Six—
Biomass and Energy Consumption of the South Georgia Population of Southern Elephant Seals
Ian L. Boyd, Tom A. Arnbom, and Michael A. Fedak
ABSTRACT. The total annual energy expenditure was estimated for different age and sex classes of southern elephant seals, Mirounga leonina , that breed at South Georgia. The estimated energy costs of reproduction, growth, foraging, and molt were used to calculate an annual energy budget for individuals in each age and sex class. This was combined with population size and age structure to estimate population energy requirements. The estimated average metabolic cost of maintenance for adult males and females was 0.17 and 0.39 MJ/year, respectively. Male biomass accounted for 63% of the total population biomass (222,903 metric tonnes), and the metabolic power for the whole population averaged over one year was 190 MWatts. Total energy expenditure of each age class declined during the first two years but then began to increase because of the onset of reproduction in females and because of increased energy costs of foraging and growth in males. Foraging accounted for 63.2% and 68.2% of the annual energy budget in males and females, respectively. The total annual energy expenditure was 6.01 × 109 MJ/year, and 59% of this was accounted for by males. The gross energy requirement was 7.89 × 109 MJ/year. The production efficiency was 8.2% Average daily gross energy intake during potential foraging periods was 77.3 and 43.2 MJ/day for males and females, respectively. This suggested a capture rate of 0.26 and 0.15 kg of fish or muscular squid per dive for males and females, respectively. Biomass of food consumed depended on assumptions about diet composition. If southern elephant seals at South Georgia fed exclusively on squid, the consumption biomass was 2.28 × 106 tonnes/year.
Total food or energy requirements of pinnipeds are important for assessing the impact of their populations on marine resources and the potential effects of changes in the abundance of prey on population size and distribution. Within a pinniped population, demand for food can vary between different age and sex classes because the abundance of individuals is a declining function of age and there are differing nutritional requirements for growth
or reproduction within each age and sex class. Although an estimate of the total energy requirements of a population can be useful, it is often more instructive to estimate energy demand for different age and sex classes because this can help to identify which parts of a population are most vulnerable to food shortage or which have the greatest potential impact on food resources.
This study is concerned exclusively with southern elephant seals, Mirounga leonina , that breed at South Georgia. This population has remained stable since the 1950s (Laws 1960; McCann and Rothery 1988), but this study has clear implications for interpretations of factors leading to declining numbers of southern elephant seals in other populations (van Aarde 1980; Skinner and van Aarde 1983; Hindell and Burton 1987; Hindell 1991). In addition, calculation of the total energy requirements of a population involves the synthesis of data about age structure, growth, and energy costs of reproduction, molt, locomotion, and foraging. Therefore, it is a means of highlighting the strengths and weaknesses of current data sets within a structured model.
Estimates of annual food consumption among phocids have been made for harp, Phoca groenlandica (Lavigne et al. 1985), gray, Halichoerus grypus (Fedak, Anderson, and Harwood 1981), and southern elephant seals (Laws 1977; McCann 1985). The estimates for harp and gray seals were based on empirical and best estimates of the energy budgets of individuals (derived from analyses of carcass composition), energy investment in reproduction, and measured metabolic costs of maintenance, growth, and age-dependent survivorship, combined with diet composition and assimilation efficiency to estimate food consumption. In contrast, estimates for southern elephant seals were largely based on crude estimates of food consumption per unit of biomass, which gives little scope for estimating the effects of changes in prey abundance on the population or of changes in the population parameters on food consumption. More recently, studies using time-depth-temperature recorders have provided significant information about the foraging of southern elephant seals (Boyd and Arnbom 1991; Hindell 1991) in terms of both swimming behavior and the location of prey in the water column. This has been augmented by detailed studies of the diet (Rodhouse et al. 1992) and population size (McCann and Rothery 1988).
The main objectives of this paper were to: (1) bring together recent information about southern elephant seal energetics (Fedak et al., this volume) by considering energy consumption in the broad context of the South Georgia population; (2) show how energy demand changes in relation to age both for individuals and for whole age classes; (3) contrast patterns of energy demand in the two sexes; and (4) provide an indication of what interaction there is likely to be between elephant seals and commercial fisheries in the Atlantic sector of the Southern Ocean.
