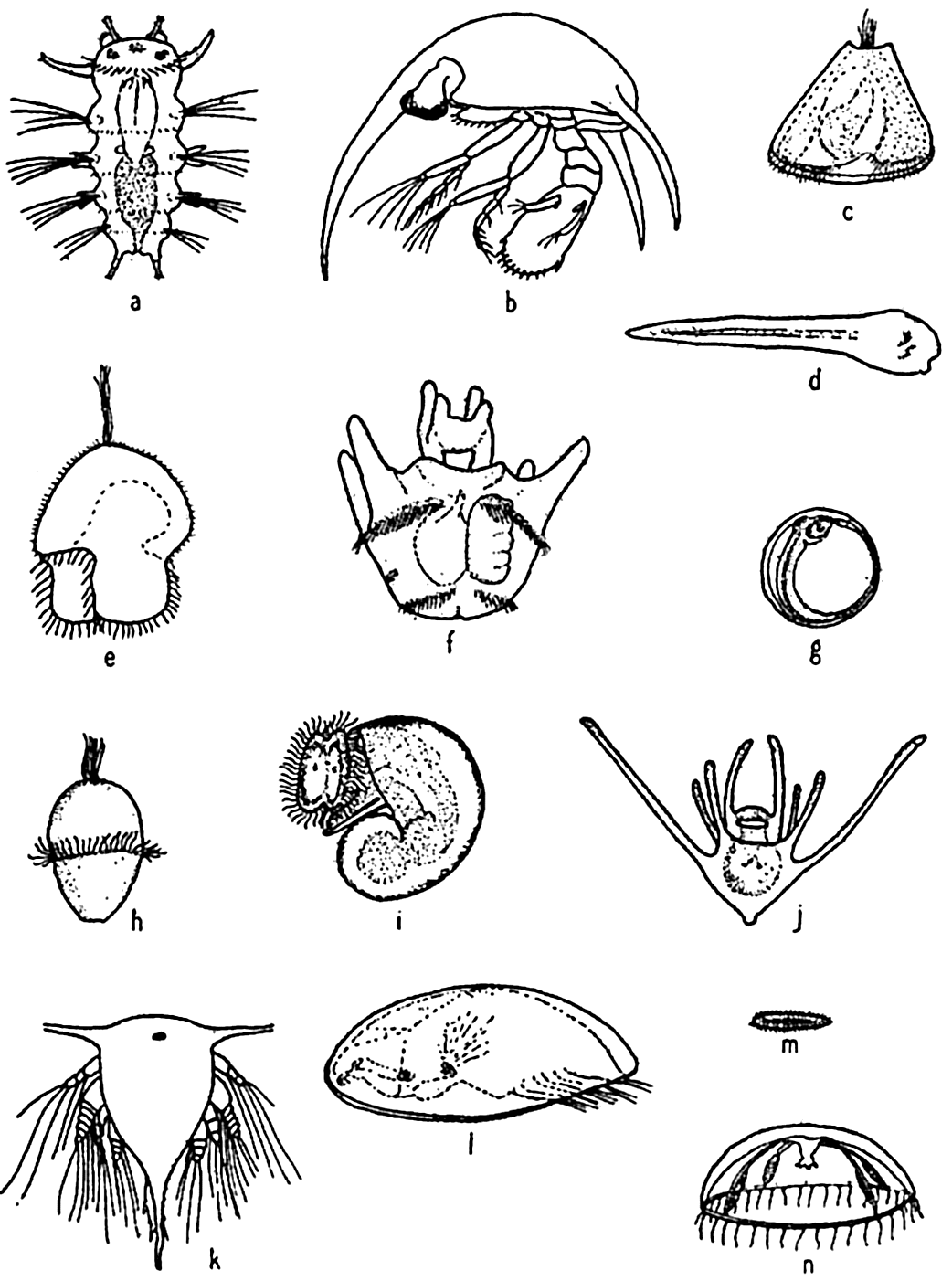
Characteristic larvae of the meroplankton: (a) chaetate larva of the annelid Platynereis agassizi; (b) zoea of sand crab, Emerita analoga; (c) cyphonautes larva of bryozoa; (d) tadpole larva of sessile tunicate; (e) pilidium larva of nemertean worm; (f) advanced pluteus larva of sea urchin; (g) fish egg with embryo; (h) trochophore larva of scaleworm; (i) veliger larva of snail; (j) pluteus larva of brittle star; (k) nauplius larva of barnacle; (l) cypris larva of barnacle; (m) planula larva of coelenterate; (n) medusa of hydroid.
