6.4—
Glyoxysomes
There are many plant species in which lipid is the main storage material in the cotyledons or endosperm of the seed. During the first few days of seed germination there is a dramatic decrease in the lipid content of the seed and sugars, principally sucrose are formed. These sugars are subsequently translocated to the growing embryo or embryonic axis. This lipid to carbohydrate conversion has been correlated with an increase in the activity of the glyoxysomal enzymes, malate synthetase and isocitrate lyase, and as germination proceeds and the lipid reserves are depleted, the number of lipid storage bodies or spherosomes decrease and there is a drop in the activities of malate synthetase and isocitrate lyase. The elucidation of the metabolic processes involved in this process of gluconeogenesis, ultimately led to the isolation of particles in which were localized the crucial enzymes of this pathway. These particles were found to be morphologically similar to animal peroxisomes and were called glyoxysomes.
The breakdown of lipids is initiated by their hydrolysis to fatty acids. Triglycerides are hydrolized to glycerol and fatty acids by the enzyme lipase while phospholipids are hydrolized by phospholipase. The resultant long-chain fatty acids are subsequently degraded by the successive removal of 2-carbon fragments in the process of b -oxidation.
6.4.1—
b -Oxidation
In the process of b -oxidation the removal of each 2-carbon fragment from a long chain fatty acid involves a succession of five reactions (Fig. 6.3). The sequence is initiated by the activation of the substrate by coenzyme-A, catalyzed by the enzyme fatty acid thiokinase in the presence of ATP. The fatty acyl-CoA is then oxidized by the removal of hydrogen from carbons 2 and 3 of the chain and a double bond between 2 and 3 is formed. In this reaction the hydrogen is transferred to FAD. This reacts with molecular oxygen to produce peroxide which is broken down by catalase, and results in the uptake of one mole of oxygen for every two moles of fatty acyl-CoA oxidized. The unsaturated acyl-CoA produced is hydrated to form 3-hydroxy acyl-CoA, the reaction being catalysed by enoyl hydratase or crotonase, and this product then oxidized, with a concomitant reduction of NAD+ , to form a 3-keto acyl-CoA by the action of hydroacyl-CoA dehydrogenase. In the final reaction the 3-keto acyl-CoA is cleaved by the enzyme thiolase into acetyl-CoA and a new fatty acyl-CoA. The fatty acyl-CoA re-enters the reaction sequence and successive acetyl-CoA units are generated.
Fatty acids with an even number of carbons yield only acetyl-CoA units but those with an odd number of carbons result in the formation of acetyl-CoA and propionyl-CoA. In plant tissues propionyl-CoA is degraded by a modified b -oxidation sequence to yield acetyl-CoA and carbon dioxide.
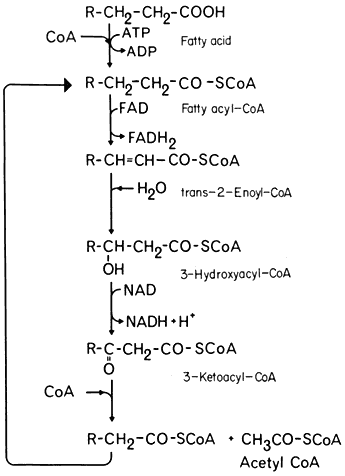
Figure 6.3
The b -oxidation pathway.
The b -oxidation pathway was unequivocally demonstrated to occur in plant tissues by Stumpf and Barber (1956) who showed that long chain aliphatic acids were oxidized to carbon dioxide by mitochondrial preparations from germinating peanut cotyledons, when these preparations were supplemented with a number of cofactors including ATP, CoA, and NAD+ . The rate of release of 14 CO2 from specifiically labelled butyric and palmitic acids was consistent with their degradation by b -oxidation and subsequent oxidation by the TCA cycle.
6.4.2—
The Glyoxyllate Cycle
The acetyl-CoA derived from fatty acid breakdown in germinating seeds could be consumed by the TCA cycle, as indicated by the experiments of Stumpf and Barber (1956). However this would not result in the net formation of a glucose precursor since for each molecule of acetyl-CoA consumed two molecules of carbon dioxide would be produced. Furthermore, it was known at the time that little of the fatty acid was oxidized to CO2 but instead contributed to a net synthesis of sugars.
