15—
UNIQUE ECOLOGICAL AND MANAGEMENT PROBLEMS OF CALIFORNIA DESERT RIPARIAN SYSTEMS
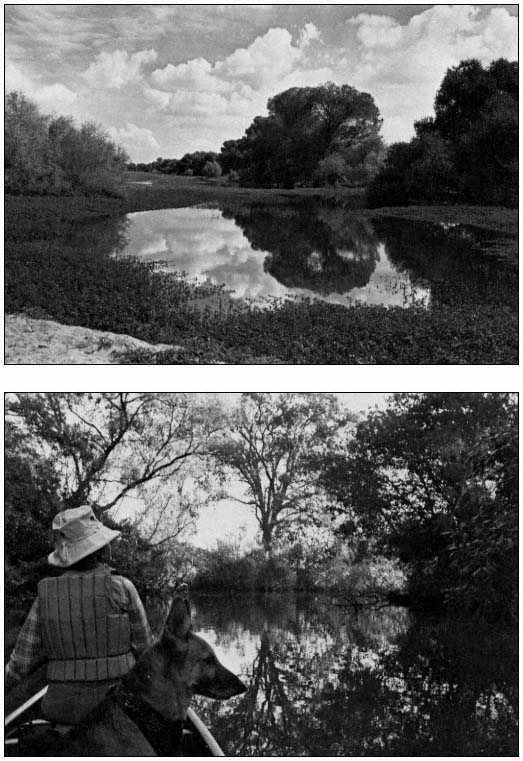
Temporal Desert Riparian Systems—the Mojave River as an Example[1]
Louis A. Courtois[2]
Abstract.—During years of high precipitation, temporal riparian zones form on the dry lake playas within the Mojave River drainage and can exist for several years. This is followed by establishment of pioneer aquatic species which eventually give way to halophytes as surface waters recede. Once surface waters evaporate the alkali sink vegetation becomes reestablished.
Introduction
Riparian systems are usually associated with permanent water such as lotic (rivers, streams or springs) or lentic (lakes, ponds or seeps) habitats. Riparian vegetation may also be found in areas of intermittent flow such as arroyos. In each situation, riparian systems occupy the moist transition (riparian) zones between the wet aquatic and the dry upland zones (Thomas etal . 1979). Water is the major limiting factor upon which riparian vegetation is totally dependent.
A temporal desert riparian system is also dependent upon water and only becomes established during years of high precipitation. Flash floods, which result from high precipitation, carry water through the drainage until it ultimately reaches dry lake playas (e.g., flat floored bottom of an undrained basin) where it becomes impounded. Inundation of these alkali playas kills the existing plants and provides the necessary water source for establishment of a temporal riparian system. The word temporal best defines this system because, as the surface waters evaporate, the riparian zone slowly advances down into the basin, continually establishing itself along the dry shoreline. Immediately above the riparian zone the drier upland zone allows the alkali sink plant community to become reestablished.
The extent of a temporal desert riparian system is dependent upon the amount of seasonal runoff reaching the old lake basin. The temporal system, like a vernal pool, is filled by seasonal runoff. The vernal pool, however, is a much shorter-lived system, lasting several weeks to a few months. The temporal desert riparian system may last much longer, several months to a few years. The physical characteristics of the soil in these systems are also similar—both are common to older soils which have either a dense claypan or hardpan some depth below the surface. This permits an aquatic habitat to become established, since water cannot seep away into the lower soil column (Holland and Griggs 1976). Vernal pools in California form only during the spring, but temporal riparian systems can form during the winter, spring, or as a result of summer storms.
The Mojave River drainage has both lotic and lentic riparian zones, as well as temporal riparian systems. To better understand these temporal riparian systems we must first understand the drainage characteristics which allow them to form and perpetuate.
Historic Drainage
During the Pleistocene a major portion of the Mojave River drainage consisted of three large lakes, Little Lake Mojave, Lake Mojave, and Lake Manix, which eventually drained into the Colorado River (fig. 1). Buwalda (1914) was one of the first to study Lake Manix and based its pre-existence upon lacustrine deposits, fossils, and ancient shorelines. The outflow from Lake Manix eventually carved out Afton Canyon, which flowed into Little Lake Mojave and Lake Mojave. Little Lake Mojave, the smallest of the three lakes, was intermittent and relatively short-lived. This lake included what today is known as East and West Cronese lakes (fig. 2). Lake Mojave was extensive, covering the present day playas of Soda and Silver lakes (fig. 2). Thompson (1921, 1929) studied the wave-cut cliffs, terraces, beach ridges, sand bars, and lacustrine deposits of Lake Mojave. He also found an "unmistakable outlet channel" which
[1] Paper presented at the California Riparian Systems Conference. [University of California, Davis, September 17–19, 1981].
