IV. Making and Chasing Echoes:
The Marine Physical Laboratory
Another project of broad spectrum began in San Diego under the auspices of the University of California even earlier than the Marine Life Research program, but it did not become a unit of Scripps Institution until several years later. This was the Marine Physical Laboratory, the peacetime successor to the University of California Division of War Research (see chapter 2).
The possibility of university scientists' pursuing postwar studies of underwater sound was proposed to President Sproul by the Chief of the Bureau of Ships on 31 January 1946, when UCDWR was about to be dismantled. Roger Revelle, who was serving in the Bureau of Ships office at that time, recalled later that the establishment of the Marine Physical Laboratory “required a pledge of long-term support from the Bureau. This was a radical departure from previous Navy practice and it required much prayerful consideration by the chief of the Bureau, Vice Admiral Edward L. Cochrane.”[1]
Already some of UCDWR's staff members had completed their projects; Francis P. Shepard and Eugene LaFond, for instance, and some of their workers had
It opened on 1 July 1946 with a scientific staff of five people. Under Task 10 of Contract NObs-2074, on 25 November 1946, the Navy Bureau of Ships assigned as research problems of the Marine Physical Laboratory:
Theoretical and experimental investigations of the physical principles governing the generation and propagation of sound in the sea;
Studies of related phenomena as necessary to provide a broad scientific foundation for the above principles;
Investigations of the principles governing the recognition of signals, with special emphasis on underwater sound signals of all kinds.
Carl Eckart, who liked a university environment and had encouraged the establishment of this university laboratory, became the first director of MPL. In 1942 he had joined UCDWR from the physics department of the University of Chicago, where he had made noteworthy contributions in quantum mechanics, particle physics, and thermodynamics. At UCDWR Eckart turned to the complexities of sonar system concepts and also became associate director there in charge of planning and coordinating research.
The new laboratory was set up on Point Loma, alongside
The first research projects at MPL set the pattern for the nature of the laboratory's studies. Senior Research Associate Russell W. Raitt pursued studies on the reflection of supersonic waves from the sea bottom, assisted by Research Fellow William C. Kellogg, Jr. Research Associate Robert W. Young began work on image interference in the presence of refraction, a project that he soon transferred to NEL. Research Associate Leonard N. Liebermann joined the MPL staff in its first year from Woods Hole Oceanographic Institution, at Eckart's invitation, to study the effect of small-scale inhomogeneities in the sea — very small temperature changes, salinity gradients, and even gas bubbles — on the propagation of sound.
Director Eckart, whose first project was described as a study of the absorption of sound by liquids, gave considerable impetus to all the laboratory's projects. He was especially interested in determining means of finding sound signals buried in other noise, the field that came to be known as signal processing. He also investigated the problem of how sound is scattered from a rough surface. Eckart was the spiritual leader of MPL from its founding until his death in 1973. He tackled each problem with keen insight — and occasional whimsy, such as defining Kelvin's First Law of Oceanographic Instrumentation as: “There is ample driving power in the sea.”
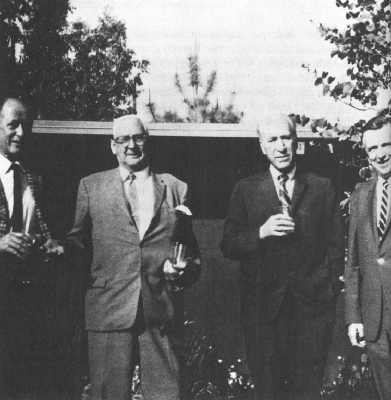
The four directors of the Marine Physical Laboratory in April 1968. From left: Fred N. Spiess, Sir Charles S. Wright, Carl Eckart, and Alfred B. Focke. Photo by Elizabeth Shor.
A mainstay of the laboratory from its beginning was Finn W. Outler, who began as the marine supervisor in 1946 and continued as the technical superintendent and business
When Sverdrup left Scripps in the spring of 1948, Carl Eckart became director of the oceanographic institution, and simultaneously MPL became part of Scripps. Eckart considered his Scripps post temporary, and yielded it readily to Revelle as acting director in March 1950.[*]
[*] Some say he forced the decision by notifying President Sproul that he would no longer be at the director's desk after a certain date. See also chapter 16.