[Full Size]
The temporary plankton is characteristically seasonal in occurrence since it is dependent upon the spawning habits of the parental stock. But there is sufficient variation in spawning time of different species, or even continuous spawning of a single species, to provide a greater or smaller amount of temporary plankton at all seasons, even in high boreal waters where the temporary element is usually much suppressed. The length of larval period is also important in this respect, and may vary
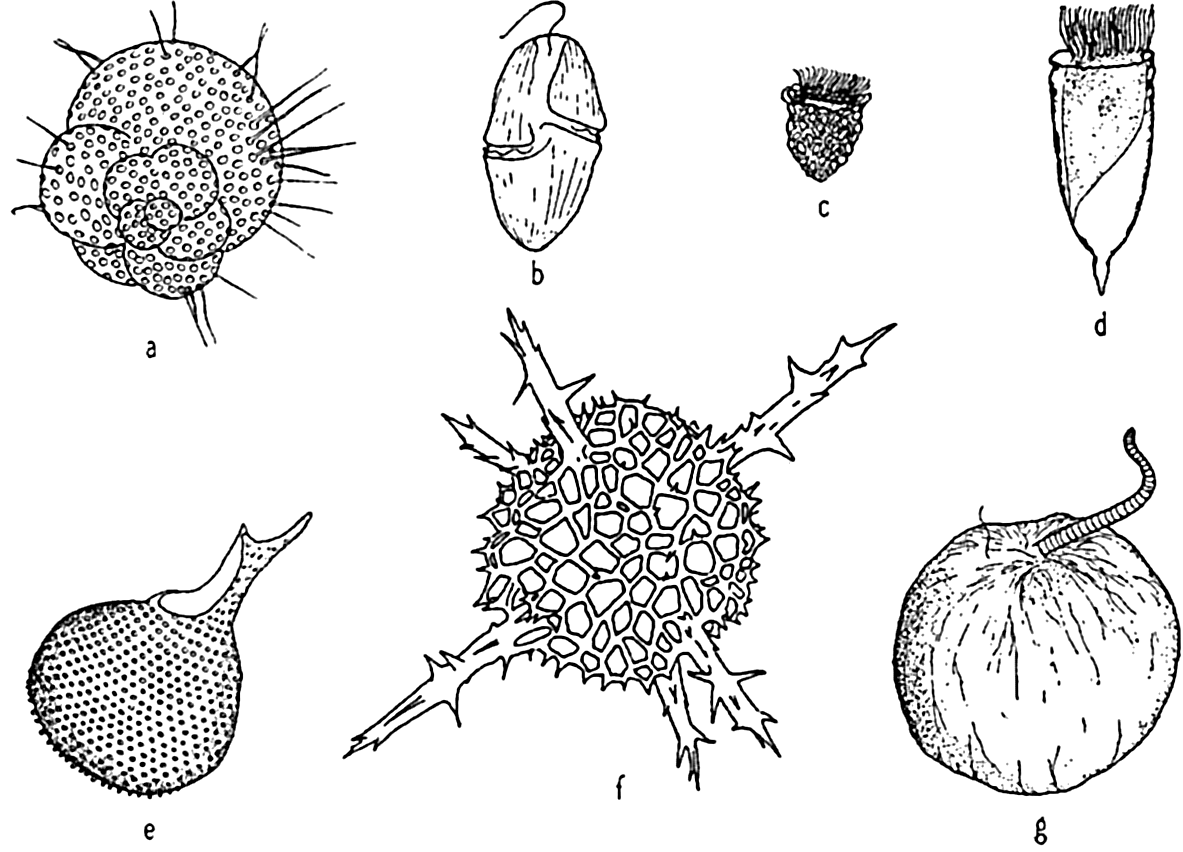
Characteristic holoplankton protozoa: (a) foraminifera (Globigerina); (d) dinoflagellate (Gymnodinium); (c) tintinnid (Stenosomella); (d) tintinnid (Favella); (e) radiolarian (Protocystis); (f) radiolarian; (g) dinoflagellate (Noctiluca).