[2] Louis A. Courtois is Associate Fishery Biologist for California Department of Fish and Game, Rancho Cordova, California.
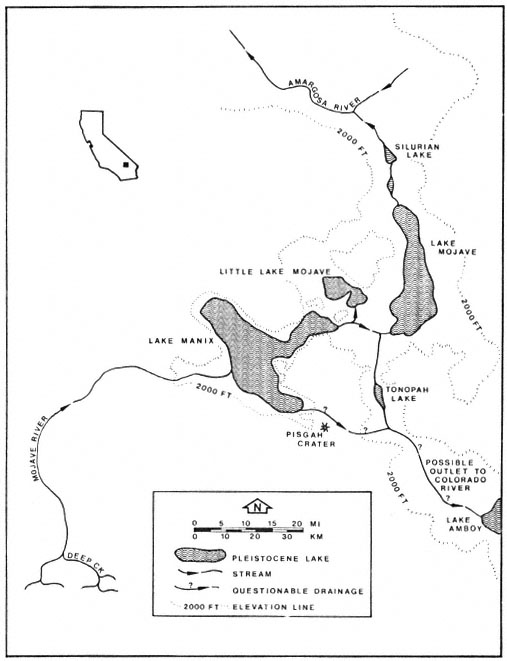
Figure 1.
Historic lakes present within the Mojave River drainage.
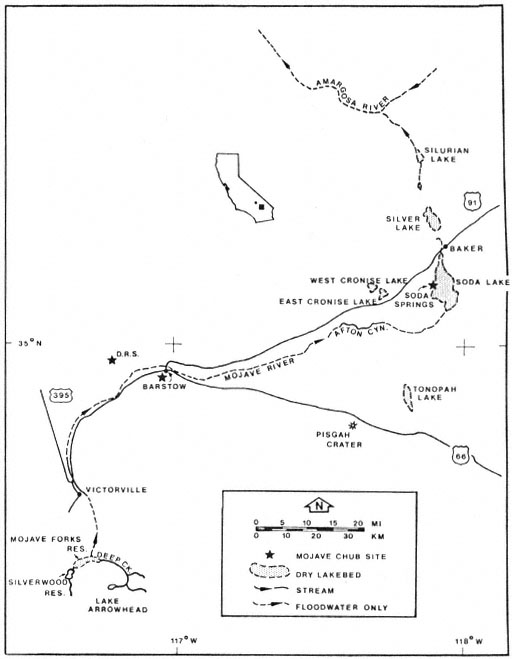
Figure 2.
Present features of the Mojave River drainage.
suggested the Mojave River at one time flowed northward meeting the Amargosa River.
As the climate became more arid, the lakes receded, and a more riverine habitat became established (Hubbs and Miller 1943). Today the Mojave River is an intermittent system of surface and subsurface flows draining into several inland basins. The basin which is the subject of this paper is East Cronese Lake, San Bernardino County, California (fig. 3). Periodic visits were made to this site during 1980–81 to determine what fish species occupied the impounded water. The basin flooded in 1978 following approximately 10 years of desiccation. The narrative which follows describes existing physical characteristics of the drainage and the gradual change in the structure of the plant community following inundation.
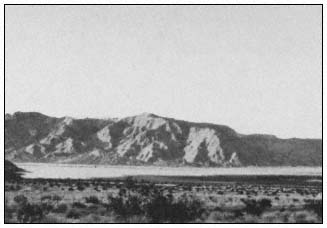
Figure 3.
East Cronese Lake basin filled with water (April 1981).
Present Drainage
The Mojave River drainage is fed by two headwater streams which originate in the San Bernardino Mountains (fig. 2): the west fork of the Moiave River, which drains 182.1 km2 (70.3 mi2 ); and Deep Creek, which drains 352 km2 (136 mi2 ). Flows from the two tributaries combine at an area known as "the forks" approximately 9.6 km. (6 mi.) southeast of Hesperia (fig. 2). From there the river flows underground to Victorville where a granite shelf forces it to the surface. Flows at Victorville and in Afton Canyon are nearly permanent and, at a minimum, supply standing pools of water during low-flow years. Afton Canyon (fig. 4) has a permanent riparian community modified only by periodic floods which scour and rearrange the
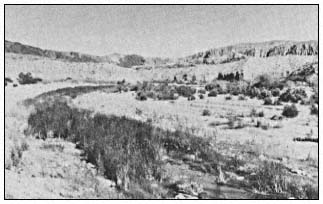
Figure 4.