He continued as director of MPL until his sabbatical leave in 1952. Eckart and Revelle invited Sir Charles S. Wright to serve as Eckart's replacement; they had known this physicist, who was born in Toronto in 1887, in his longtime position as Director of Scientific Research for the British Admiralty and, after the war, as scientific advisor on the British Naval Staff in Washington, D.C. Wright's earliest scientific work had been with the ill-fated Scott Antarctic expedition from 1910 to 1913. During World War I he was occupied in “wireless (Radio now) and later ‘Wireless Intelligence.’ ” With the British Admiralty, Wright said that “most of my time involved Geophysics, detectible properties of ships & submarines & their countermeasures. Mines,At MPL in 1955 his successor was Alfred B. Focke, who had joined the laboratory's staff in January 1954 from NEL, where he had worked on studies of very low frequency airborne sound and on the effects of underwater explosions. Focke directed the nuclear depth charge project, Wigwam, soon after assuming the MPL directorship. In 1958 he became technical director of the Pt. Mugu Naval Air Missile Test Center, and from there became a professor at Harvey Mudd College. Fred Noel Spiess, who had joined MPL in the fall of 1952, became director of the laboratory in 1958. A change in research emphasis in the Bureau of Ships led to shifting the major support for MPL to the Office of Naval Research at about that time, so among Spiess's first tasks was separating the facilities of the laboratory from those of NEL, then still funded by the Bureau of Ships. Spiess, like Eckart, served double duty to institution and laboratory, for he continued as director of MPL while he was acting director of Scripps from 1961 to 1963 and director of Scripps from October 1964 to June 1965.
From the handful with which it began, the Marine Physical Laboratory has expanded to about 150 people, has of course outgrown its even enlarged facilities at Point Loma, and now also has offices on the La Jolla campus, where most of the senior staff teach and continue to direct graduate students. The program of this laboratory, which was generated by military need for basic science studies of a complex liquid medium that covers three-quarters of the globe, was defined in 1970 by MPL's senior staff:
… For over twenty years it [the Marine Physical Laboratory] has supported the work of a group of physicists challenged by a desire to understand the oceans and the earth's crust beneath them and interested in the ways in which man can best work on and within the sea. … The program of the laboratory is generated within the staff, giving due consideration to the relevance of the work to basic marine science and to the national interest. In particular this includes concern for the deep ocean problems of the Navy and the basic understanding of the environment needed for it to operate intelligently.[5]
The key to MPL's work is “naval relevance,” a term defined by Robert W. Morse, then Assistant Secretary of the Navy for Research and Development, when he spoke at the dedication of the Nimitz Marine Facilities in March 1966:
In oceanography especially it will often be the scientist who first senses naval relevance in his research. We must make it clear that the term ‘naval relevance’ is not synonymous with military applied oceanography and its possible security restrictions. The scientist must understand that the Navy's problems
― 86 ―with the environment are as large as the oceans themselves and as long range as science itself.[6]
MPL has concentrated on underwater acoustics:
The study of the manner in which sound travels through the sea and the nature of the noises which occur within it. Such studies bring one quickly into contact with the physical, chemical, and biological nature of the sea itself, as well as to a broader look into two related fields — marine geophysics, in which sound signals provide a major tool, and signal processing, in which the goal is to understand the principles which provide the basis for design of sonar systems.[7]
In its early years a major proportion of MPL's work was classified research. The complications and delays in obtaining security clearances for new employees, and the impact of Communist-hunting Senator Joseph McCarthy on these delays, were frequent topics of conversation throughout the 1950s. The situation gradually eased, and MPL staff members contributed to the easing by urging the declassification of many reports and by encouraging publication of research results in nonclassified locations whenever possible. The proportion of classified work is now relatively small. Rapport with the U.S. Navy continues to be very close.
In its pursuit of sound in the ocean — which Revelle once called a “badly designed auditorium” — MPL has produced a good deal of sound itself, and sometimes fury, usually signifying something. “The sound pulses generated by marine physicists,” George Shor said, “have undoubtedly provided more information about the contents and contours of the sea and sea floor than any other single research tool.” The physicists have recorded and analyzed the background
SIGNAL PROCESSING
MPL has distinguished itself in a field that had not been christened when the laboratory began: signal processing, “the theory and practice of applying spatial and/or temporal transformations to samples of an acoustic wavefield to enhance the measurement of a desired signal in the presence of an interfering background.”[8] In other words, how to select the noise that one wants to hear among many others. Signal processing has led to the development of highly sophisticated sonar equipment.
In the 1940s the field was waiting for new technology, something to substitute for bulky and expensive vacuum tubes. High-speed digital circuits appeared in the mid-1950s, and signal processing was fairly launched. MPL was among the pioneers, thanks to Eckart's leadership.