[Full Size]
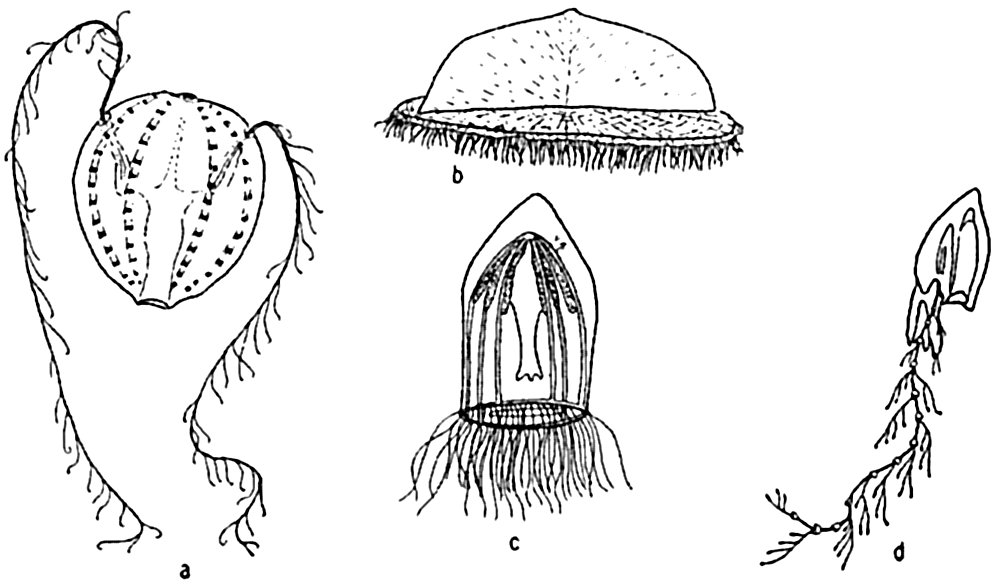
Characteristic holoplankton coelenterates and ctenophores: (a) comb-jelly (Pleurobrachia); (b) siphonophore (Velella); (c) jellyfish (Aglantha); (d) siphonophore (Diphyes).
[Full Size]
Permanent Plankton. The remaining part of the plankton is made up of animals living their complete life cycle in the floating state and is called the permanent plankton or holoplankton (figs. 225–228).