Afton Canyon has a permanent riparian biotic community.
streamcourse. During periods of high runoff, water flows from Afton Canyon into East and West Cronese lakes and also into Soda and Silver lakes which are the termini for the drainage. When flooded, the surface area of East Cronese Lake is estimated at 517 ha. (analysis of dry lake contours on Cave Mountain, California, 1:62,500 USDI Geological Survey topographic map). Historic records indicate that both Silver Lake and the Cronese Lake basin have previously flooded (1916) to depths of over 3 m. (10 ft.) (Blanc and Cleveland 1961).
Rainfall and Flow Patterns
Annual rainfall in the Mojave River drainage varies from 876.1 mm. (34.5 in.) at Deep Creek to 145 mm. (5.7 in.) at Victorville and less than 101 mm. (4 in.) for Cronese Valley (California Department of Water Resources 1964). Flow records which go back to 1930 recorded runoff at Victorville varying between 16,600 acre-feet (AF) (1975) and 290,000 AF (1969) per year (USDI Geological Survey 1930–1979). Runoff at Barstow was 0.3 AF and 146,000 AF per year for those years.
Analysis of surfacewater flow records (ibid .) reveals some interesting factors about the lower Mojave River drainage. During wet years the bulk of the runoff passes through the system over a period of a few days to several weeks (table 1). Victorville and Afton Canyon both had base surface flows recorded every year, but Barstow had no appreciable flow during most years. The system appears to fit an all-or-none flow pattern for Barstow—when seasonal runoff exceeds 150,000 AF at Victorville, the surface flow is continuous from Victorville past Barstow through Afton Canyon and into the Cronese Lake basin. The Cronese Lake basin has flooded at least five times in the past 65 years: 1916, 1922, 1938, 1969, and 1978 (California Department of Water Resources 1964; USDI Geological Survey
|
1979). Besides these periodic floods, the only other surface flow to the Cronese basin is runoff from the surrounding mountains.
Elapsed time for peak flows to move from Victorville to Barstow range between seven (1969) and 23 hours. Corresponding times from Barstow to Afton Canyon range between six (1969) and 24 hours. Elapsed times appear to depend upon the amount of runoff moving through the system at that particular time.
Floral Associations
Establishment of a distinct riparian plant community following the filling of the lake playa is a progressive process. The gentle slope of the lake basin provides a shallow littoral zone which, when warmed by the sun, allows the planktonic community to bloom. Green filamentous algae are common to this zone. As the impounded waters slowly evaporate, the water surface recedes, leaving filamentous algal mats along the shoreline. East Cronese Lake had a large amount of filamentous algae hanging from the mesquite (Prosopis sp.) trees. These trees had been originally covered by water in 1978. The algal mats were approximately 1.8 m. (6 ft.) above ground level when observed in early 1981 (fig. 5). These mats appeared to form a continuous ring around the lakebed. Where there were no trees, algae was deposited on the playa, possibly providing a nutrient source for the subsequent plant community. The remains of smaller desert shrubs (creosote bush, sage, and desert holly) were evident on the damp soils near the lake surface. Small mounds of alkali sand covered by leaf litter at the base of these plant skeletons were all that remained (fig. 6).

Figure 5.
Dry algal mats hanging in dead mesquite on the lake playa.
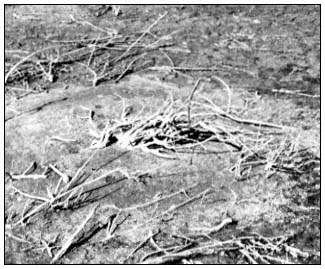
Figure 6.
Skeleton of desert shrub on the lake playa.
Zones of riparian growth were evident around the basin, each in a concentric pattern equidistant from the evaporating water surface (fig. 7). Later that summer (1981) many plant seedlings had grown sufficiently to produce a lush meadow-like area in the desert (fig. 8). Salt cedar (Tamarix sp.), heliotrope (Heliotropiumcurassavicum ) (fig. 9) and sea-purslane (Sesuviumverrucosum ) (fig. 10) were the dominant species. These plants commonly grow in alkali sinks but are apparently limited by water availability, especially the heliotrope.
Once the surface water is gone, the vegetation slowly reverts to the pre-flood alkali sink community (Brown 1968).
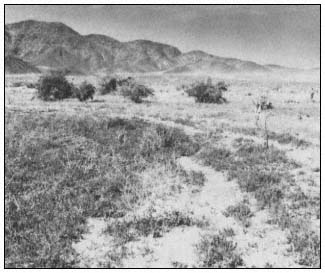
Figure 7.