|
Methods
Population Parameters, Growth, and Biomass
Annual Survival Rate
T. S. McCann's (1985) revision of R. M. Laws's (1960) life tables for male and female southern elephant seals was used to provide statistics on survival rates from birth to 20 years of age (table 6.1). These were based on cross-sectional age distributions from material collected by Laws and from a collection made in 1978. Comparison was made between these survival rates and those for the Macquarie Island stock (Hindell 1991), where longitudinal data have been collected on survival rates of individuals. Estimates of survivorship differed for the two stocks, especially survival rates up to 1 year and in the 5- to 12-year age classes (ibid.). Given that the Macquarie Island stock has been in decline while the South Georgia stock has been stable in recent years (Hindell and Burton
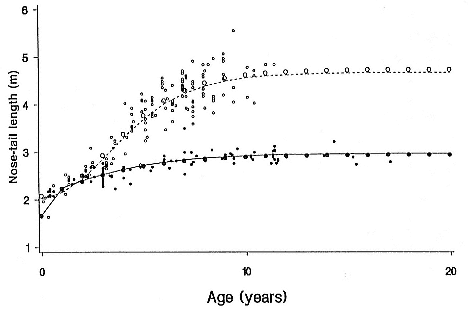
Fig. 6.1
The relationship between nose-tail (N–T) straight length and age for female (dots)
and male (open circles) southern elephant seals. Data were from Laws (1953), and
Gompertz growth models (large dots and solid line for females; large open circles
and dashed line for males) were fitted by least squares regression.
The regression equations were:
female length = 168 + 128 × e(–e – 0.028[age – 4.5])
male length = 202.4 + 264.9 × e(–e – 0.039[age – 39.73])
1987; McCann and Rothery 1988), reduced survival at Macquarie Island would be sufficient to account for this difference. The maximum longevity for a female elephant seal from South Georgia is 21 years (Arnbom et al. 1992), which is close to the 20 years predicted by McCann's life table.
Survival rate estimates for males are less certain than those for females. Males were assumed to become sexually mature at 4 years of age but did not breed until they were > 6 years of age (Laws 1953).
Growth
For the purposes of this analysis, we define the mean size of seals in any age class as the size at the end of the period of reproductive investment each year (the end of the breeding season). For seals < 1 year old, this means from the time of nutritional independence or weaning. Laws (1953) gave the nose-tail curved lengths of male and female elephant seals in relation to age. Curved lengths are about 10% greater than nose-tail straight length, so Laws's data were corrected to the equivalent nose-tail
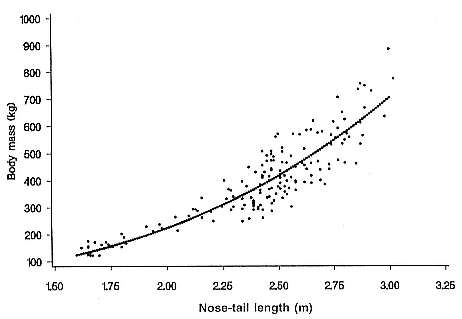
Fig. 6.2
The relationship between nose-tail straight length and mass for 162 female elephant
seals. The line was fitted by least squares regression (length = 22.61 + 25.33 mass3 ).
straight length and asymptotic growth curves were fitted to the data by least squares regression (fig. 6.1). The predicted values for each age were used as the mean length for a given age (table 6.1). The model overestimated weaning mass of both sexes, so the values given by T. S. McCann, M. A. Fedak, and J. Harwood (1989) were used for this age class.
Recent studies in which females were chemically immobilized (Baker et al. 1990; McCann, Fedak, and Harwood 1989; Boyd, Arnbom, and Fedak 1993) have yielded a relationship between mass and nose-tail length for females (fig. 6.2). This was used to estimate mean mass for each age class of elephant seal (table 6.1). The relationship between mass and length in males described by Bryden (1969b , 1972) was used to calculate the equivalent mass-age relationship for males. The pectoral girth of seals was also estimated using the equations in M. M. Bryden (1969b ) which were subsequently corrected (Bryden 1972; see table 6.1).
Biomass
The number of individuals in each age class was calculated from a pup production of 102,000 (McCann and Rothery 1988), assuming a 1:1 sex ratio at birth (McCann 1985) and a stable population age structure. The biomass of each age class was derived from the product of the
number of individuals in each age class and the mean mass of those individuals (table 6.1). The total body gross energy (TBGE) for that biomass was calculated using the equation provided by J. J. Reilly and M. A. Fedak (1990) derived from body composition analyses of gray seals. We assumed a mean water content of 55% derived from estimates of northern elephant seal, M. angustirostris , water content during lactation and molt (Costa et al. 1986; Worthy et al. 1992). The validity of using the Reilly and Fedak equation on different species was discussed in relation to other work on ringed seals, Phoca hispida (Stirling and McEwan 1975), and was broadly confirmed by I. L. Boyd and C. D. Duck (1991). Estimates of biomass are calculated in metric tonnes; 1 tonne equals 1,000 kg.
Energy Expenditure
The annual cycle of southern elephant seals involves three distinct activities: (1) reproduction in adult seals, (2) molt, and (3) foraging at sea (Laws 1960). Each of these will have a characteristic energy cost depending on the mass, reproductive status, and sex of the seal. An additional cost will be incurred in terms of body growth, which will vary with age, although reversible growth, such as seasonal fattening before breeding or molt, is considered as one of the costs of reproduction or molt in this analysis. The total energy expenditure (E) for an individual southern elephant seal can be expressed as

where ER, EG, EF, and EM are the energy costs of reproduction, growth, foraging, and molt, respectively. ER includes the heat increments of lactation, gestation, and, in the case of males, harem defense and competition for mates. EF includes the heat increments of locomotion and feeding the estimates of assimilation efficiency and duration of foraging. EG includes the energy value of tissue growth but excludes seasonal changes in body reserves.
