Blood Pressure and Blood Flow
During systole the semilunar valves are wide open, and the pressure within the cavities of the two ventricles and the arterial trunks on the respective sides is identical. The highest level of pressure within the ventricle and the arterial system on the corresponding side is called systolic pressure . The systolic pressure within the left ventricle and the aorta is about five times higher than the corresponding pressure within the right ventricle and the pulmonary artery. The onset of diastole and the closure of the semilunar valves signal the separation of pressure between the ventricles and the arterial trunks; pressures in the ventricular cavities drop sharply to levels close to zero; pressures in the aorta and pulmonary artery level off to a point slightly lower than that of semilunar-valve closure. The lowest pressure in the ventricues and the lowest pressure levels in the arterial trunks are termed diastolic pressure . Since
during most of diastole pressures in the two ventricles and their respective atria are identical, ventricular diastolic pressure and atrial pressure are usually the same. Normal average pressures in the adult are as follows:
|
The heart, being a pressure pump, functions properly if it can maintain an adequate flow of blood and adequate pressure. Both blood flow and blood pressure have to be regulated in response to needs of the body. The quantity of blood ejected into each circulatory system—the volume of blood flow—is the cardiac output . Cardiac output can be expressed in two ways: either as the quantity of blood ejected into the arterial system with each ventricular contraction (stroke volume ), expressed in cubic centimeters per heartbeat; or the total quantity of blood ejected into each arterial system within a minute (minute volume ), expressed in liters per minute. It is customary to use the general term "cardiac output" to indicate minute volume and to specify stroke volume as such. The maintenance and regulation of cardiac output is one of the most intricate functions of the circulatory system; it will be discussed later in this chapter in connection with the physiology of exercise.
Blood pressure commonly refers to pressures in the systemic arterial circulation. The arterial blood pressure can be maintained at its level of 100 mm Hg or above only because the blood is enclosed within a system of vessels so regulated that the same amount of blood
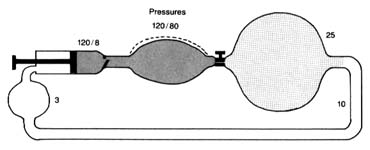
Figure 11. A circulatory model resembling the human circulation.
is pumped into it and discharged from it simultaneously. Thus if a stroke volume of 70 cc of blood is ejected into the aorta with each beat, an equal amount leaves the arterial system through the "exit"—the arterioles—into the capillary system. The arterial system is protected at one end by the aortic valves and at the other end by the sum total of the arterioles. A simplified diagram of such a system is provided in figure 11, in which a pump (at left) ejects fluid into an elastic container that has at its end a stopcock; that container is connected to a much larger container from which a system of tubes returns the fluid into the pump. The pump represents the left side of the heart; the first "closed" container, the high-pressure arterial system; the stepcock, the arterioles; the larger, "open" container, the capillary and venous reservoir of blood; the returning pipes, the larger veins; and the container at the lower left, the right atrium. The arterioles are normally in a semiconstricted state and are regulated by impulses reaching them from the central nervous system via the nerves. These impulses are capable of either constricting the arterioles further or relaxing them, thereby regulating outflow from the first reservoir and hence the arterial pressure. In the arterial system resistance to blood flow is very high; in the pulmonary arterial system where arterioles are wide open, resistance and pressures are much lower than on the systemic side. The capillary and venous systems in both circuits have no narrow area offering any resistance to flow and hence their pressure is close to zero.
This relationship between pressure, flow, and resistance is usually presented in the form of an equation derivative of Poiseuille's law and analogous to Ohm's law in electricity: pressure equals flow (cardiac output) times resistance, or P = F × R . It follows that
pressure can be maintained at a constant level only if resistance falls each time cardiac output increases. This actually takes place because of a barostat, a mechanism analogous to the thermostat that regulates pressure rather than temperature. The human barostat consists of pressure-sensitive points within the walls of some arteries. If the volume of blood ejected into the aorta increases, raising the cardiac output, the aorta becomes distended. Pressure-sensitive receptors react to the distention of arterial walls by sending signals through the nervous system to relax the arterioles just enough to let the excess blood out of the arterial system and to maintain constant arterial pressure.