Concentric growth zones within the lake
basin appear as surface waters recede.
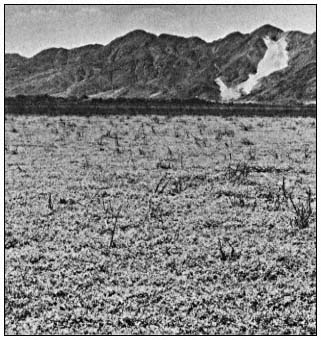
Figure 8.
The lake playa resembles a lush meadow following
establishment of a temporal riparian system.
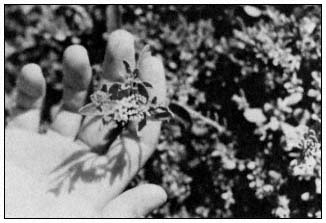
Figure 9.
Heliotrope (Heliotropium curassavicum ).
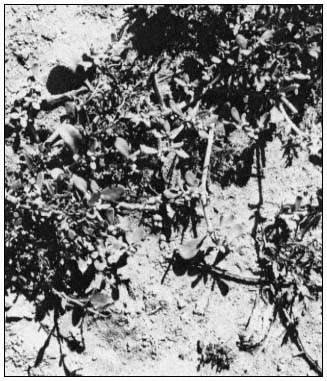
Figure 10.
Sea-purslane (Sesuvium verrucosum ).
Faunal Associations
There was much evidence of wildlife use of this area—wild horses and cattle were observed grazing on surface vegetation. Bird life, including Brown Pelican (Pelecanusoccidentalis ), Western Bluebird (Sialiamexicana ), Dark-eyed Junco (Juncohyemalis ), Common Crow (Corvusbrachyrhynchos ) and Robin (Turdusmigratorius ), utilized the trees, shoreline, and lake for feeding and nesting. There were also signs of coyote (Canislatrans ) in the area. The only amphibians noted were the Pacific tree frog (Hyla regilla ) and the bullfrog (Rana catesbeiana ). They were observed either living in the cracks of the drying lake surface or desiccated on the dry lake playa. Black bullhead (Ictalurusmelas ), fathead minnow (Pimephalespromelas ), green sunfish (Lepomiscyanellus ), mosquitofish (Gambusia affinis ), and arroyo chub (Gilaorcutti ), washed into the basin from upstream sources, were collected from surface waters.
The temporal desert riparian system provides an area where impounded fish species rapidly grow, reproduce, and eventually die once surface waters evaporate. The author observed numerous skulls and skeletons of Ictalurusmelas and Lepomiscyanellus along the shoreline. Historical records (Thompson 1929) indicate this to be a common cycle—windrows of fish "mummies" being found around the playa once the lake surface totally desiccates. Their loss provides additional nutrient input to the soils.
The Future
The temporal desert riparian system has some interesting physical and biological features which have been briefly examined in this paper. More study of this type of system is needed to provide a clearer understanding of the interrelationships among the various components. The ultimate fate of this system depends upon an awareness of its unique properties and how they influence the desert community.
Future management of the drainage will most certainly include increased demands for water. Already three flow-regulating dams have been con-
structed within the drainage: Lake Arrowhead Dam in 1923; Mojave Forks Dam in 1970; and Cedar Springs Dam in early 1972. Each of these structures was build to provide for increased waterrelated activities such as recreation, flood control, groundwater recharge, and irrigation. Construction of a diversion structure in Afton Canyon by the railroad during the mid-1900s has also modified historic flow patterns. This structure diverts most of the high-water flow into the Cronese Lake basin except during extremes in runoff when water reaches the Soda Lake basin.
Should agricultural development occur in areas downstream from Afton Canyon, the existing ecological balance of the temporal system would be upset. Certainly any permanent agricultural runoff into the Cronese Lake system would provide conditions for establishment of a permanent riparian community. Currently the spread of the exotic salt cedar into the basin has been limited. A more permanent water source, whether surface flow or unbound subsurface water, could provide conditions for the expansion of salt cedar as well as other plants common to permanent riparian communities. One consequence of such vegetation expansion would be the loss of the temporal system. The impact of this loss cannot be fully understood at the present time because of limited biological information. More intensive field surveys and biological studies of this system need to be completed before further modifications occur.
Literature Cited
Blanc, R.P., and G.B. Cleveland. 1961. Pleistocene lakes of southeastern California. California Division of Mines, Mineral Information Service. Vol. 14(4): 1–8.
Brown, G.W., Jr. (ed.). 1968. Desert biology: Special topics on the physical and biological aspects of arid regions. Volume 1. 635 p. Academic Press, New York, N.Y.