A major contributor was Victor C. Anderson, a lanky physicist who arrived at MPL as a UCLA student in 1947 to investigate the deep scattering layer under Raitt's direction. Anderson proved to be fantastically adept in electronics as well as highly capable of assimilating theoretical approaches. He combined forces with quiet, unobtrusive
Rudnick expanded the concept of “hard clipping,” which was a continuation of the principle of representing the desired signal merely as present or absent, in a continuing series. From this, Anderson in the 1950s, while working on a postdoctoral with Frederick V. Hunt at Harvard, developed a digital technique of rapidly repeating the stored information. Anderson called his system the DELTIC correlator, for Delay Line Time Compressor. The first one was developed for a project in architectural acoustics, but studies of underwater sound could use the technique, and the Navy was promptly interested.
“Those were truly the primitive days of digital technology compared to today,” said Anderson in 1972. “Quantity prices for vacuum tube shift registers were in the 10 to 20 dollars per bit category. Compare that with today's prices for LSI [large scale integrated circuit] random access memories, which are capable of operating at 10 times the speed of those early vacuum tube shift registers and which are now selling in quantity for one cent a bit. We've seen a reduction of a factor of 1000 to 1 in cost alone, let alone the factor of 10 in improved operating speed within the last 15 years.”[9]
The Navy's interest was in ambient noise in the water, and they encouraged other developments in the study of underwater noises. DIMUS, the Digital Multibeam Steering unit, for example, was assembled at MPL. “This was an extension of the two-channel DELTIC correlator concept into a multiple-channel clipped waveform processor,” said Anderson. “Signals from an array of hydrophones are converted into sequences, or one-bit samples, which are delayed in digital memory circuits and then combined in multiple ‘beams,’ each ‘beam’ representing a reinforcement of signals arriving from a specific direction.” Of a series of
“By 1960,” said Anderson, “we were dealing with multiple channel processors using solid-state electronics as discrete components. By mid-1960s, the integrated circuits were filling the gap, and now, of course, we have the MSI [medium scale integrated circuit] and LSI [large scale integrated circuit] technology which has opened new horizons in the implementation of the more sophisticated signal processing techniques.”[10]
It is a far cry indeed from prewar oceanography, when, as Revelle said: “We even had a slogan that the best oceanographic instruments should contain less than one vacuum tube per unit.”[11]
Anderson noted: “Reverberation in the sea presents a major obstacle to the performance of ASW [anti-submarine warfare] sonar systems on the one hand, while it stands as an open portal to oceanographic research on the other. Regardless of which viewpoint is chosen, the incentive for intensive study of this complex phenomenon remains strong.”[12] He has carried out measurements of the distribution of volume-scattering coefficients as a function of depth and frequency in the hope of classifying the larger contributors, such as schools of fishes and dense masses of plankton. He concluded that bubble resonance appeared to be a “dominant scattering mechanism in the deep scattering layer.”[13]
MARINE GEOPHYSICS
The marine geophysical work at MPL was begun by Russell W. Raitt in 1946 with a project to determine how sound waves are reflected from within the sea floor. He first used oscillograms of bottom echoes gathered by Jeffery Frautschy while at UCDWR. Raitt quickly determined that the bottom reflects sound waves diffusely — indeed complexly. By 1947 Maurice Ewing (then at Columbia University, before Lamont Geological Observatory was established there) had shown that low frequency sound waves would penetrate the bottom and that reflections from layers beneath the sea floor could be obtained, thus providing information on the structure.
In 1948 Raitt began using TNT charges to study sub-bottom layers. At that time, and for a number of years afterward, excess explosives were readily available through the Navy, without cost, for research purposes. Raitt began with five-pound charges, but he soon enlarged his scope to fifty-pound TNT bombs. His first attempt at refraction measurements was with Sofar bombs set to sink and detonate when the ship had moved about ten miles away, but the charges proved to be detonating too deep to produce a signal in the low frequencies needed. Raitt then devised a successful refraction method by using a whaleboat as the shooting ship, by using slow-burning time fuses to set off the charges at shallow depth, and by floating the hydrophones at nearly neutral buoyancy one hundred to two hundred feet beneath the surface, streamed well behind the noisy ship. Those who wallowed in the whaleboat, however, did not enjoy it, and Raitt admitted that “experience of several cruises demonstrated that this technique was practicable only when restricted to areas near islands or other land points where lee from wind and waves provided sufficient protection for whaleboat operation.”[14]
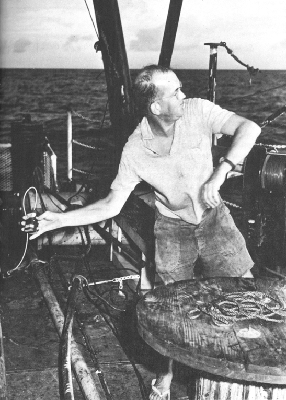
Russell W. Raitt throwing a one-half-pound TNT charge from the Spencer F. Baird, Capricorn Expedition, 1952.
