Silicic Domes and Extrusion of Viscous Lava
Extrusions of viscous lava form domes in many different volcanic settings during the evolutionary stages of volcanic fields. Because viscosity depends on thermal, compositional, and textural factors, lava compositions from andesite to rhyolite can display relatively high viscosities (105 to 1012 Pa/s; Murase and McBirney, 1973); therefore, the rheology of such viscous fluids is the dominant feature in controlling extrusion mechanisms.
Common Geologic Settings
Table 5.1 lists some common occurrences of domes in various volcanic settings. The most common are lava domes of dacite and rhyolite that form before and after caldera collapse. These extrusions, which generally appear in groups of at least several vents along major structural features such as ring faults and resurgent cores in calderas, are manifestations of larger bodies of differentiated magma at depth. Silicic domes are frequently associated with composite cones in late evolutionary stages (see the discussion in Chapter 7). In these cases, domes form either plugs in the central vent region of the cone or parasitic vents around the flanks of a composite cone. Silicic domes may also occur as isolated extrusions along major tectonic lineaments such as grabens near plate boundaries and rifts. In addition, domes appear in or near explosion craters that are created by gaseous bursts preceding the extrusion of viscous lava.
Evolution and Internal Structure
Figure 5.1 illustrates a common evolutionary scheme for silicic domes. Preceding activity is usually marked by the passive or explosive release of gases at depth above a rising mass of viscous lava. Such a release might be characterized by only fumarolic activity and bulging ground. On the other hand, the gases can be released in violent explosions when high-pressure gases from either vaporized groundwater or the gas-rich top of the magma body burst through the ground surface in crater-producing eruptions. Because of the high viscosity of dome lavas, the extrusion process may continue over periods of months to years. Figure 5.2 depicts types of domes, including both endogenous and exogenous forms. The lava structures formed at domes are distinctive because of the complex rheology as a result of lavas ranging from viscous to
|
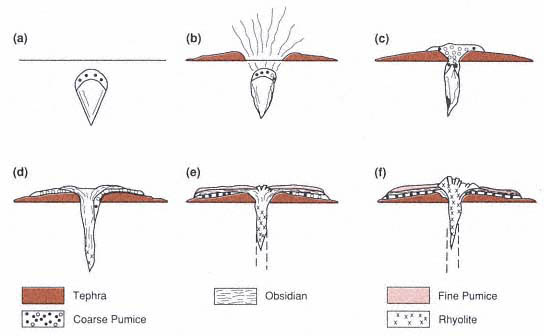
Fig. 5.1
Idealized diagram showing the eruptive evolution of silicic domes. (a) Magma with volatile-rich
pumiceous top approaches surface. (b) Initial pyroclastic eruption in which volatiles and pumice
erupt to form tuff ring. (c) Cessation of explosive eruptions and emplacement of coarsely pumiceous
rhyolite lava. (d) Extrusion of obsidian over coarse pumice. (e) Development of a mantle of finely
pumiceous lava. (f) Final extrusion of crystalline rhyolite.
(Adapted from Fink, 1983.)
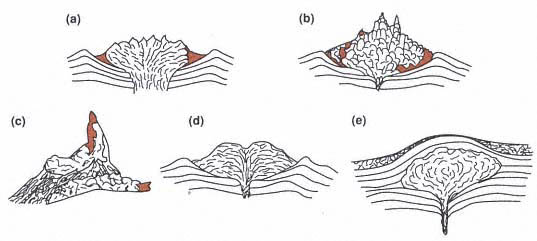
Fig. 5.2
Forms of endogenous and exogenous domes, showing various fracture foliations. (a) Upheaved,
exogenous dome similar to that illustrated in Fig. 5.1. (b) Peléean dome showing vent spines,
blocky fracture, and development of talus aprons. (c) Detail of vent spine of Mont Pelée
(from Lacroix, 1904).
(d) Exogenous dome that discharges viscous flow lobes from a summit vent. (e) Intrusive
dome in which viscous magma body is emplaced just below the earth's
surface and maintains a carapace of upwarped strata.
(Adapted from Williams and McBirney, 1979.)
partly solidified. These lava flows exhibit behavior varying from that of a Bingham fluid to that of a brittle, plastic rock (Shaw, 1972; Hulme, 1974; Fink, 1980a).
The surfaces of silicic lava domes are characterized by a wide variety of textures that produce variations of color, flow layering, crystallinity, and vesicularity. Fractures and foliations in the lava are useful for mapping the surface of domes—and especially for locating the vent area (Fink, 1983; Fink and Pollard, 1983). Figure 5.3 illustrates the development of foliations in lava domes, and Fig. 5.4 shows surface distributions of flow features at an obsidian flow.
Silicic dome lavas generally develop a textural stratigraphy related to their cooling, vesiculation, and crystallization during extrusion (Fig. 5.5; Figs. 5 and 7 in Fink and Manley, 1987). However, such textural features can easily be mistaken for those that develop during the welding of pyroclastic flows. Basal tephra layers are often found because dome extrusions are commonly initiated by pyroclastic eruptions of the gasrich tops of silicic bodies; but a flow breccia, if found above the tephra layer, can distinguish dome/lava-flow stratigraphy from that of welded ash flows. Obsidian layers near the base and top of the flow stratigraphy are similar to the quench vitrophyres that develop in similar positions in welded ash flows. The central portion of dome/lava-flow stratigraphy consists of crystalline lava, which frequently contains lithophysae. This textural type grades upwards into glassy and pumiceous zones that can be mixed into the crystalline part of the flow; in such a complex textural case, it is again difficult to distinguish the lava textures from those of welded pyroclastic deposits. However, scanning electron microscope analysis may differentiate the origin of the complex textural character. For example, vestiges of shard and pumice textures, revealed by electron microscopy, are very flattened and elongated in welded tephra. This distinction is very difficult to observe in rheomorphologically deformed tuffs, and field relationships are used to determine the dome origin of silicic volcanic rocks (Bonnichsen and Kauffman, 1987). Fink and Manley (1987) noted that small domes display little textural variation and usually display structural evidence of their vent geometry. This is not the case for larger silicic lava extrusions because they can exhibit all the textural features discussed above.
Fink and Manley (1987) discuss three principal steps in the development of textures in silicic lava flows and domes.
(1) Crystallization and the accompanying release of magmatic volatiles is strongly related to cooling rate and flow stresses.
(2) Microcracks, formed during the lava's advance, allow gases to escape upward.
(3) Quenching of the upper surface creates a crust of high yield strength that resists flow deformations.
These three processes result in the formation of a gas-rich, low-density layer below the crust that can rise buoyantly. This layer eventually may break through the surface crust, producing the banded appearance of silicic lava flow surfaces. Bonnichsen and Kauffman's (1987) summary for the development of rhyolite lava flow textures is shown in Table 5.2.