Bulwalda, J.P. 1914. Pleistocene beds at Manix in the eastern Mojave Desert region. University of Calfornia, Department of Geology Bull. 7: 443–464.
California Department of Water Resources. 1964. Ground water occurence and quality, Lahontan Region. Bull. 106–1.
Holland, R.F., and F.T. Griggs. 1976. A unique habitat—California's vernal pools. Fremontia 4(3): 3–6.
Hubbs, C.L., and R.R. Miller. 1943. Mass hybridization between two genera of cyprinid fishes in the Mohave Desert, California. Pap. Mich. Acad. Sci., Arts and Letters 28(1942): 343–378.
Thomas, J.W., C. Maser and J.E. Rodiek. 1979. Wildlife habitats in managed rangelands—the great basin of southeastern Oregon. Riparian zones. USDA Forest Service GTR-PNW-80, Portland, Ore. 18 p.
Thompson, D.G. 1921. Pleistocene lakes along Mohave River, California. J. Wash. Acad. Sci. 11(17): 423–424.
Thompson, D.G. 1929. The Mohave Desert region California: a geographic, geologic, and hydrologic reconnaissance. USDI Geological Survey Wat. Sup. Pap. 578.
USDI Geological Survey. 1930–1979. Water resources data for California. Water years 1930–1978. Vol. 1: the Great Basin.
Composition and Abundance of Bird Populations in Riparian Systems of the California Deserts[1]
A. Sidney England, Larry D. Foreman, and William F. Laudenslayer, Jr.[2]
Abstract.—Avian population diversity, density, and species richness in desert riparian systems were analyzed using 73 breeding bird surveys, 62 winter bird-population studies, and biweekly surveys at 15 sites. Breeding bird surveys indicated that cottonwood/willow vegetation-types had the highest number of breeding and visiting species and the highest bird diversity among desert vegetation-types. Willow had the highest bird density. Winter bird-population studies showed that cottonwood/willow also had the highest species richness and diversity during winter, but palm and palo verde/ironwood had higher bird densities. All population variables were higher in desert riparian systems than in non-riparian desert vegetation-types during winter and breeding seasons. Results from desert riparian systems were compared with 27 breeding bird surveys and 18 winter population studies in cismontane California riparian systems. Avian density, diversity, and species richness generally were greater in cismontane riparian systems. A small number of resident ground granivorous bird species were found to comprise a large proportion of all individuals using desert riparian systems especially during summer. Biweekly surveys showed species richness varied considerably during the year; the 15 sites surveyed fell into four general patterns. Ecological factors such as size of riparian zone, presence of overstory and shrub vegetation, availability of water, visibility, and geographic location may affect total and breeding species numbers. Results are discussed with respect to ecological factors and land management implications.
Introduction
In 1976, the United States Congress charged the USDI Bureau of Land Management (BLM) with responsibility for preparing a comprehensive management plan for public lands in the deserts of California. As part of this effort, the California Desert Plan Program conducted extensive inventories and studies of many resources and uses found in the planning area. The wildlife staff began the inventory by compiling existing data including museum records, checklists, unpublished field notes and BLM sight records, and published articles. It was soon realized that little quantitative information existed on birds in the California deserts. Most publications prior to 1970 were primarily annotated species accounts confined to specific areas (e.g., Clark Mountain [Miller 1940]; Providence Mountains [Johnson etal . 1948]; Joshua Tree National Monument [Miller and Stebbins 1964]) and did not cover many of the widely distributed vegetationtypes. Data collected by bird watchers were also restricted to a few sites (e.g., Morongo Valley, Furnace Creek Ranch) during selected seasons, and frequently only unusual species were recorded.
A twofold inventory design was used to collect basic descriptive data on the distribution, abundance, and seasonal variation of birds in California desert vegetation-types. First, per-
[1] Paper presented at the California Riparian Systems Conference. [University of California, Davis, September 17–19, 1981].
[2] A. Sidney England is Wildlife Biologist, Larry D. Foreman is District Wildlife Biologist; both are with the Bureau of Land Management, California Desert District, Riverside, Calif. William F. Laudenslayer, Jr. is Regional Wildlife Ecologist, USDA Forest Service, Tahoe National Forest, Nevada City, Calif.
manent study plots were established throughout the deserts and were censused during the breeding and winter seasons using standard spot-mapping techniques. The advantages of this method included compatibility with similar surveys conducted by others, relative repeatability, and accessibility to results by others through publication in "American Birds."