So the Paolina-T or the Saluda (a racing yacht of NEL, also used for research) or the E. W. Scripps was called into service as the shooting ship to accompany NEL's PCE(R)-857, and Raitt began accumulating refraction profiles throughout the southern California borderland. He then joined Midpac Expedition in 1950 for deep-sea refraction work — on which he recorded 1,200 miles of seismic profiles — and for seismic surveys of atolls. Two years later he also sailed on Capricorn Expedition, where he enthusiastically called for larger and larger shots until he had used up 41,409 pounds of TNT, including one single outburst of 480 pounds. As the empty powder boxes accumulated, some of the adept members of the group occupied their spare time with fashioning furniture from them.
George G. Shor, Jr., joined the MPL staff in the fall of 1953, having become interested in Raitt's reports on his deep-sea results, and because the chance of getting to exotic spots like Adak and Rapa seemed much more exciting than analyzing seismic data for an oil company probably based in Houston. He hasn't changed his mind in twenty-odd years. Raitt and Shor, wanderers both, have separately and together crisscrossed the Pacific Ocean from the Aleutians to New Zealand and the Indian Ocean from Mombasa to Djakarta. They are usually planning the next expedition before they have worked up the results of the previous one(s) — and have often departed without even a remorseful glance at the unfinished data. In this they are not alone among oceanographers.
These geophysicists have bombarded the sea floor with sound waves, in the interest of delineating the features within the crust and to the mantle of the earth. Whenever possible they are seeking a signal returning from the Mohorovičić discontinuity, the boundary between crust and mantle. The most satisfactory method for producing the necessary high-energy, low-frequency waves continues
For a while the Arcer was favored; its energy source was electricity. One version — with 60 capacitors and 60 rectifiers producing 10,000 volts — was put together with “the perfection of a fine Swiss watch” by Harold Sammuli and Baron Thomas in 1968 for use on Styx Expedition. Sammuli, who is of Finnish ancestry, believed in reincarnation and approached the Arcer with less trepidation than most others. The Arcer was expanded to reach 18,000 volts, too hazardous for comfort, and it turned out to be not the best sound source, so it was abandoned for other methods. It was spectacular in use, especially at night, for it lit up a patch of sea behind the ship with a stunning electric-blue flash.
Another method, the airgun, has proved to be a very useful tool. Simple and versatile, it creates a succession of bubble pulses under water by means of an air compressor. The records derived from the continuously operating airgun draw a profile of reflections from the upper layers of the sea-floor sediments.
Sonobuoys offer another means of tracing sub-bottom layers while the ship is under way. The sonobuoy was originally devised as a submarine detector to be used from an airplane. It consists of a listening device (a hydrophone) and a radio transmitter to relay the hydrophone output to the airplane. An array of sonobuoys of various radio frequencies can be dropped from an airplane to monitor noises in the ocean. When flung off a ship, a sonobuoy provides a receiver for sound waves generated at the ship and transmitted through the sea floor as the ship moves away. The Navy has been generous with sonobuoys for such researches.
The various seismic reflection and refraction techniques, used by Scripps and by other institutions, have shown that the structure of all the ocean basins is quite similar.
Raitt's early refraction studies established the average thickness of the sediment column throughout the Pacific Ocean, results that were unexpected in the 1950s and were only understood much later when geologists realized that sediments are constantly destroyed as the sea floor shifts outward from spreading centers and into the trenches.
In 1964, Harry Hess of Princeton noted that some seismic records off California and Hawaii indicated that the velocity of sound within the mantle is faster in an east-west direction than in a north-south one. Anomalously, this does not correspond with the structural trends of the Pacific coast. Raitt and Shor set out to confirm the existence of what is technically called anisotropy (which Raitt pronounces “ani so' tropy” while Shor says “ani' sotropy”). The task requires an elaborate pattern of shooting and receiving, such that at least one-half the arc of a circle 30 miles in radius is included in the recording. (Twenty years ago, in the open ocean, a ship wasn't always sure of its position within 30 miles! An expanding Loran network and satellite navigation have made possible much more precise navigation now.)