The holoplankton is composed of forms representing nearly every phylum of the animal kingdom with the exception of the sponges, bryozoans,
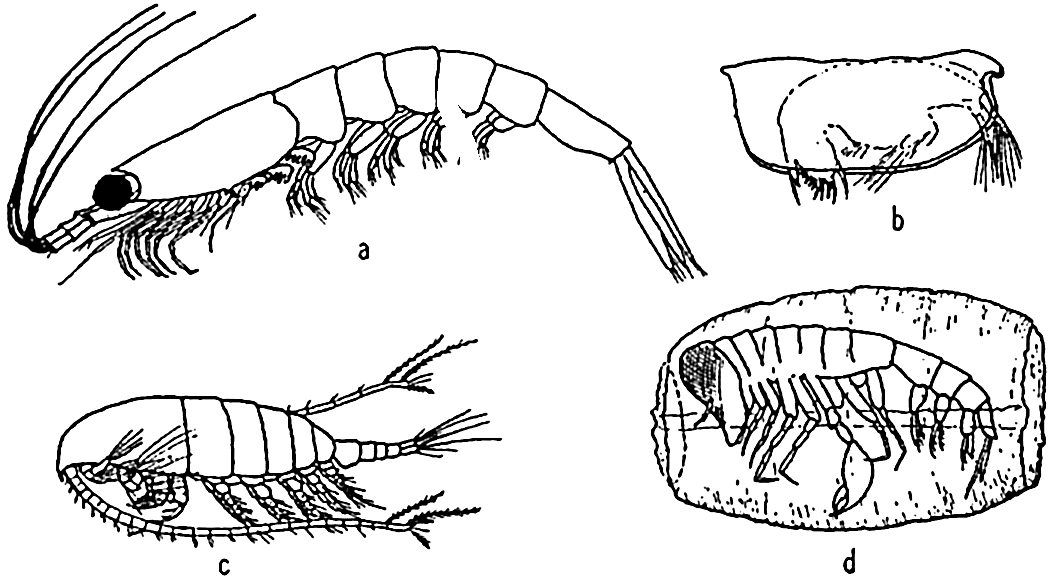
Characteristic holoplankton crustaceans: (a) euphausiid (Euphausia); (b) ostracod (Conchoecia); copepod (Calanus); (d) amphipod (Phronemia) in empty mantle of the pelagic tunicate Salpa.
[Full Size]
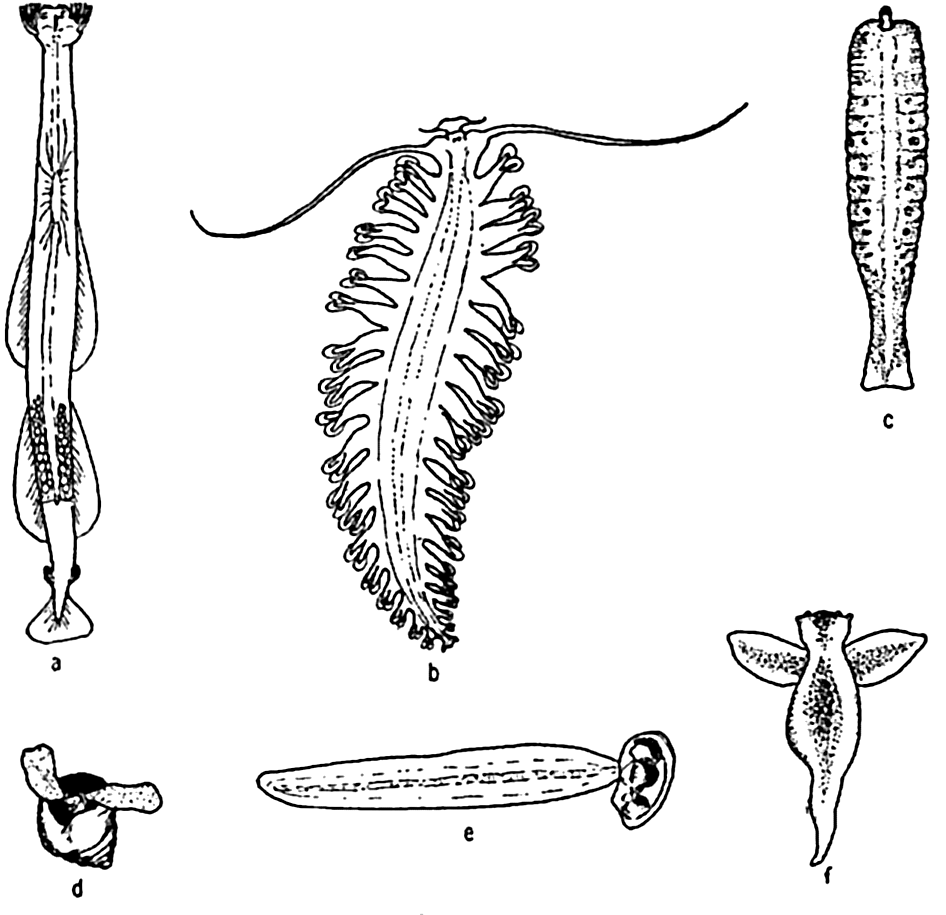
Characteristic holoplankton, miscellaneous: (a) arrow worm (Sagitta); (b) annelid (Tomopteris); (c) nemertean (Nectonemertes); (d) pteropod mollusc (Limacina); (e) tunicate (Oikopleura); (f) pteropod mollusc (Clione).