The second approach was a yearlong survey of selected desert riparian sites. This concentrated effort was warranted by the rarity of these sites in the California deserts, general knowledge of the importance of riparian systems to birds and other desert wildlife, and management concern arising from competing land uses. This year-round approach at specific sites allowed investigation of temporal changes in species richness and abundance not practical with spotmapping techniques. It also permitted comparison of several vegetation-types of various sizes including very small oases which are typical of most desert riparian areas but are seldom addressed in riparian studies.
This paper presents inventory findings relevant to avian use of desert riparian systems. The following specific questions are discussed.
1. How do abundance and species richness vary by season and between desert riparian vegetation-types?
2. How important are desert riparian systems relative to non-riparian desert sites?
3. How do avian use patterns in desert riparian systems compare to those of cismontane California riparian systems?
4. What factors affect species richness in desert riparian systems?
Answers to questions such as these are needed for management of riparian systems in the California deserts.
Methods
Study Area
For the purposes of this analysis, California deserts include all of the Mojave and Colorado deserts within California and that portion of the Great Basin Desert within California east of the White and Inyo mountains. Riparian systems along the Colorado River and the perimeter of the Salton Sea are excluded.
Breeding and Winter Bird Surveys
Quantitative data on breeding and winter birds were collected using the spot-mapping techniques prescribed by the National Audubon Society for breeding bird censuses (Svensson 1970; Van Velzen 1972a) and winter bird-population studies (Kolb 1965). From 1976 through 1978, BLM employees and contractors conducted 62 winter birdpopulation studies and 73 breeding bird censuses in a wide variety of desert sites. Findings from these surveys were combined with other published studies using standardized National Audubon Society techniques in California desert and cismontane California riparian systems.
Avian diversity, population density, and species richness values were calculated for each survey. Diversity was computed using the Shannon-Wiener Index (Shannon and Weaver 1949):
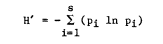
For comparisons among desert riparian vegetation-types, between riparian and non-riparian desert vegetation-types, and between desert and cismontane riparian vegetation-types, surveys were grouped into the following 11 vegetation-types based on the visually dominant plant(s) occurring on each study plot.
|
Average number of breeding and wintering species, average density, and average species diversity were computed for each vegetation-type. Breeding bird density and diversity values used only breeding males. Average number of species visiting census plots during the breeding season also was calculated; these data were not available for all censuses. Overall density, diversity, and species richness were also averaged for all desert vegetation-types, all "perennial" desert riparian systems, all "ephemeral" desert riparian systems, and cismontane riparian systems. For the purposes of this paper, the continuum of riparian system types present in the California deserts has been divided into two types: 1) "perennial"; and 2) "ephemeral". "Perennial" riparian systems typically have water at or near the surface throughout the year (e.g., springs, seeps, streams, etc.). "Ephemeral" riparian systems occur in washes where surface water typically is available for relatively short periods following storms.
Biweekly Surveys
Data on avian temporal and habitat use patterns were collected at 15 locations selected to represent the wide range of perennial riparian vegetation-types and sizes found in the California deserts (table 1). Study sites were established at each location; sites ranged in size from 0.2 ha. to 36.4 ha. One feature common to all sites was year-round presence of surface water. Censuses were conducted by systematically walking through each site recording all birds detected; individuals seen in riparian vegetation were recorded separately from individuals in adjacent non-riparian vegetation. Two plots were visited per day, one during the three-hour period following dawn and one during the three-hour period preceding sunset. Length of visit varied with the size of study site and the number of birds found. Each study site was visited approximately biweekly during the following periods: 6 November 1977 to 28 January 1978; 9 April 1978 to 17 June 1978; and 16 July 1978 to 3 January 1979.
|
Biweekly survey results and literature review were used to assign each species at each site to one or more of eight seasonal status designations. Year-round, summer, and winter residents described species found throughout these time periods. Spring and fall migrants passed through the sites relatively quickly. Summer and winter transients included species that moved through the site, but unlike migrants, remained for extended periods on the way to or from breeding areas (e.g., Great Blue Heron), and species that occurred widely in the desert but were irregular in riparian systems (e.g., Redtailed Hawk, Roadrunner). Vagrants differed from migrants because they were well outside their normal distributions (e.g., Brown Thrasher, Rosebreasted Grosbeak).
Breeding status at a study site was considered "confirmed" if nesting activity was observed or recently fledged young of species not found in the adjacent desert were seen. Breeding was considered "probable" if the species was known from literature or from other study sites to breed in desert riparian systems and was present at the site throughout the breeding season.
Total number of species observed at each site on all visits (species richness) and total number of breeding species (confirmed plus probable) at each site were plotted against study site size. Sites with shrubs only, shrubs with tree overstory, and shrubs with tree overstory and ponds were examined separately to determine the effects of vegetation structure on species richness and number of breeding species.