Energy of Reproduction
The metabolic rate, measured by metabolic water production, of female southern elephant seals during lactation was 88 MJ/day (Fedak et al., this volume) or 3.1 times the predicted standard metabolic rate (SMR). The general relationship (SMR = 0.293 W0.75 MJ/d) between SMR and body mass of mammals has been discussed and confirmed for seals by Lavigne et al. (1986). Energy expenditure on metabolism was 46% of total energy expenditure. This shows that the total energy expenditure of lactation is 6 times SMR, which is in close agreement with measurements made in northern elephant seals (Costa et al. 1986). The mean duration of suckling used to calculate the heat increment of lactation was 23 days for mothers with either male or female pups (McCann, Fedak, and
Harwood 1989; Fedak et al., this volume). Thus, the energy of reproduction for an individual female was obtained from 6 × SMR × 23.
The mean mass of elephant seals at birth was 42.5 kg (McCann, Fedak, and Harwood 1989). The energy density of fetal seals at term has been calculated for ringed seals (9.04 MJ/kg; Stirling and McEwan 1975), harp seals (7.24 MJ/kg; Worthy and Lavigne 1983) and gray seals (5.76 MJ/kg; Anderson and Fedak 1987). The variation in these figures probably reflects species differences in the fat content of pups at birth. We do not know which value is the most appropriate for southern elephant seals, but because some of the ringed seal pups in I. Stirling and E. H. McEwan's study (1975) could have been suckled and this figure may be an overestimate of energy density, we used a conservative value of 6.5 MJ/kg in this analysis. The mass of southern elephant seal placentas is 5 kg, and an energy density of 0.46 MJ/kg (Lavigne and Stewart 1979) was used to calculate total energy content.
There is less information about the energy costs of reproduction in males. The only pinniped studies that have measured energy expenditure showed that breeding male northern elephant seals and territorial male Antarctic fur seals, Arctocephalus gazella , both have metabolic rates 3.3 times SMR (Deutsch, Haley, and Le Boeuf 1990; Boyd and Duck 1991). The competitive mating behavior of these species is similar to the southern elephant seal (McCann 1981). Therefore, we used the same value to calculate the metabolic costs of reproduction in males > 6 years of age. According to McCann (1980), the number of bulls ashore at the breeding grounds increases from September 1 to October 1 and remains roughly stable thereafter until November 18 when the number declines rapidly. We have assumed that they are present from September 14 until November 20 (58 days). The duration of the postweaning fast for pups was 45 days.
Energy of Molt
The energy cost of molt in southern elephant seals was estimated to be 2.4 times SMR (Boyd, Arnbom, and Fedak 1993), which is similar to that measured for adult female northern elephant seals (Worthy et al. 1992). Molting lasts 28 and 40 days on average in adult female and male southern elephant seals, respectively (Ling and Bryden 1981). These values were used to calculate the heat increment of molt in both sexes.
Energy of Growth
The body composition of southern elephant seals shows minor changes at different stages of life (Bryden 1969a ). However, because the scale of these changes is small, we have assumed that growth of body components is proportional to growth in total mass. The annual growth increment of southern elephant seals was calculated from the data on body mass (table 6.1), and the energy increment it represents was calculated using the equation for TBGE provided by Reilly and Fedak (1990).
Energy of Foraging
Activity budgets of seals during the aquatic phases of the annual cycle are based on time depth recorder outputs for southern elephant seals (Boyd and Arnbom 1991; Slip, Hindell, and Burton, this volume). While at sea, adult elephant seals spend 88% of their time diving. Velocities of descent and ascent were 1.64 m/s and 1/44 m/s, which makes sense in terms of empirical measurements of the cost of transport in phocids (Davis, Williams, and Kooyman 1985; Williams and Kooyman 1985). Seventy-four percent of dives had a profile showing that the seals remained at a specific depth for 54% of the duration of the dive. A further 10% of dives had a period when the seals remained at a constant depth before the resumption of a descent phase, and 16% of dives had no period spent at constant depth. Mean dive duration was 17 minutes for each type of dive.
The work required to transport a body through seawater is given by

where CD is the coefficient of drag, V is the velocity, r is the average water density (1.027 × 103 kg/m3 for the Southern Ocean, data from British Antarctic Survey), A is the maximum cross-sectional area, and L is the distance. This is an extended form of the equation of drag (Williams 1987). Estimates of CD will depend on the shape and size of the seal. Values of CD measured for seals range from 0.06 to 0.09 (Williams and Kooyman 1985; Feldkamp 1987), but these are for gliding seals, and it is well known that the drag associated with active swimming is greater. Furthermore, an average adult female elephant seal (length 3.0 m) has a Reynolds number (Re) of 4.3 × 106 , which is close to Re = 5 × 106 for turbulent flow at a velocity of 1.5 m/s. This suggests that most elephant seals are close to the boundary of turbulent flow. Put together, this suggests that the CD for southern elephant seals is greater than measured values for other species would suggest. Therefore, a value of 0.12 was used in the calculation.