The most intensive effort at proving anisotropy was in 1966, on Show Expedition, which had five ships, and personnel, equipment, and expertise from four institutions (Scripps, University of Hawaii, Oregon State University, and University of Wisconsin — hence the expedition's
There is a Jekyll-and-Hyde effect in shipboard communication, which is probably caused by Neptune's resentment at the invasion of his realm. Somehow it seems that any perfectly reasonable oceanographer — a fine fellow on land to swap sea stories with, and who sounds most rational there when discussing intricate details of equipment — becomes an impossible tyrant and tyro across a few miles of ocean when he is condensed into a voice or message over the radio. All of one's own shipboard complications are so straightforward to explain; all of the other guy's are beyond comprehension, and could be so easily resolved.
Such a Neptune effect complicated the multiple Show Expedition, as it sometimes has others. Ships became lost; buoys drifted contrary to supposedly known currents; confusion reigned. But the records did confirm anisotropy.
The complications and the costs of multi-ship expeditions have led to designing means of recording anisotropy from a single ship, using moored sonobuoys and balloon-suspended radio transmitters. The rigmarole of simultaneously setting out moorings down to the sea floor as deep as three miles, releasing upward a 20-foot balloon, and casting outward a hydrophone array is very much like a three-ring circus, although rather more colorful in language.
Gerald B. Morris coped with the complications of the balloon-and-buoy system on Scan Expedition in 1969; Morris became chief scientist abruptly, after Russell W. Raitt had to be airlifted to Tahiti when he broke his leg while boarding a longboat at Pitcairn Island. Morris found numerous problems: “The balloons occasionally fell victim to intense squalls, sharks bit through the mooring line setting the buoy adrift, and fish actually ate large parts of the styrofoam floats.”[16]
Raitt's enthusiasm for seismic work at sea has never flagged. From Tasaday Expedition in 1973 he wrote to Shor:
Tasaday leg 6 was extraordinarily successful refraction-wise, having completed 44 refraction stations. … In the western part of the Bay of Bengal, many sonobuoys were rendered useless by a tremendous low-frequency noise, evidently caused by a large current shear between the surface and hydrophone depth, which produced strumming noise on the hydrophones. We had considerable success with primitive hydrophone balancing in which the ship's galley-force were very cooperative by providing us with a fantastic variety of bottles for hydrophone and cable floats. The most successful combination seemed to be a ketchup bottle supporting the hydrophone and Tabasco sauce bottles supporting the leader. Unfortunately, consumption of Tabasco sauce is very small on the T. Washington in spite of my efforts to popularize it and we were forced to resort to unsatisfactory substitutes, such as mustard, pickle and garlic-salt bottles.
Other exciting aspects of the cruise were being chased successively by a Burmese gun-boat, which was seen to man its guns but didn't fire on us, and then by a severe tropical storm, which may have been called a typhoon in other parts of the world. …
HEAT FLOW
The first successful instrument for measuring the flow of heat through the ocean floor was laboriously devised at
One of the disadvantages of the original Bullard probe was the necessity of taking a separate sediment core for thermal conductivity measurements immediately after the probe lowering, which not only required considerable extra time but also could occur some distance from the probe measurement because of ship drift. Scripps and its frequent competitor in creativity, Lamont Geological Observatory, naturally devised slightly different techniques for solving this problem. The Scripps approach was to attach a conductivity needle to a slider on the probe, which measured the conductivity at an average depth of 50 cm below the surface of the sediment. Lamont developed a heat probe attached to the piston corer.
The streamlined instrument devised by Charles Corry, Carl Dubois, and Victor Vacquier in 1968 has proved to be a convenient device at sea, even in weather scarcely comfortable for its handlers: “with the ship rolling up to 35° and in wind speeds up to 60 knots.”[17] The heat probe appears to be more susceptible to breakage or bending on deck than on the sea floor.
The results from the first measurements of heat flow through the sea floor were among several surprises gathered on the first major Scripps expedition, Midpac, in 1950: the temperature gradient was very similar to that measured on land, whereas it had been expected to be considerably less. “The only adequate source of heat that has been suggested is radioactivity within the earth,” noted Bullard,[18]
Other surprises were in store, as scattered measurements of heat flow were taken on various expeditions in Atlantic and Pacific. From Capricorn Expedition in 1952 Maxwell and Revelle reported an unusually high value on the broad topographic feature first known as the Albatross Plateau (part of the East Pacific Rise), which was not recognized until later as having been taken on a ridge. About the same time, Bullard, then at the National Physical Laboratory in England, found a high value in the Atlantic Ocean. As measurements continued, a pattern began to emerge. Heat flow on the crests of the East Pacific Rise and the Mid-Atlantic Ridge, and in the Gulf of California, was generally higher than elsewhere. Geologists were examining these regions closely and by every means at their disposal, as these anomalous regions appeared to be the foci of intensive sea-floor activity. As theories developed around the concept of sea-floor spreading, the measurements of heat flow fitted into the emerging picture: high heat flow indicated areas of more intensive crustal activity.