[Full Size]
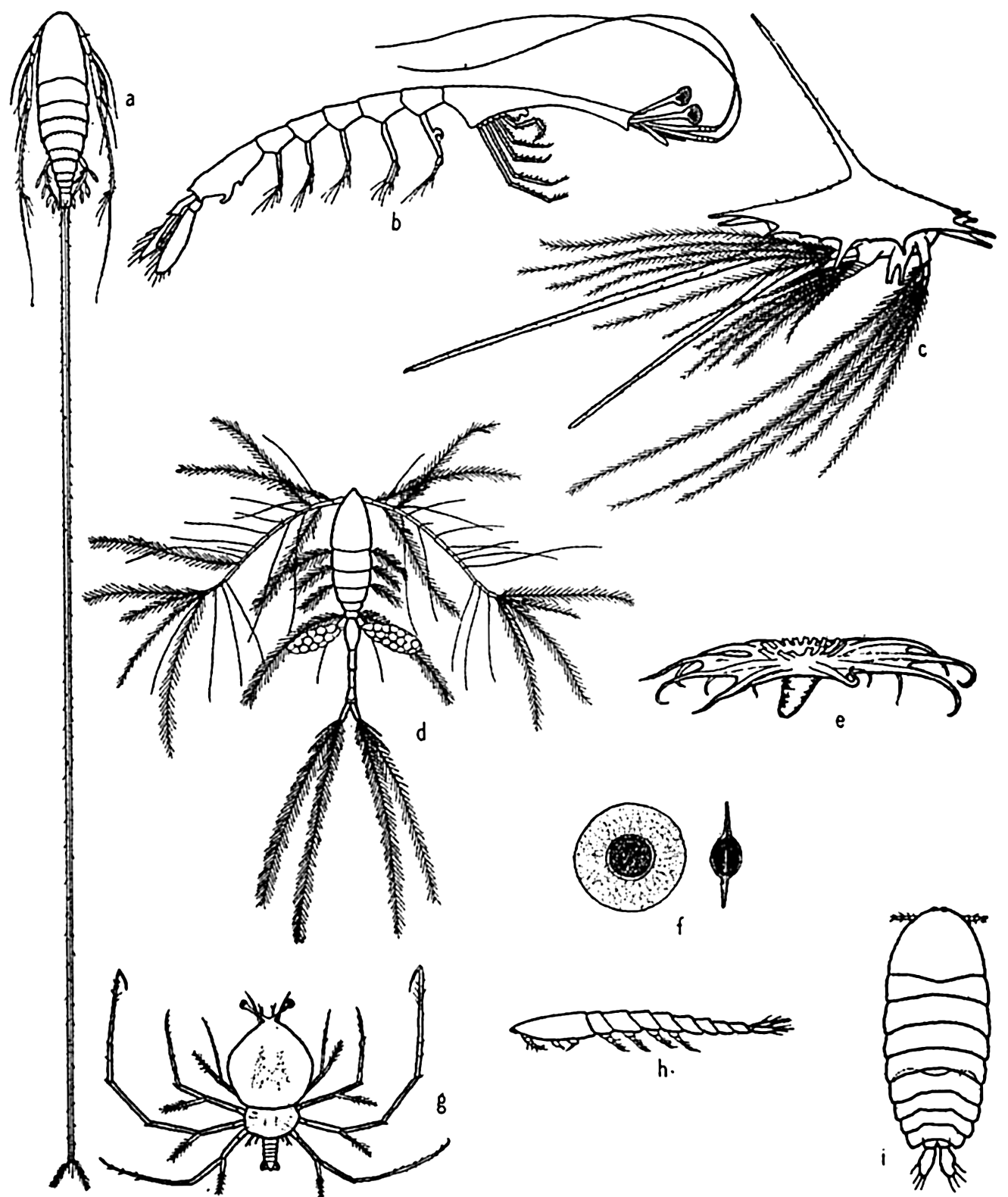
Some floating adaptations of plankton animals: (a) copepod (Aegisthus); (b) decapod (Lucifer); (c) barnacle nauplius; (d) copepod (Oithona); (e) holothurian (Pelagiothuria); (f) pelagic egg of copepod (Tortanus); (g) phyllosome larva of lobster; (h, i) copepod (Sapphirina), side and dorsal views.