T. M. Williams, G. L. Kooyman, and D. A. Croll (1990) showed that harbor seals, Phoca vitulina , had a swimming efficiency (power output as a percentage of power input) that varied with velocity but was approximately 10% at 1.5 m/s.
Using the parameters given in table 6.1, we calculated the average cost of transport for the South Georgia elephant seal population. This assumed that most elephant seals forage in a way similar to the small sample for which detailed measurements are available. It also assumed that nonbreeders continued to forage during the breeding season but that all seals molted on land.
For harp seals, the heat increment of digestion was estimated to be 17% of the gross energy intake (Gallivan and Ronald 1981), and for harp seals and northern fur seals, the assimilation efficiency was estimated to be 90%
of gross energy intake (Keiver, Ronald, and Beamish 1984; Fadely, Worthy, and Costa 1990) on a diet of fish. There is no information for elephant seals, so we have used these figures in our calculations of the heat increment of foraging.
Diet Composition and Population Energy Consumption
It has been difficult to obtain an accurate measure of the diet of southern elephant seals at South Georgia mainly because it has not yet proved possible to measure diet at the foraging grounds. We have to rely on stomach samples obtained from elephant seals at the breeding and molting areas, which can be many hundreds of kilometers from the foraging areas (Fedak et al, this volume). Laws (1956) examined 139 stomachs, of which 108 contained no food; of the remainder, 24 contained squid and 9 fish. Laws (1977) therefore suggested that the diet of southern elephant seals consisted of 75% squid and 25% fish by weight. P. G. Rodhouse et al. (1992) have described the cephalopod prey, from samples obtained by stomach flushing, and confirmed that squid made up the greatest proportion of the diet. No fish remains were discovered, but fish are probably underrepresented in stomach flushes because of their rapid rate of digestion. Therefore, we have little justification for departing from Laws's overall figure for diet composition. In addition, we have to assume for the present that diet measured from stomach lavaging is broadly indicative of overall diet, although the analysis presented here also provides an indication of what amounts of different types of prey would be taken if the proportions of squid and fish departed from those suggested by Laws (1977).
Energy densities vary considerably between different species of squid, so the squid diet was divided into three parts: (1) muscular squid, (2) gelatinous squid, and (3) cranchiid squid, which have a leathery mantle. The classification of each species plus its representation by percentage mass of squid in the diet is given in table 6.2. Energy values were assigned following A. Clarke et al. (1985). We assumed that fish taken were a mixture of myctophids and notothenids. Although there is no evidence that southern elephant seals feed on myctophids, they are dominant among species of shoaling fish in the parts of the water column in which elephants seals apparently feed (Hindell 1991; Boyd and Arnbom 1991), which suggests that they are potential prey. Energy densities of myctophids are in the range of 7–8 MJ/kg (Cherel and Ridoux 1992), and we have used a value of 7.5 MJ/kg. The energy density of other fish species was assumed to be 4 MJ/kg (ibid.).
The total biomass of each item consumed in the diet was calculated from the gross energy requirement of the population (GE), so that

|
where I1m , I2m , . . . , lnm denote the mass of each item in the diet and I1e , I2e , . . . , lne denote the energy value (MJ/kg) of each item. This equation was solved for the mass of each item from knowledge of the proportion by mass of each item in the diet. Therefore,

where r1, r2, r3, and so on were the ratios of Inm to the mass of the other items in the diet.
Results
Annual Energy Budget
The pattern of energy demand by each age class reflected the changing number, mass, and reproductive condition with age (fig. 6.3). Total energy expenditure in both sexes declined initially, because of the high rate of juvenile mortality, and then began to increase. Among females, this was due to the introduction of the additional burden of reproduction, while among males, the increase was the result of growth and increased costs of locomotion associated with larger body size.
The energy expenditure of individual males increased to 55 × 103 MJ up to age 10 with a sharp increase in energy expenditure associated with the
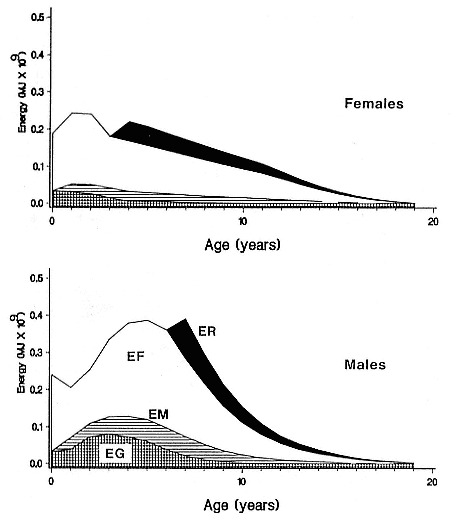
Fig. 6.3
Changes in the energy expenditure of different age classes of the
stable southern elephant seal stock at South Georgia.
beginning of reproduction at age 7 (fig. 6.4). In females, the asymptote of annual energy expenditure was reached at a similar age but at about one quarter of the energy expenditure of males.
