6.5.3—
Photorespiration
The oxidation of glycollate in the peroxisome is accompanied by a consumption of oxygen and ultimately results in the release of carbon dioxide. The net result is a respiratory gas exchange where the substrate of respiration is glycollate rather than glucose. Since glycollate is only formed in light this respiration is light-dependent and is called photorespiration.
The direct measurement of oxygen uptake during a net photosynthetic release of oxygen, or CO2 release during net photosynthetic CO2 fixation is impossible. However, indirect methods have demonstrated that photorespiration occurs in plants and that the rate of CO2 loss in light is higher than that in the dark. Measurements of photorespiration rates vary considerably with the assay method used and can only be considered as approximations of the magnitude of the process. Nevertheless, it is becoming clear that the process of photorespiration has great importance in decreasing the rate of net photosynthesis in many plants.
Photorespiration can be detected by measuring the flux, that is the simultaneous uptake and release, of oxygen or carbon dioxide of photosynthesizing tissue by using isotopic tracer methods. The uptake of 18 O2 by leaves during photosynthesis has been detected but experiments using 18 O2 have generally given equivocal results which have been difficult to interpret.
The measurement of carbon dioxide flux during photosynthesis is much more convenient and depends upon the accurate measurement of carbon dioxide concentration in the atmosphere using an infra-red gas analyser and a simultaneous measurement of the total activity of supplied 14 CO2 with an ion counter or Geiger-Müller counter. When a plant is placed in a closed system in light, there is a rapid uptake of carbon dioxide and the CO2 concentration of the atmosphere around the plant decreases to a concentration at which the uptake of CO2 exactly balances the output of CO2 by the plant (Fig. 6.9). This concentration of carbon dioxide is called the CO2compensation point of the plant and is usually measured in parts per million (ppm) of CO2 in air. Plants such as tobacco, sunflower and wheat have compensation points, ranging from 35 to 100 ppm indicating a marked loss of CO2 (i.e. photorespiration) during photosynthesis, but others such as maize and sugarcane have compensation points of 3 to 10 ppm indicating a low loss of CO2 or a low photorespiration rate. If photosynthetic carbon fixation is similarly measured in a closed-system but in an atmosphere containing 14 CO2 , the CO2 arising from, the plant by photorespiration will, over short time periods, be 12 CO2 . Thus, while a decrease in the CO2concentration of the atmosphere around the plant will occur, the radioactivity of 14 CO2 in the atmosphere will appear to decrease at a faster rate because of the efflux of unlabelled CO2 from the plant, and continues to decrease even after the compensation point is reached (Fig. 6.9a). In a plant which
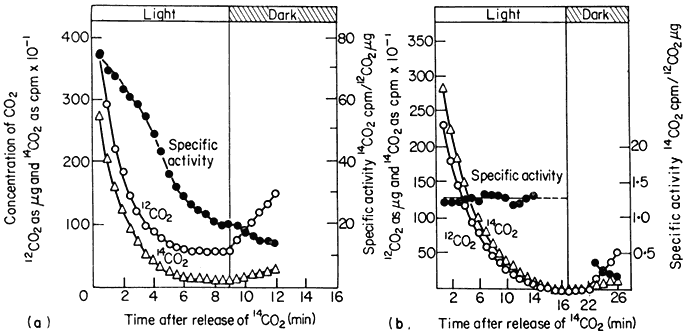
Figure 6.9
The concentration of CO2 and 14 CO2 and the specific activity of 14 CO2 around
a detached sunflower leaf (a) and a detached maize leaf (b) during illumination
and in subsequent darkness in an atmosphere of 21% oxygen and at 21°.
(Reproduced with permission from Hew et al., 1969.)
is photorespiring, therefore, a decrease in the specific radioactivity of 14 CO2 will occur, while in a plant with no photorespiration the uptake of 14 CO2 will not be accompanied by an efflux of 12 CO2 and the specific radioactivity of the 14 CO2 in the atmosphere will remain constant (Fig. 6.9b). In the dark the specific radioactivity of the 14 CO2 decreases with the efflux of 12 CO2 and the rate of decrease of this activity is a measure of dark respiration. The rate of photorespiration as measured by these methods has been shown to be 1.5 to 2.5 times that of dark respiration, while in plants with low photorespiration, such as maize, loss of CO2 in light is only a fraction of the rate of CO2 loss in the dark. This method clearly indicates that photorespiration occurs in some species while it is absent in others.
Another method which has been used to detect differences in the rate of photorespiration and dark respiration was devised by Zelitch (1968). In this method leaf discs are allowed to fix 14 CO2 for a period of 45 to 60 minutes. The remaining 14 CO2 is then quickly flushed out of the closed system, and the release of 14 CO2 from the tissue into CO2 -free air is measured over short periods in the light and the dark. The method is based on the assumptions that the rate of 14 CO2 loss is a measure of total CO2 loss i.e. that the specific radioactivity of the CO2 evolved remains constant over short time periods and that low CO2 tensions have no effect on the loss of CO2 . These assumptions may not be valid for all photosynthetic tissues but within these limitations the method is a very rapid and sensitive means for detecting photorespiration. Photorespiratory loss of CO2 in tobacco has been shown by this method to be 2 to 5 times higher than in the dark while CO2 loss from maize is not detectable (Fig. 6.10a). This method has been similarly used to detect photorespiration in other species.