The problem of how acetyl-CoA was converted to a glucose precursor was solved by the discovery of the glyoxyllate cycle, by Kornberg and Krebs in 1957. This cycle represents a modification of the tricarboxylic acid cycle in which two molecules of acetyl-CoA are consumed and a molecule of succinic acid is formed (Fig. 6.4). Five enzymes are involved in this process three of which,
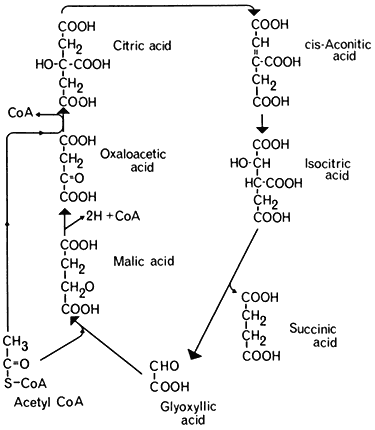
Figure 6.4
The glyoxyllate cycle.
citrate synthetase, aconitase, and malic dehydrogenase are components of the TCA cycle. The remaining two enzymes, the key enzymes of the glyoxyllate cycle, are isocitric lyase (isocitratase) and malate synthetase. The first reaction of the glyoxyllate cycle, catalyzed by citrate synthetase, is the condensation of oxaloacetate and acetyl-CoA to form citrate, which is then converted to isocitrate by the action of aconitase. The next reaction, unique to this cycle, is the cleavage of isocitrate into succinate and glyoxyllate catalyzed by isocitrate lyase. One of the products of this reaction, glyoxyllate, is then condensed with a second molecule of acetyl-CoA under the catalytic action of malate synthetase, to produce one molecule of malate. Malate is then oxidized by malate dehydrogenase to oxaloacetate with the concomitant reduction of NAD+ . The overall equation for the cycle is therefore:

Succinate produced by the glyoxyllate cycle can then be converted to hexose by conversion to oxaloacetate, by the action of succinic dehydrogenase and fumarase. The oxaloacetate is converted to phosphoenolpyruvate, a reaction catalised by phosphoenolpyruvate carboxykinase,

and the phosphoenolpyruvate converted to glucose by a reversal of the reactions of the Embden-Meyerhof-Parnas pathway. Thus four molecules of acetyl-CoA will give rise to two molecules of succinate and this in turn will result in the
formation of one molecule of glucose and the loss of two molecules of carbon dioxide (Fig. 6.5).
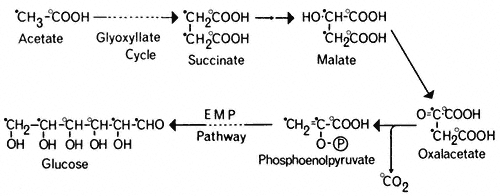
Figure 6.5
The pathway of incorporation of [14 C] from [1-14 C]-acetate (O) or
[2-14 C]-acetate (

The glyoxyllate cycle was first demonstrated in the bacterium Pseudomonas (Kornberg & Krebs, 1957) grown on two-carbon compounds, and has since been shown to operate in many micro-organisms and plant tissues. The evidence for the cycle is based on the presence of the two key enzymes, malate synthetase and isocitrate lyase, and on the distribution of 14 C in organic acids, sugars and carbon dioxide when the tissue is supplied with specifically labelled [14 C] acetate. Malate synthetase and isocitrate lyase are found in a wide variety of plant tissues (Carpenter & Beevers, 1958), particularly in fatty seedlings where they increase in activity during germination. Similarly the activities of aconitase and citrate synthetase increase in these tissues during germination. Incubation of castor bean endosperm tissue with [I-14 C] acetate or [2–14 C] acetate results initially in the formation of [14 C] malate, and subsequently the radioactivity from [I-14 C] acetate results in about an equal labelling of CO2 and sucrose. In the cotyledons of germinating peanut and sunflower seedlings (Bradbeer & Stumpf, 1959) and castor bean endosperm (Canvin & Beevers, 1961), [1-14 C] acetate was converted to carboxyl-labelled malate and to sucrose in which the glucose moiety was labeled in the 3 and 4 carbons, while [2-14 ]C acetate gave rise to malate labelled in the methylene carbons and to sucrose where the glucose moiety was labelled in the 1, 2, 5 and 6 carbons. These patterns or labelling of the products of acetate metabolism are consistent with the operation of a glyoxyllate cycle in these tissues (Fig. 6.5).
6.4.3—
Metabolic Functions of the Glyoxysome
The reactions of the glyoxyllate cycle and the pathway of b -oxidation were generally thought to be associated with the mitochondria since the enzymes of these pathways were usually present in the mitochondrial fraction isolated from cell homogenates. Elegant studies by Beevers' group at Purdue University however showed that sucrose density centrifugation of crude particulate fractions of castor bean endosperm resulted in the separation of three distinct bands of
particles which were identified as proplastids, mitochondria and a new particle sedimenting at a high density. Enzymes of the glyoxyllate cycle, isocitrate lyase and malate synthetase were found exclusively in this dense particle together with catalase and a large proportion of the glycollate oxidase of the gradient. On the other hand citrate synthetase and malate dehydrogenase were associated with both the mitochondrial band and the band containing the new particle, while succinic dehydrogenase, fumarase and NADH oxidase were located exclusively in the mitochondrial band with cytochrome oxidase (Fig. 6.6).