[Full Size]
All other phyla are abundantly represented in the holoplankton. However, no plankton animals play so vital a role in the economy of the sea as do the Crustacea of the phylum Arthropoda, and among these the copepods rank first in most parts of the ocean although in many instances euphausiids are of equal or greater importance as food for the larger plankton-feeding animals (p. 905). Among the higher crustaceans we should mention the decapod crustacean, Lucifer, which, though of small importance numerically, illustrates a remarkable divergence from others of the group in adjusting itself to a floating life (fig. 229-b). It is of interest to note here that in the sea, as on land, it is the arthropods that have gained the greatest diversity and numbers. In the sea the Crustacea are a counterpart of the Insecta on land.
Macro-, Micro-, and Nannoplankton. Most of the planktonic organisms are microscopic or semimicroscopic in size but there are notable exceptions, for instance, among the scyphozoan jelly fishes, some of which may attain 1 m or more in diameter, with tentacles up to 25 m long. This wide range in size has, for convenience of study, led to yet further subdivision of the plankton on the basis of relative size. Thus we may distinguish broadly between three (or more) convenient size groups. No sharp lines can be drawn between these, but usually the macroplankton is that taken with a coarse net. It includes the large forms and many small ones that can be readily seen with the unaided eye, that is, animals of about 1 mm or more in length that would be normally caught with a net of No. 00 or 000 bolting cloth. (The phytoplankton does not usually form a part of this division.) The forms between about 1 mm and 1 cm are sometimes called mesoplankton, but the term should be avoided in this sense because it is also used to designate the general plankton living in mid-depth waters below the epiplankton. The largest of the plankton forms are sometimes called megaloplankton. The microplankton, also in part called net plankton, is that which is composed of individuals below about 1 mm in size, but yet large enough to be retained by a net of No. 20 bolting cloth with a mesh aperture of about 0.076 mm. The nannoplankton (dwarf plankton) comprises many of the very small forms (about 5 to 60 μ) such as the smaller diatoms, dinoflagellates, coccolithophores, protozoans, and bacteria, which readily pass through the meshes of a new No. 20 net (fig. 90, p. 377) and must therefore be collected by centrifuging the water. Sizes below these are the ultraplankton. Lohmann (1903) demonstrated the presence of nannoplankton by using hard filter paper, and also by a unique method in which he examined the tiny forms caught in the filtering apparatus of the “house” of the appendicularian Oikopleura. The appendicularian “house” is a temporary gelatinous structure that serves as a complete investing case which protects the animal and also acts as a filtering apparatus for catching food (fig. 239). In the “house” there is a set of
Neritic and Oceanic Plankton. Many pelagic organisms, both plants and animals, are bound strictly to coastal waters while others occur normally only in offshore waters. These requirements give rise to two divisions of the plankton, based on their relative dependence on the coast.