The total population size was 469,000, with a sex ratio of 1.25 females for each male. The total biomass was 222,903 tonnes, of which male biomass made up 63% and the mean mass was 205 kg. The estimated total annual energy expenditure for the population was 6.01 × 109 MJ, which was equivalent to 190 Mw averaged over the whole year.
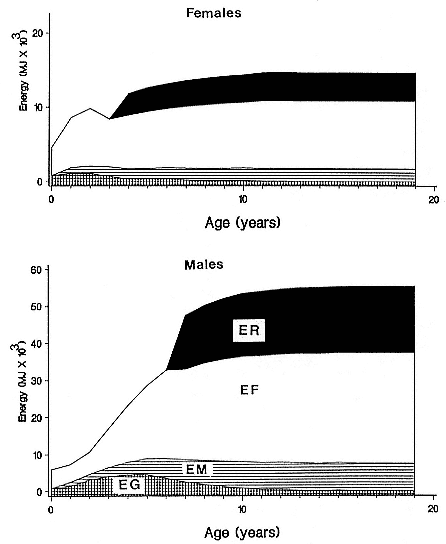
Fig. 6.4
Changes in the per capita energy expenditure of female and
male southern elephant seals in relation to age.
The annual energy expenditure associated with foraging (EF) was 63.2% and 68.2% of the total energy costs in males and females, respectively (fig. 6.5), and EF was the largest single form of energy expenditure. Overall, 65.2% of the energy expenditure of the population was spent on foraging. Among males, the energy expenditure of molt (EM) was the next largest energy demand (13.7%), and this was greater than the total energy
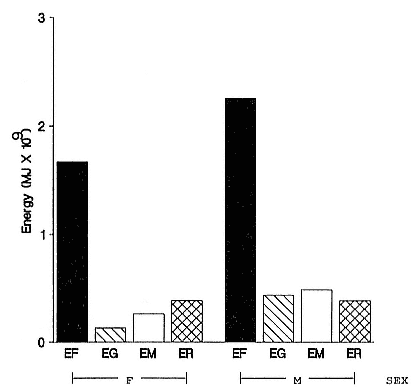
Fig. 6.5
Components of the annual energy budget of southern elephant seals from
South Georgia. EF = foraging energy expenditure; EG = energy expenditure
of growth; EM = energy expenditure of molt; ER = energy
expenditure of reproduction.
expenditure of molt in females (10.8% of female total). Energy expenditure on reproduction (ER) was similar in the two sexes (3.87 × 108 MJ for females and 3.88 × 108 MJ for males). This was 10.9% and 15.8% of the total energy expenditure for males and females, respectively. The energy expenditure on growth was 12.3% and 5.3% of the total expenditure for males and females, respectively.
Food Consumption
The total biomass of squid and fish (assuming 100% myctophids) consumed was 1.71 and 0.26 million metric tonnes per year, respectively (table 6.3). The female section of the population consumed 0.63 and 0.11 million tonnes of squid and fish, while equivalent values for males were 1.08 and 0.15 million tonnes, respectively. The biomass of each squid species consumed suggested that both males and females specialized in the large,
|
muscular species of high energy density (table 6.2) compared with the species of lower energy density (fig. 6.6).
Production Efficiency
The gross energy requirement (GE) of the population was 7.82 × 109 MJ (3.18 × 109 MJ for females and 4.64 × 109 MJ for males), and the production energy was 0.65 × 109 MJ. The production efficiency was therefore 8.2%. On average, gross energy intake during potential foraging time was 77.3 and 43.2 MJ/day for males and females, respectively. This suggests a capture rate of 0.26 and 0.15 kg of muscular squid or fish per dive for males and females, respectively, based on dive rates obtained from Boyd and Arnbom (1991) and D. Slip, M. Hindell, and H. Burton (this volume).