The development of a convenient shipboard device for measuring the flow of heat resulted in the gathering of more measurements at sea than on land. In 1967 Von Herzen (by then at Woods Hole Oceanographic Institution) and Vacquier estimated that only 11 percent of the 1,300 measurements to that time had been taken ashore. In an ingenious use of the shipboard technique, these researchers carried a temperature probe to Africa and obtained “land” heat-flow measurements aboard a Fisheries Research Unit vessel in Lake Malawi in the east African rift zone. Later Vacquier and John Sclater used a temperature probe in Lake Titicaca in South America. The heat-flow enthusiasts
MAGNETISM
Recording the magnetism harbored in sea floor rocks was a pioneering venture of Scripps and MPL. Jeffery D. Frautschy participated in the early stages of compiling a set of equipment from parts of surplus units, in consultation with the Naval Ordnance Laboratory. In 1952, Ronald G. Mason joined Capricorn Expedition, at the invitation of Revelle, following a meeting of the Institute of Geophysics in La Jolla. On that trip Mason recorded more than 4,000 miles of magnetic profiles, although he noted that such lines “have limited application since they give no indication of the lateral extent or direction of anomalous magnetic trends and therefore provide no basis for quantitative geological interpretation.”[19] Also on Capricorn Expedition, Mason made detailed magnetic surveys of small areas of special interest, such as the Tonga Trench.
Sir Charles Wright particularly encouraged the continuation of a magnetic program at MPL, and Scripps ships began towing magnetometers frequently. In August 1955, Scripps researchers seized an opportunity for a detailed magnetic survey by way of the U.S. Coast and Geodetic Survey ship Pioneer on its hydrographic survey off southern California on a series of east-west lines about five miles apart. Arthur D. Raff and Maxwell Silverman, both alumni of Capricorn Expedition, alternated aboard ship to keep the equipment working. The magnetometer was described by Mason as a
The chart of magnetic intensities from the Pioneer survey startled geologists. Before them lay evidence of great north-south lineations and a single right-lateral offset of 155 kilometers along the Murray fracture zone off southern California. The survey was “the first attempt to make a detailed magnetic map of an extensive area of the oceans,” said Bullard and Mason. “The results are of exceptional interest in that they reveal major structural trends of which there is little or no indication in the topography, and they provide evidence for unsuspected horizontal displacements along some of the faults of the north-east Pacific greater than any that have so far been observed over the continents.”[21]
Mason returned to the Imperial College of Science in London in 1962, but the magnetic program continued at MPL under the guidance of Victor Vacquier. During World War II he had developed the flux-gate magnetometer, the most sensitive method then known for measuring a magnetic field — which, among other uses, could detect submarines. Later, with Sperry Gyroscope Company, Vacquier developed highly accurate gyrocompasses. When he joined the MPL staff in 1957, from the New Mexico Institute of Mining and Technology, he promptly relieved sea-weary Raff on the Pioneer. He then began his own modifications of a borrowed bulky proton magnetometer, which he first improved by separating the amplifying and tuning equipment from the towed “fish” and placing it aboard ship. To get away from magnetic objects such as automobiles while calibrating and testing equipment, Vacquier found a quiet spot under the eucalyptus trees
In 1958 Vacquier made a magnetic survey along the Pioneer Ridge north of the Murray fracture zone and there found the magnetic anomalies offset 265 kilometers — in the wrong direction, i.e., left lateral or opposite to that of the Murray. The next discovery was the vast Mendocino fracture zone where the greatest offset of all was found: 1,185 kilometers, also left lateral. Revelle later called these results “the most important geophysical discovery of the past ten years.”[22]
The structural geologists found that the magnetic lineations were a valuable addition to the data on the history of the sea floor. In 1963, while analyzing magnetic surveys in the Indian Ocean, F. J. Vine and D. H. Matthews at Cambridge University “suggested that [Harry] Hess' idea that a strip of new ocean floor was continually being formed on the axis of the mid-ocean ridge would provide a double tape recording of the intensity and the reversals of the earth's magnetic field. Each magnetic stripe was magnetized when that piece of ocean floor was formed in the central valley on the ridge axis.”[23]
During the 1960s the Scripps magnetic program became part of the routine shipboard measurements and the data were coordinated and tabulated with other geological information by the Geological Data Center. MPL has continued development work on equipment for magnetic measurements and has carried out special programs using magnetometers. Vacquier, for example, conducted detailed surveys of seamounts for measuring the orientation of their magnetization, surveys that showed that the Pacific plate
PLATFORMS AND VEHICLES
MPL's staff includes several people who, as Spiess said, “want to build something and take it out and run it.” Over the years they have produced an astonishing array of devices, under a collection of contrived acronyms — the kind of ocean-going gear that frequently draws puzzled queries from passing ships.