To the neritic ecological division belong the forms which normally inhabit the waters in the coastal areas and extend only a short distance seaward, depending upon the depth and type of circulation. The neritic forms on the whole prefer relatively warm water during the growing season, with some reduction of salinity. They are usually seasonal in occurrence, especially in the northern latitudes. Vast swarms of pelagic larvae of benthic invertebrates and many fish eggs and fish larvae are characteristic of the neritic plankton. Resting stages occur in the life histories of many of the species, such as the cladocerans Podon and Evadne, and the rotifers. It must not be understood, however, that the neritic plankton is composed chiefly of temporary plankton. On the contrary, the dominant element is often the holoplankton, adults and young, which are bound to the coastal regions for some unknown reason—perhaps by nutritive, physical, or chemical bonds. The copepods loom large among these permanent plankton forms.
In discussing the plankton of the Gulf of Maine, Bigelow (1926) cites certain of the medusae as conspicuous inhabitants of neritic nature. These are the moon jelly Aurelia; the large red jellyfish Cyanea; and the small hydromedusæ Melicertum companula and Sarsia, all of which are budded from sessile stages in the shallow coastal zone. Many other medusae and also pelagic larvae of such animals as shore crabs and molluscs could be added to this list of strictly neritic forms. It is significant to note, as Bigelow has pointed out for Melicertum companula, that some of these pelagic stages are confined mainly to coastal zones because they have originated there from parental stock dependent upon the immediate coast and not because of any inability to withstand oceanic conditions. Some neritic animals may be found in oceanic waters into which they have been carried, but they are relatively or totally sterile, being unable to complete successfully all stages of the life cycle (p. 859).
The neritic forms are the greatest producers of the sea, for in the coastal waters the food materials are most readily available for plants and through these, successively, for small and large animals. Practical evidence of this relation is seen in the fact that most commercial fish are taken in coastal waters.
The oceanic division, as the name implies, includes forms that are contrasted with the coastal population, since they occur typically at some distance from the coast and over profound depths in the open sea. They constitute the so-called “blue-water” or high-oceanic population. The animals of the oceanic plankton are characteristically holoplankton types that are not dependent on the sea bottom or on the proximity of land; indeed, as a rule, they do not survive long if blown by winds or swept by currents into really neritic situations. Characteristic animals of the oceanic plankton are: Velella, which floats in vast swarms at the surface with its oblique sail projecting to catch the surface breeze; the salps; the violet snail, Janthina exigua, which in time of storm drifts to the beaches in company with Velella; many copepods of different genera, Sapphirina, Rhincalanus, Eucalanus, and others. It is not possible to distinguish sharply between the neritic and the oceanic populations, for there is much overlapping at the border line; there are also forms of relatively great ecological valence, that is, forms tolerant of a wide range of ecological conditions, and such forms are to be found indifferently in both coastal or offshore situations. These are the panthalassic types.
Special Adaptations of Animals to Planktonic Existence. In discussing the adaptations of the phytoplankton, it was pointed out that the rate of sinking of a body heavier than water depends upon the ratio of surplus weight to friction, and friction in turn is determined mainly by the surface area. The simplest way to obtain a relatively large area is to reduce the absolute size. Therefore the small size of most planktonic animals is of extreme importance in keeping them afloat; but, as indicated for the plants, special structural adaptations are also provided.