Discussion
It was not possible to provide statistical confidence intervals on estimates of energy expenditure because of the very variable quality of the data used to produce these estimates. Therefore, the results should be interpreted with care, although there is evidence supporting their accuracy. For example, our estimate of the gross energy requirements of the South Georgia elephant seal population compares well with the estimate for harp seals given by D. M. Lavigne et al. (1985). They estimated a per capita energy requirement of 11.3 × 103 MJ/year, while for the larger elephant seal, the value was 16.8 × 103 MJ/year. In addition, W. Sakamoto et al. (1989) esti-
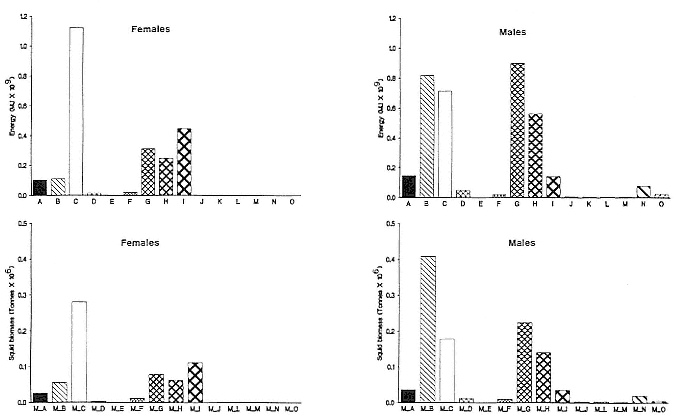
Fig. 6.6
Composition of the squid portion of the diet of southern elephant seals from the South Georgia stock
expressed in terms of energy value and biomass. The squid species are those labeled in table 6.2.
mated the daily gross energy requirement for an average adult female northern elephant seal when foraging as about 40 MJ/day. In this study we estimate a value of 41 MJ/day for the daily energy expenditure of adult females averaged over the whole year.
We have estimated population biomass to be 1.21 times that estimated by McCann (1985). This is because we calculated biomass at the end of the breeding season, whereas McCann did not include the biomass of the 0+ age class. His estimates of increasing mass with age were also more conservative, but our experience of weighing both male and female elephant seals has suggested that the estimates of mass given in table 6.1 are realistic. There are also significant differences between the food consumption of the population estimated in this study compared with the estimate made by McCann (1985). We found a total biomass consumption of 2.1 million tonnes (given the 3:1 numerical ratio of squid to fish in the diet), whereas McCann (1985) estimated this to be 3.68 million tonnes. The difference is largely caused by the figures used by McCann to translate population biomass into an estimate of food consumption. Following Laws (1977), he assumed that the annual food consumption was 20 times body mass. Our estimates suggest that for elephant seals the multiplier should be closer to 10. This is similar to the estimate made for harp seals on a fish diet by Lavigne et al. (1985).
A further reason for the reduced size of the multiplier is the apparently high production efficiency shown by southern elephant seals. W. F. Humphreys (1979) showed that production efficiency for mainly small herbivorous mammals rarely exceeded 5%. Respiratory costs are reduced in elephant seals because of the large body size (Lavigne et al. 1986) and because the cost of transport per unit of mass declines with increased mass. Since the cost of foraging, which is largely associated with the cost of transport, was the major form of energy expenditure, then the respiratory costs of a population of large swimming mammals will be lower than a population with an identical biomass but made up of a greater number of small individuals. Thus, the production efficiency of a harp seal population is about half that of the elephant seal. The long delay in reproductive maturity of males also contributes greatly to the production efficiency because energy normally expended on reproduction is available to be routed into growth.
Apart from the suggestion of Laws (1977), there is no evidence that elephant seals feed with a ratio of three parts squid to one part fish by weight. This highlights one of the greatest deficiencies in our knowledge of southern elephant seal foraging ecology. The diet may contain more or less fish, and it may also contain more benthic and demersal species that will have energy densities more similar to the muscular squid (4 MJ/kg, table 6.2, Cheral and Ridoux 1992) than to myctophids, which are particularly
oily. Table 6.3 compares the biomass of fish and squid consumed with different proportions of fish and squid in the diet. Estimates of total food consumed also depend on the energy values applied to each item of the diet. In this study these are only crude estimates that, in the case of squid, are based on estimates of the energy density of related temperate squid species (Clarke et al. 1985). Little is known about some of the species found in the diet of southern elephant seals, and we cannot be certain that the general classification into "muscular" or "gelatinous" forms is correct. Estimates of total consumption must also be interpreted with care because lavaging animals soon after they arrive on land probably only indicates diet in the immediate surroundings of the landing site or is biased by items, such as squid beaks, that persist in the stomach longer than other food remains.
One of the most significant potential threats to the stability of the South Georgia elephant seal stock is competition for food with commercial fisheries. This study is a synthesis of knowledge about the biology of southern elephant seals at South Georgia that provides an important, albeit imprecise, insight into the potential interactions between the South Georgia southern elephant seal population and its food supply. The metabolic costs of maintenance for fully grown adult female southern elephant seals was 0.39 MJ/kg.75 , and for adult males it was 0.17 MJ/kg.75 . The differences between the sexes is probably caused by differences in the foraging component of energy expenditure because the cost of locomotion increases in direct proportion to cross-sectional area. Large changes in mass are accompanied by relatively small changes in cross-sectional area, resulting in reduced costs of locomotion per unit mass. The total annual energy expenditure for the southern elephant seal population at South Georgia was 6.01 × 109 MJ/year, and 59% of this was accounted for by males. Despite contributing nothing to the energy costs of raising young and having only a small chance of reproducing (Deutsch, Haley, and Le Boeuf 1990), males demand a greater proportion of the total resources than females. They may also require richer foraging grounds because during potential periods of foraging, the rate of energy intake of males has to be almost twice that of females. These differences suggest that male survival, growth, and condition may be more responsive to changes in environmental conditions than that of females. However, the high production efficiency of southern elephant seals also suggests that they may be able to exploit highly dispersed prey resources.