Flip is the most widely known: the Floating Instrument Platform. It is defined as a manned ocean buoy, and its purpose is to gain access to the relatively calm water below the wave-churned surface. This it does by standing on end, or flipping upright, in which position it exposes 55 feet above water and extends 300 feet below the surface. Upright it becomes a stable platform that moves up and down only a small fraction — about five percent — of the height of the passing waves. Designed originally, and successfully, for fine-scale studies of sound sources in the water, it has proved “more valuable to the Navy than when it was first launched.” Those who work from Flip appreciate her stability for their own sake.
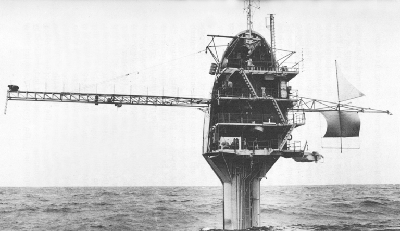
Flip, the floating instrument platform of the Marine Physical Laboratory, in its working position.
Submariners had long known of the advantages of working beneath the wave zone. But submarines are expensive research tools, and do not hold a set depth except under way. Allyn Vine at Woods Hole Oceanographic Institution once suggested standing a submarine on end for research studies. In the late 1950s war-experienced
The first trials (with some trepidations) took place in Dabob Bay in Puget Sound in late July of 1962. There the flipping on end and the return to horizontal were entirely successful, and so they have continued.
When being towed in the horizontal position, the craft is stabilized by concrete and steel ballast well below the horizontal center line. The transition to vertical is accomplished by filling ballast tanks with sea water, which takes about twenty minutes. Personnel on Flip always stay on the outside platform during a flip, for safety's sake, each with one foot braced on the deck, the other on the bulkhead, as the craft shifts. All permanent equipment is on trunnions so that everything can manipulate a 90-degree turn. Mistakes rarely happen, but once an unnoticed can of food became lodged under the galley range during a flip, which prevented that unit from turning, so, of course, the simmering stew was flung all over the neatly made bunks.
Flip has chiefly been used for studies of sound propagation in the water; she was designed, in fact, and used by Fred Fisher for studies of the “twinkle” of a sound source
ORB and RUM are a pair of MPL vehicles that often work as a team. The Remote Underwater Manipulator (RUM) was first intended to work alone, crawling about on the sea floor at depths down to 6,000 meters to gather objects and samples, to take photographs, and to install deep-sea instruments. Victor C. Anderson began assembling it in 1958, starting with a Marine Corps self-propelled rifle carrier; to this he added a boom and a steel claw that could be pivoted in any direction out to about five meters to pick up objects. The gasoline engine was replaced with a pair of heavy electric motors in an oil-filled compartment. Sonar was installed, and a powerful light and four television cameras for sea-floor surveillance from a portable shore station (actually a bus). Power for RUM and sensor signals were provided by way of a coaxial cable 8,000 meters long. Early tests in shallow water were only moderately successful, and RUM was set aside for other projects.
By December 1967, ORB (Ocean Research Buoy) had been developed as a platform for suspending equipment and particularly as a service vehicle for RUM. ORB is a barge 45 feet by 65 feet with a large center well through which the ten-ton RUM is operated by means of a constant-tension winch. It has two laboratories, a galley and messhall, and sleeping quarters for twelve people. “Loading RUM is a somewhat unconventional operation,” its designers wrote. “RUM is first lowered to the bottom of the bay by a crane. Then ORB is moved to a position over RUM, divers attach the strain cable, and RUM is lifted up through the well doors.”[24] Unconventional or not, it does work. RUM has been used for taking cores at depths down to 1,900 meters, for measurements of sediment properties in place, for underwater photography, for recovering equipment at depths down to 1,260 meters, and for sampling deep-sea biological communities. It has the advantage of being able to stay on the sea floor at work much longer than manned submersibles. On one of its earliest sea trials, in 1970, RUM placed two small sonar reflectors on the sea floor, crawled away from them, and returned to find and retrieve them. It also found a third sea-floor object:
… a can of a well-known brand of stewed tomatoes. … The can was found to be the dwelling of a small and very frightened octopus. We feel [said RUM's inventors] that this is one of the first times that a mobile biological specimen has been selectively retrieved by a remotely controlled manipulator as well as record of the first sea-going anti-pollution effort by such a unit.[25]
Anderson also developed the Benthic Laboratory, first used as a communications center for Sealab II in 1965 (see chapter 6). The laboratory housed electronic equipment
Another of MPL's novel contrivances is the Deep Tow (an attempt to name this FISH, for Fully Instrumented Submersible Housing, has not been entirely successful, although almost any slender object towed behind a ship is called a fish anyway). The Deep Tow is a mapping and navigation system in the form of a package of instruments that can be lowered and towed close to the sea floor to make detailed surveys. Development of such a unit began early in the 1960s, chiefly by Maurice S. McGehee and Dwight (“Tony”) E. Boegeman, Jr. The first design problem was in the motion of the towed unit, so a roll meter, a pitch meter, and a flow meter were developed. Upward-looking and downward-looking sonars were added, and these were followed by a variety of oceanic instruments.