In contrast to most members of the phytoplankton, the planktonic animals usually have some power to swim. That this is highly important, especially for the larger crustacean forms, is shown in the following summary from Gardiner (1933), which gives the average time required for anesthetized Calanus finmarchicus of different lengths to sink through a column of water 250 mm deep having a temperature of 18.5°C and a salinity of 35.01 ‰:
Length of specimen (mm) | Mean time for sinking (seconds) | Length of specimen (mm) | Mean time for sinking (seconds) |
2.1 | 181.3 | 3.2 | 70.3 |
2.3 | 110.6 | 3.4 | 58.9 |
2.6 | 114.9 | 3.6 | 57.9 |
2.8 | 106.8 | 3.9 | 43.9 |
3.0 | 74.3 | 4.0 | 31.0 |
The experimental data included additional lengths, and the correlation between sinking time and length of specimen, expressed as coefficient of correlation, was
The ability to swim varies greatly with different animals, but even very feeble swimming, when favorably directed as a response to gravity or to light, must work to great advantage in keeping the organism within the water stratum having tolerable living conditions. The ciliates depend largely on ciliary action as do also the ctenophores, the trochophores, and other similar larvae. It is significant to note that the pelagic young of bottom-dwelling forms almost invariabley possess a great development of cilia or a greater length of spines and bristles in greater profusion than occurs in the adults. Euphausiids and at least the larger copepods keep their locomotive organs (pleopods, maxillae, second antennae, and so forth) almost incessantly in motion for feeding, respiration, and swimming, and appear to depend rather little on special suspensory organs. The chaetognaths, or arrow worms, all species but one of which are planktonic, depend mainly upon swimming, for which they are rather well adapted by development of fins and longitudinal muscles. But many of the plankton animals that can swim are also especially adapted to resist sinking when they are in a state of rest. Here the means of adaptation are mostly along the lines of increased length of appendages, of spines or bristles, or of dorsoventral flattening of the body (fig. 229, p. 818).
The radiolaria and pelagic foraminifera develop long spines and irregular shapes, a method not unlike that employed by some diatoms, and the size of some species of radiolaria may be much decreased in the warmer surface water. In Challengeria, for example, certain of the smaller species averaging about 0.11 to 0.16 mm live in the less viscous surface waters of 50 to 400 m depth, whereas the larger species averaging from 0.35 to 0.58 mm live in the colder viscous waters of 1500 to 5000 m depth. Between these depths the average size of different species ranges from 0.26 to 0.28 mm. The chief method of flotation for many of the copepods is found in the increased length of appendages and the spines or bristles that grow upon them. The tropical forms show a greater development of plumose structures than do the northern species, and this is just what we should expect because of the reduced viscosity of the warmer water (see p. 857). The warm-water copepod, Sapphirina (fig. 229h and i), has no extensive development of spines of plumose structure, but it is flattened dorsoventrally in a manner that enlarges the ventral surface and gives greater water resistance while sinking. The lobster phyllosoma larva is similarly constructed and adapted to its pelagic period. The long spines, antennae, and so forth, common in
The presence of oil is a common and most effective method of reducing specific weight in pelagic eggs; it is also found in the copepods, euphausiids, radiolarians, Noctiluca, and others. In the copepod Calanus finmarchicus there is a well-defined hydrostatic organ filled with oil; this consists of a very thin sinus lying in the mid-dorsal axis of the body. The oil also apparently functions as a food reserve since it varies greatly in quantity, and its quantity is perhaps indicative of past living conditions.
Among the siphonophores, pneumatic floats serve as a hydrostatic apparatus. Physalia and Velella are good examples of this type of adjustment and, in addition to the floats, possess sails that extend above the water surface to enlist the aid of the wind in giving more rapid transportation.
Finally, it should be noted that the water content of many animals of the plankton community is very high, being in excess of 95 per cent in some jellyfish. The jelly-like transparency of many plankton animals, for instance the salps, the leptocephalus eel larvae, the pelagic annelid Tomopteris, the ctenophores, the heteropods, the chaetognaths, the copepods Eucalanus and Haloptilus, and many others attest to the high water content of their bodies. The maintenance of this high water content is facilitated because the body fluids are isotonic with sea water. The skeletons (when present) of planktonic animals are remarkably thin in contrast to many related benthic forms. Some of the typical means of flotation are illustrated in fig. 229.
For a fuller discussion of the planktonic adaptations, the reader is referred especially to Steuer (1910).