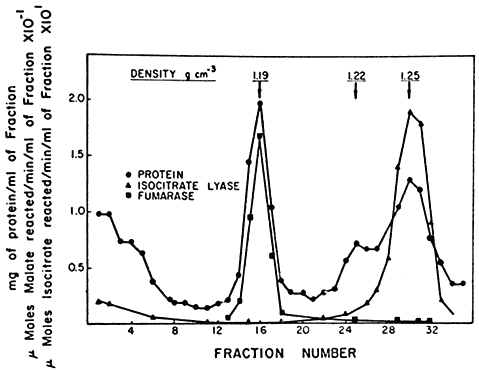
Figure 6.6
The distribution of protein, fumarase and isocitric lyase
after sucrose density gradient separation of the components of
a crude particulate fraction of the endosperm of germinating castor bean.
(Reproduced with permission from Breidenbach & Beevers, 1967.)
The enzyme distribution indicated that the TCA cycle enzymes were located in the mitochondria while the enzymes of the glyoxyllate cycle were compartmentalized in the denser particle. These particles were therefore called glyoxysomes (Breidenbach & Beevers, 1967; Breidenbach et al., 1968). The isolated glyoxysomes were found to be organelles with a single unit membrane bounding a finely granular matrix. Similar structures were recognized in electron micrographs of intact castor bean endosperm tissue indicating that the isolated organelles were not artefacts of the isolation and centrifugation processes. Since the finding of glyoxysomes in endosperm tissue, they have been reported to be the site of the glyoxyllate cycle in the cotyledons of a number of fatstoring seeds including those of watermelon, sunflower, peanut and cucumber. Microbodies containing catalase and enzymes of the glyoxyllate cycle have also been found in yeast (Szabo & Avers, 1969) and although these have been called peroxisomes they clearly have the enzyme complements of glyoxysomes.
In addition to glyoxyllate cycle activity, the glyoxysomes were shown to be the site of b -oxidation in castor bean endosperm (Cooper & Beevers, 1969b; Hutton & Stumpf, 1969). Glyoxysomes isolated from this tissue oxidized palmitoyl-CoA to acetyl-CoA with a concomitant reduction of NAD+ and uptake of oxygen. Addition of [14 C] oxaloacetate during this reaction resulted in the formation of [14 C] citrate and [14 C] malate from palmityl-CoA indicating that the acetyl-CoA produced by the b -oxidation process was consumed in the glyoxyllate cycle also located in the organelle (Cooper & Beevers, 1969b). Similarly, ricinoleate, linoleate and palmitate were oxidized by glyoxysomes of castor bean with the formation of acetyl-CoA, and three enzymes of the b -oxidation complex, crotonase, b -ketothiolase, and b -hydroxyacyl dehydrogenase were found to be located specifically in the organelle (Hutton & Stumpf, 1969). The activity of the b -oxidation complex in this tissue during the germination of the seed was found to parallel the increase in activity of the glyoxyllate cycle enzymes indicating that an integrated system for lipid utilization develops together with the formation of the glyoxysome.
The principal pathways of gluconeogenesis are therefore compartmentalized in a single organelle, the glyoxysome. The complete pathway of gluconeogenesis however involves at least three organelles, the spherosome, the glyoxysome and the mitochondrion. The reactions are initiated by a hydrolysis of lipids in the spherosome by the action of lipase, and the glycerol and fatty acids produced diffuse out of the organelle. Glycerol is utilized directly by the EMP pathway in the cytosol and contributes to sucrose synthesis (Beevers, 1956) while the fatty acids diffuse into glyoxysomes which are located near the spherosome. In the glyoxysome the fatty acid is oxidized in the b -oxidation pathway and the acetyl-CoA released is converted to succinate by the action of the glyoxyllate cycle enzymes located in the organelle. Although the b -oxidation pathway is reversible, the equilibrium is presumably maintained in a catabolic direction by the removal of peroxide, produced in the oxidation step of the pathway, by the catalase present in the glyoxysome. Reduced NAD+ produced in these reactions is probably oxidized in the mitochondria. Succinate produced in the glyoxysome is further metabolized to oxalacetate and finally to phosphoenolpyruvate in the mitochondria since succinic dehydrogenase and fumarate are not component enzymes of the glyoxyllate cycle and are absent from glyoxysomes.
This compartmentalization of the b -oxidation complex and the glyoxyllate cycle together in an organelle discrete from the mitochondrion is probably the reason why acetyl-CoA is utilized in gluconeogenesis in plant tissues rather than being oxidized to carbon dioxide and water as in animal tissues. Free acetyl-CoA-is presumably not released from the glyoxysome and made available for oxidation in the mitochondrion, while consumption of succinate, the final product of the glyoxyllate cycle, by the mitochondrion, would not stimulate the rate of oxidation in the TCA cycle since this can only occur by increasing the supply of acetyl-CoA. Some measure of control of competing metabolic pathways is therefore achieved by separation of these reactions within different organelles.