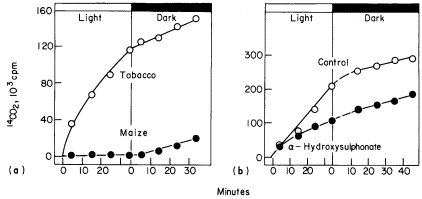
Figure 6.10a
A comparison between the release of 14 CO2 by tobacco and maize discs
in the light and the dark after previously being supplied 14 CO2 in light.
(b). The effect of a -hydroxysulponate on the release of
14 CO2 from tobacco leaf discs in the light and dark.
(Reproduced with permission from Zelitch, 1968.)
Photorespiration is not simply a stimulation of dark respiration since the two process of photorespiration and dark respiration respond differently to changes in oxygen concentration. Dark respiration of leaves has an optimal rate at about 2% oxygen in air and any increase in the O2 concentration up to 100% does not increase the rate (Fig. 6.11). Net photosynthesis however is inhibited
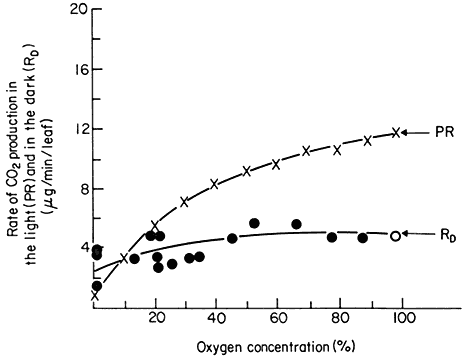
Figure 6.11
The effect of oxygen concentration on the rate of photorespiration
(PR) and dark respiration (RD ) of detached soybean leaves.
(Reproduced with permission from Forrester et al., 1966.)
by oxygen, a phenomenon called the Warburg effect, and this is attributable to an increase in the rate of photorespiration rather than to an inhibition of photosynthesis per se . A reduction in the oxygen concentration in the atmosphere around a leaf from the ambient 21% O2 of air has been shown to lower the compensation point and increase the rate of net photosynthesis, while an increase in concentration raises the compensation point and lowers net photosynthesis (Forrester et al., 1966). Plants with photorespiration have been found to evolve CO2 at a high rate when they are transferred to darkness after a period of photosynthesis. This post-illumination CO2 burst lasts for a few minutes before a steady dark respiration is established and the magnitude of the burst has been found to depend on the light intensity and oxygen concentration during the previous period of photosynthesis: the CO2 -burst increases with an increase in light intensity and decreases with a decrease in O2 concentration (Tregunna et al., 1961). This burst has been explained as being due to the continued slow oxidation, in the dark, of a product produced in photosynthesis after the uptake of CO2 by photosynthesis has stopped. Photorespiration therefore appears to have different characteristics than dark respiration and to have a different substrate.
The substrate for photorespiration is thought to be glycollic acid. Glycollic
acid production in leaves and in chloroplasts is stimulated by an increase in oxygen concentration as is photorespiration, while the inhibition of glycollate oxidation by HPMS has been found to lower the rate of photorespiration in leaf discs to that of dark respiration (Fig. 6.10b). Photorespiratory loss of CO2 is also stimulated by the infiltration of leaves with glycollic acid while acetate has no effect on this rate. Thus the oxidation of glycollate, mediated by the leaf peroxisomes results in a photorespiratory loss of carbon dioxide by the leaf.
Plants with low compensation points are said to lack photorespiration. These plants are C4 plants, that is, the primary carboxylation step is catalysed by phosphoenolpyruvate carboxylase rather than by RBP carboxylase. This efficient fixation of CO2 has been postulated to preclude the formation of glycollate, but maize for example, has been shown to possess a glycollate pathway (Osmond, 1969) and peroxisomes occur in the mesophyll cells of the leaf. Presumably in such plants glycollate may be formed and oxidized, but the efficiency of refixation of the CO2 resulting from glycollate oxidation is such that no CO2 is released from the plant and hence no photorespiration is detected.
Estimates from various plant species suggest that from 15 to 40% of the carbon fixed in photosynthesis is lost by photorespiration. There is no unequivocal evidence to show that energy in the form of ATP is recovered during the oxidation of glycollate and the process appears to be a wasteful one in terms of energy conservation. An important question therefore arises: does photorespiration serve a useful function or is it simply an inevitable consequence of photosynthesis being carried on in an atmosphere of 21% oxygen? It has been suggested that photorespiration might act as a 'safety valve' for the plant, in that, under conditions of high light intensity and low carbon dioxide concentration, the oxidation of glycollate would consume both excess reduced NADP+ and excess oxygen, which would serve to protect the chloroplast from photo-oxidative damage. If the process of photorespiration imposes limitations on the growth of plants, it would be expected that there would have been some selective pressure to eliminate it by natural selection during the evolution of the higher plants. The occurrence of C4 plants, which have low photorespiration, may represent such an evolutionary step to correct for the presence of photorespiration by the development of an efficient CO2 -fixation mechanism. Since variations in the rate of photorespiration occur within a single species (Zelitch, 1971) it may be possible to select artificially for low photorespiration in crop plants and consequently increase net photosynthetic productivity and crop yield.