The systemic circulation consists of many circuits connected in parallel (see fig. 1, p. 2). Each of these smaller circuits has its own resistance at the arteriolar level. The general equation mentioned above applies to each of these circuits as well as to their sum total; consequently, arteriolar resistance in each region determines blood flow independently within the systemic flow and pressure available. Thus, if in a given organ the arterioles were to constrict, its blood supply would be reduced, since blood would flow more easily through alternate circuits; if the arterioles relaxed in a given circuit, blood flow would increase proportionally. This mechanism, mediated less through the central nervous system than through local reflexes, controls blood flow through individual organs and parts of the body. Such a regulating mechanism (regional flow control ) provides for increased blood flow in areas where it is most needed. Thus during exercise the working muscles receive a more abundant blood supply, and after meals the digestive tract is provided with increased blood flow—all without disrupting the general balance of the total flow or altering the arterial pressure in the systemic circulation.
The blood pressure is the same in all arteries up to the beginning of the arterioles. There the pressure falls abruptly from a systolic level of 120 mm Hg to 25 mm Hg in the capillaries, a level just sufficient to drive the blood into the venous system through the narrow capillary channels. On the venous side blood flows slowly toward the heart. The veins have virtually no driving power, and the blood flow is aided only by the venous valves and by the massaging action of the various muscles of the body.
The aorta and its principal branches are, as mentioned, elastic
vessels. This property plays an important part in causing the blood to flow through the body evenly rather than intermittently. During systole all the elastic arteries are expanded by the addition of blood ejected from the left ventricle; the elastic vessels then revert to their original size as the excess blood drains into the capillaries. If blood were ejected into a system of totally rigid pipes, then forward flow of blood would occur only during systole and would stop during diastole. Elastic tissue in arterial walls makes for a reservoir of variable capacity that acts as a pressure and flow equalizer. This principle is often used in perfume and cologne atomizers: simple dispensers with one bulb spray only when the bulb is squeezed; dispensers with a double bulb are capable of spraying continuously because the second bulb, which is not squeezed, distends with air when the first bulb is squeezed and acts as a pressure and flow equalizer.
The sudden dilation of the aorta by the blood ejected from the left ventricle is transmitted along the arterial system as a wave of elastic displacement of the arterial wall, usually referred to as the arterial pulse . The pulse is an index of the heart rate but also reflects the quantity of blood ejected into the aorta, the mode of its injection, the elasticity of the larger arteries, and the condition of the closed arterial system. It obviously provides important and readily obtainable information concerning the circulatory apparatus. Study of the arterial pulse has been a basis of diagnosing disease for many centuries.
The circulation through the pulmonary circuit is much simpler than the systemic circulation. It is confined to a single organ, the lungs; therefore there is little need for the regulation of blood flow (see fig. 1). Its pressure is low because, under normal circumstances, pulmonary arterioles are wide open and offer little resistance to flow, and nervous regulation of pulmonary arterioles is practically nonexistent. Consequently, the fall in pressure from the arteriolar side to the capillary side of the pulmonary circulation (pressure gradient ) is very low, less than 10 mm Hg.
The final destination of the circulatory system is the capillary network in both circuits. In it the exchange between blood and tissue, and between blood and air, takes place. The thin walls of the capillaries are a semipermeable membrane, a partition through which certain chemical substances can pass back and forth: gases,
water, and simple chemical substances, such as sodium, potassium, and glucose can leave or enter the blood as needed, but blood cells and large molecules (for example, proteins) remain in the blood. The capillaries thus act as an intermediary in two vital body functions: (1) blood-gas exchange, the acquisition of oxygen in the lungs and its delivery to the tissues coupled with the taking of carbon dioxide from the tissues and its excretion through the lungs (by means of a chemical reaction between the gases and hemoglobin, the red dye contained in the red blood cells); and (2) the physical process of regulating the water content in the tissues and blood and permitting an exchange of electrolytes (such as sodium and potassium) between tissue fluid and the blood. Water and chemical substances dissolved in the blood plasma can enter and exit as needed, driven by two forces: the osmotic pressure on the two sides of the capillary walls and the hydrostatic pressure within them. Each substance dissolved in the blood on one side of the capillary wall, and in the tissue fluid on its other side, reaches equal concentration in the two media. The pressure in the capillaries, about 25 mm Hg, maintains a fluid balance within the vascular system, as this pressure is identical with the osmotic pressure exerted by plasma proteins and electrolytes. If the hydrostatic pressure increases, or the osmotic pressure falls, then fluid leaves the vascular system, increasing the fluid content of tissues—a situation that may lead to edema (see chap. 5). A fall in hydrostatic pressure or increase in osmotic pressure draws fluid into the vascular system, raising the volume of circulating blood. These factors play an important role in maintaining blood volume at an optimal level. Water and solutes are quickly and efficiently exchanged in the capillary system.