Temporal species richness patterns were analyzed by plotting number of species observed per visit at each site against time. If more than one visit occurred at a site in a single biweekly sampling period, the average number of species observed was used. Species richness:time curves for each site were inspected visually and combined into groups with similar patterns. Characteristic curves for each group were constructed by averaging the total number of species observed in each sampling period.
Total number of individuals counted on each visit to each site was plotted against time to show temporal abundance patterns. If multiple visits occurred at a site in one sampling period, the average number of individuals counted was used. Abundance curves were inspected visually to determine gross abundance patterns. Common bird names are those found in the "Checklist of North American Birds" (American Ornithologists' Union 1957), as amended by supplements (1973, 1976).
Results
Breeding and Winter Bird Surveys
Distribution of surveys among vegetation-types and results of the analysis are presented in tables 2 (breeding birds) and 3 (winter birds). Ninety-nine breeding bird censuses and 80 winter bird-population studies were compiled for use in this analysis. Of these 179 surveys, 134 were in California desert (45 riparian and 89 nonriparian) and 45 were in cismontane California.
Among desert riparian vegetation-types, cottonwood/willow, willow, and palo verde/ironwood supported the highest numbers and greatest densities of breeding species. Diversity of breeding species also was highest in these vegetation-types. Cottonwood/willow supported approximately two to five times more breeding species than any other. The number of visiting species counted during breeding bird censuses was higher in perennial than in ephemeral desert riparian systems.
Results from winter bird-population studies indicated that cottonwood/willow, palm, and palo verde/ironwood supported more species than other desert riparian vegetation-types. Winter densities were highest in willow, palm, and palo verde/ironwood. With the exception of visiting species during the breeding bird censuses, all values for palo verde/ironwood, an ephemeral desert riparian vegetation-type, fell within the ranges of values for vegetation-types within perennial riparian systems. Comparisons between catclaw and other vegetation-types were complicated by the lack of replicate samples.
Comparing desert riparian and non-riparian systems data from tables 2 and 3 showed that density, diversity, and species richness of wintering birds were higher in desert riparian systems. Breeding bird densities were higher in all desert riparian systems than in non-riparian areas. The number of breeding species in desert woodland was comparable to that in willow and palo verde/ironwood and exceeded that in palm and mesquite. Desert scrub with overstory supported approximately the same number of breeding species as mesquite; palm supported a smaller number. Breeding species richness was higher in all desert riparian systems than in desert scrub. Only desert woodland was comparable to desert riparian vegetation-types in number of visiting species during the breeding season.
Cismontane riparian vegetation-types generally supported greater numbers of species as well as greater densities than desert riparian vegetation-types (table 2 and 3); diversity values were greater in cismontane vegetation-types during both seasons. Breeding bird surveys indicated only desert cottonwood/willow supported breeding species numbers comparable to cismontane riparian vegetation-types. Only in desert cottonwood/willow and willow were breeding densities similar to
|
cismontane riparian vegetation-types. However, the numbers of visiting species were greater in nearly all desert riparian vegetation-types than in cismontane riparian vegetation-types. Cismontane cottonwood/willow exceeded all desert riparian vegetation-types in species richness and density in winter; sycamore/live oak was comparable to several desert riparian vegetation-types.
Biweekly Surveys
A grand total of 190 bird species was observed at all sites on all visits combined. The total number seen at each site ranged from 41 at Brewery Spring to 122 at Old Woman Springs (table 4). Considerably more species were seen at the two large, man-created sites (Old Woman Springs and Iron Mountain Pumping Station) than at any other site. More species were recorded at sites supporting shrub vegetation with tree overstory than at sites with shrub vegetation only (fig. 1). The total number of species observed at sites having shrub vegetation with tree overstory increased with plot size (fig. 1); sites with ponds were included in this regression after excluding species dependent on open water. An analysis using average number of species per visit in place of total number of species produced similar results.