In 1967 one of the units was lost in 3,000 meters of water when the tow wire parted. Its exact location was known, so “after quick development of some special equipment,” Spiess and others returned to the spot six months later and successfully retrieved their unit with the aid of a second one — no small feat in ocean navigation. A special
Surveys by Deep Tow have been made in trenches and canyons, over seamounts and fans, in the Pacific and in the Atlantic. The array of instruments can include precision navigation equipment, side-looking sonar, a low-frequency sound source for seismic studies, underwater cameras, television cameras, thermometers, and a magnetometer towed behind the “fish.” All these instruments can be put to work on command and be monitored from the towing ship by way of the coaxial tow cable. For precise navigation of the ship and the Deep Tow, acoustic transponders, which answer to sound pulses from the towed unit, are placed on the sea floor. The precision capability of the instrument package was well demonstrated in 1971, when it was able to pinpoint the wreckage of five munitions ships that had been pulverized during munitions disposal. Geologic features as small as ten meters across have been mapped.
As with many of MPL's devices, the Deep Tow has more than one application: its capability of fine-scale surveying makes it equally useful to geologic mapping and to locating objects, such as shipwrecks or patches of manganese nodules, on the ocean floor.
NOTES
1. “The Age of Innocence and War in Oceanography,” Oceans Magazine, Vol. I, No. 3 (March 1969), 12.
2. San Diego Union, September 1946.
3. San Diego Union, 10 October 1946.
4. Letter to Victor C. Anderson, 13 August 1975; written two and one-half months before Wright died.
5. Memorandum of 5 May 1970.
6. Quoted in Larry L. Booda, “Research — But Emphasis on Education,” Undersea Technology (May 1966), 37.
7. SIO Annual Report, 1967, 29.
8. V. C. Anderson, “The First Twenty Years of Acoustic Signal Processing,” Journal of Acoustical Society of America, Vol. 51, No. 3, Part 2 (1972), 1063.
9. Ibid., 1064.
10. Ibid., 1065.
11. “The Age of Innocence and War in Oceanography,” Oceans Magazine, Vol. I, No. 3 (March 1969), 7.
12. “Frequency Dependence of Reverberation in the Ocean,” Journal of Acoustical Society of America, Vol. 41, No. 6 (June 1967), 1467.
13. Ibid., 1474.
14. MPL Quarterly Report, 1 April-30 June 1949, 1.
15. “Explosion Seismology at Sea,” Transactions of the American Geophysical Union, Vol. 48, No. 2 (June 1967), 416.
16. SIO Annual Report, 1970, 15.
17. Charles Corry, Carl Dubois, and Victor Vacquier, “Instrument for Measuring Terrestrial Heat Flow Through the Ocean Floor,” Journal of Marine Research, Vol. 26, No. 2 (1968), 168.
18. “The Flow of Heat Through the Floor of the Ocean,” The Sea, Vol. 3 (New York and London: Interscience Publishers, 1963), 229.
19. “A Magnetic Survey off the West Coast of the United States Between Latitudes 32° and 36° N and Longitudes 121° and 128°,” Geophysical Journal of the Royal Astronomical Society, Vol. 1 (1958), 320.
20. Ibid., 321.
21. “The Magnetic Field over the Oceans,” The Sea, Vol. 3 (New York and London: Interscience Publishers, 1963), 194.
22. Memorandum to Vacquier, 16 January 1964.
23. Edward Bullard, “The Emergence of Plate Tectonics: A Personal View,” Annual Review of Earth and Planetary Sciences, Vol. 3 (1975), 19.
24. V. C. Anderson, D. K. Gibson, and O. H. Kirsten, “Rum II — Remote Underwater Manipulator (A Progress Report),” Marine Technology Society, Sixth Annual Preprints, Vol. 1 (1970), 2.
25. Ibid., 4.