Acknowledgments
This chapter is the result of a collaborative research project between the British Antarctic Survey (Natural Environment Research Council) and the Sea Mammal Research Unit (NERC). We thank T. Barton, C. Chambers, B. J. McConnell, A. Morton, and A. Taylor for assistance in the field.
References
Anderson, S. S., and M. A. Fedak. 1987. Gray seal, Halichoerus grypus , energetics: Females invest more in male offspring. Journal of Zoology, London 211: 667–679.
Arnbom, T. A., N. J. Lunn, I. L. Boyd, and T. Barton. 1992. Ageing live Antarctic fur seals and southern elephant seals. Marine Mammal Science 8: 37–43.
Baker, J. R., M. A. Fedak, S. S. Anderson, T. Arnbom, and J. R. Baker. 1990. Use of tiletamine-zolazepam mixture to immobilise wild gray seals and southern elephant seals. Veterinary Record 126: 75–77.
Boyd, I. L., and T. Arnbom. 1991. Diving behaviour in relation to water temperature in the southern elephant seal: Foraging implications. Polar Biology 11: 259–266.
Boyd, I. L., T. Arnbom, and M. A. Fedak. 1993. Water flux, body composition and metabolic rate during molt in female southern elephant seals (Mirounga leonina ). Physiology Zoology 66: 43–60.
Boyd, I. L., and C. D. Duck. 1991. Mass changes and metabolism in territorial male Antarctic fur seals (Arctocephalus gazella ). Physiological Zoology 64: 375–392.
Bryden, M. M. 1969a . Relative growth of the major body components of the southern elephant seal, Mirounga leonina (L.). Australian Journal of Zoology 17: 153–177.
———. 1969b . Growth of the southern elephant seal, Mirounga leonina (Linn.). Growth 33: 69–82.
———. 1972. Body size and composition of elephant seals (Mirounga leonina ): Absolute measurements and estimates from bone dimensions. Journal of Zoology, London 167: 265–276.
Cherel, Y., and V. Ridoux. 1992. Prey species and nutritive value of food fed during summer to king penguin, Aptenodytes patagonica , chicks at Possession Island, Crozet Archipelago. Ibis 134: 118–127.
Clarke, A., M. R. Clarke, L. J. Holmes, and T. D. Waters. 1985. Calorific values and elemental analysis of eleven species of oceanic squids (Mollusca: Cephalopoda). Journal of the Marine Biological Association 65: 983–986.
Costa, D. P., B. J. Le Boeuf, A. C. Huntley, and C. L. Ortiz. 1986. The energetics of lactation in the northern elephant seal, Mirounga angustirostris. Journal of Zoology, London 209: 21–33.
Davis, R. W., T. M. Williams, and G. L. Kooyman. 1985. Swimming metabolism of yearling and adult harbor seals, Phoca vitulina. Physiological Zoology 58: 590–596.
Deutsch, C. J., M. P. Haley, and B. J. Le Boeuf. 1990. Reproductive effort of male northern elephant seals: Estimates from mass loss. Canadian Journal of Zoology 68: 2580–2593.
Fadely, B. S., G. A. J. Worthy, and D. P. Costa. 1990. Assimilation efficiency of northern fur seals determined using dietary manganese. Journal of Wildlife Management 54: 246–251.
Fedak, M. A., S. S. Anderson, and J. Harwood. 1981. The energetics of the gray seal (Halichoerus grypus ) in European waters: Energy flow and management implications. Final Report to the European Communities on Contract ENV 405-80-UK (B).
Feldkamp, S. D. 1987. Swimming in the California sea lion: Morphometrics, drag, and energetics. Journal of Experimental Biology 131: 117–135.
Gallivan, G. J., and K. Ronald. 1981. Apparent specific dynamics action in the harp seal (Phoca groenlandica ). Comparative Biochemistry and Physiology 69A: 579–581.
Hindell, M. A. 1991. Some life-history parameters of a declining population of southern elephant seals, Mirounga leonina. Journal of Animal Ecology 60: 119–134.
Hindell, M. A., and H. Burton. 1987. Past and present status of the southern elephant seal (Mirounga leonina ) at Macquarie Island. Journal of Zoology, London 213: 365–380.
Humphreys, W. F. 1979. Production and respiration in animal populations. Journal of Animal Ecology 48: 427–453.
Keiver, K. M., K. Ronald, and F. W. H. Beamish. 1984. Metabolizable energy requirements for maintenance and faecal and urinary losses of juvenile harp seals (Phoca groenlandica ). Canadian Journal of Zoology 62: 769–776.
Lavigne, D. M., S. Innes, R. E. A. Stewart, and G. A. J. Worthy. 1985. An annual energy budget for northwest Atlantic harp seals. In Marine Mammals and Fisheries ed. J. R. Beddington, R. J. H. Beverton, and D. M. Lavigne, 319–336. London: George Allen and Unwin.
Lavigne, D. M., S. Innes, G. A. J. Worthy, K. M. Kovacs, O. J. Schmitz, and J. P. Hickie. 1986. Metabolic rates of seals and whales. Canadian Journal of Zoology 64: 279–284.
Lavigne, D. M., and R. E. A. Stewart. 1979. Energy content of harp seal placentas. Journal of Mammalogy 60: 854–856.
Laws, R. M. 1953. The elephant seal (Mirounga leonina Linn.). I. Growth and age. Falkland Islands Dependencies Survey, Scientific Reports 8: 1–62.
———. 1956. The elephant seal (Mirounga leonina Linn.). I. General, social and reproductive behavior. Falkland Islands Dependencies Survey, Scientific Reports 13: 1–88.
———. 1960. The elephant seal (Mirounga leonina Linn.) at South Georgia. Norsk Hvalfangst-Tidende 49: 466–476, 520–542.
———. 1977. Seals and whales of the Southern Ocean. Philosophical Transactions of the Royal Society of London, Series B , 279: 81–96.
Ling, J. K., and M. M. Bryden. 1981. Southern elephant seal, Mirounga leonina Linnaeus, 1758. In Handbook of Marine Mammals . 2. Seals , ed. S. H. Ridgway and R. J. Harrison, 297–327. London: Academic Press.
McCann, T. S. 1980. Population structure and social organization of southern elephant seals, Mirounga leonina (L.). Biological Journal of the Linnaean Society 14: 133–150.
———. 1981. Aggression and sexual activity of male southern elephant seals, Mirounga leonina. Journal of Zoology, London 195: 295–310.
———. 1985. Size, status and demography of southern elephant seal (Mirounga leonina ) populations. In Sea Mammals in South Latitudes: Proceedings of a Symposium of the 52d ANZAAS Congress in Sydney—May 1982 , ed. J. K. Ling and M. M. Bryden, 1–17. Northfield: South Australian Museum.
McCann, T. S., M. A. Fedak, and J. Harwood. 1989. Parental investment in southern elephant seals, Mirounga leonina. Behavioral Ecology and Sociobiology 25: 81–87.
McCann, T. S., and P. Rothery. 1988. Population size and status of the southern elephant seal (Mirounga leonina ) at South Georgia, 1951–1985. Polar Biology 8: 305–309.
Reilly, J. J., and M. A. Fedak. 1990. Measurement of the body composition of living gray seals by hydrogen isotope dilution. Journal of Applied Physiology 69: 885–891.
Rodhouse, P. G., T. A. Arnbom, M. A. Fedak, J. Yeatman, and A. W. A. Murray. 1992. Cephalopod prey of the southern elephant seal Mirounga leonina L. Canadian Journal of Zoology 70: 1007–1015.
Sakamoto, W., Y. Naito, A. C. Huntley, and B. J. Le Boeuf. 1989. Daily gross energy requirements of female northern elephant seal, Mirounga angustirostris , at sea. Nippon Suisan Gakkaishi 55: 2057–2063.
Skinner, J. D., and R. J. van Aarde. 1983. Observations on the trend of the population of southern elephant seals, Mirounga leonina , at Marion Island. Journal of Applied Ecology 20: 707–712.
Stirling, I., and E. H. McEwan. 1975. The caloric value of whole ringed seals (Phoca hispida ) in relation to polar bear (Ursus maritimus ) ecology and hunting behavior. Canadian Journal of Zoology 53: 1021–1027.
van Aarde, R. J. 1980. Fluctuations in the population of southern elephant seals, Mirounga leonina , at Kerguelen Island. South African Journal of Zoology 15: 99–106.
Williams, T. M. 1987. Approaches to the study of exercise physiology and hydrodynamics in marine mammals. In Approaches to Marine Mammal Energetics , ed. A. C. Huntley, D. P. Costa, G. A. J. Worthy, and M. A. Castellini, 127–146. Special Publication no. 1. Lawrence, Kan.: Society for Marine Mammalogy.
Williams, T. M., and G. L. Kooyman. 1985. Swimming performance and hydrodynamic characteristics of harbor seals, Phoca vitulina. Physiological Zoology 58: 576–589.
Williams, T. M., G. L. Kooyman, and D. A. Croll. 1990. The effect of submergence on heart rate and oxygen consumption of swimming seals and sea lions. Journal of Comparative Physiology 160B: 637–644.
Worthy, G. A. J., and D. M. Lavigne. 1983. Changes in the energy stores during postnatal development of the harp seal, Phoca groenlandica. Journal of Mammalogy 64: 89–96.
Worthy, G. A. J., P. A. Morris, D. P. Costa, and B. J. Le Boeuf. 1992. Molt energetics of the northern elephant seal. Journal of Zoology, London 227: 257–265.