The number of confirmed breeding species ranged from zero at Dove and Cottonwood springs to nine at Old Woman Springs and Darwin Falls (table 4). Total number of breeding species (total breeding species), which included both confirmed and probable, ranged from two at Cottonwood Spring to 17 at Old Woman Springs. Sites having shrub vegetation with tree overstory generally supported more total breeding species than sites with shrub vegetation only (fig. 2). However, Cottonwood Spring, a site with shrub vegetation and tree overstory, had the lowest total breeding species. This may have been because it had the smallest area of all sites, and consisted of only three cottonwood trees with almost no shrub understory. Mopah Spring was classified as a site with shrub vegetation only, but fell within the range of breeding species for sites with tree overstory. The vegetation at this site was predominantly shrubs but included five palm trees and a number of large palo verde.
|
A seasonal status summary for avian species found at each site is shown in table 4. The largest group of species observed during the biweekly surveys was migrants. At 10 of 15 sites, more species were present during fall migration. The number of spring migrant species approximately equalled or exceeded fall migrants at the other five. Year-round resident species were uncommon at nearly all sites. Only Old Woman Springs and Iron Mountain Pumping Station, both large and diverse man-created systems, supported a significant proportion of year-round residents relative to the number of summer and winter residents. Summer residents were more common than winter residents at nine of 15 sites and approximately equal at the remaining six sites.
Species richness graphs for each site were classified into one of four general species richness patterns (table 4; fig. 3) based on shape (i.e., relative position and height of peaks). Five sites were classified as pattern A, summer and winter lows with nearly equivalent spring and late summer/fall peaks. Three sites were pattern B, high spring peak and relatively low late summer/fall peak. Cottonwood Creek and Dove Spring were the only examples of pattern C, with a spring high declining steadily to a winter low. The remaining five sites were pattern D, similar to pattern A except that the spring species richness peak was distinctly lower than the late summer/fall peak. Patterns A, B, and D, with late summer/fall peaks, reached highest values between mid-August and late October. These same patterns reached spring peaks from mid-April to early May; pattern C reached the spring peak in late May. All patterns reached winter lows from late December to early January and began to increase in mid-January.
Figure 4 shows the results from three study sites selected to represent the range of avian abundance patterns. Abundance was considerably more erratic than species richness because abundance was affected by time of survey, weather conditions, and encounters with large flocks. Highest abundances were reached in summer and fall at 13 of 15 sites (e.g., Cottonwood Creek and Horse Thief Springs). Nine were highest from mid-July to late August (e.g., Cottonwood Creek); six of these had peak abundances two to six times greater than any other time of year. The curves for the remaining four sites with highest abundances in summer/fall also had peaks from midJuly to late August, but these were exceeded by peaks from early September to mid-October (e.g., Horse Thief Springs). Kane Springs and Darwin Falls had lows in summer and peak abundances in early December.
|
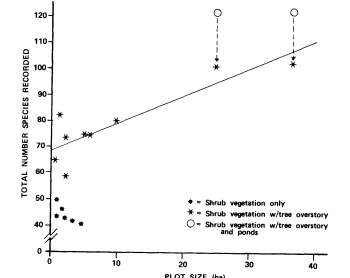
Figure 1.
Total number of species observed (species richness) as a function
of plot size and vegetation structure at 15 perennial desert riparian
study sites surveyed between November 1977 and January 1979.
Data points for sites with ponds adjusted to exclude species
dependent on open water before computing the regression
equation for plots with overstory trees (y= 1.05x + 69).
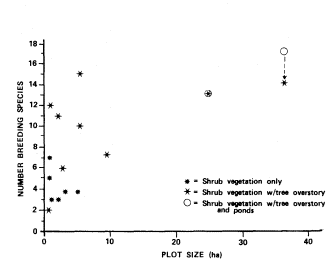
Figure 2.
Total number of breeding species (confirmed and probable)
as a function of plot size and vegetation structure at 15
perennial desert riparian study sites surveyed between
November 1977 and January 1979 (results from study
sites with ponds are shown with and without
species dependent on open water).
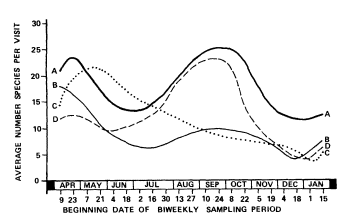
Figure 3.
Avian species richness patterns at 15 perennial desert riparian
study sites surveyed between November 1977 and January
1979. Pattern A—nearly equivalent peaks in spring and late
summer/fall. Pattern B— high spring peak and relatively low
late summer/fall peak. Pattern C—peak in spring declining
steadily to winter low. Pattern D—low spring peak and
relatively high late summer/ fall peak.
Most major abundance peaks were due to large numbers of summer, winter, or year-round resident ground granivores (i.e., California Quail, Gambel's Quail, Mountain Quail, Chukar, Mourning Dove, Horned Lark [at Old Woman Springs only], House Sparrow, House Finch, Black-throated Sparrow, Dark-eyed Junco, Brewer's Sparrow [at Cottonwood Creek only], White-crowned Sparrow, and Golden-crowned Sparrow). These species accounted for 50–90% of the height of summer/fall abundance peaks (fig. 4A and 4B). Most sites showed a small increase in bird numbers during spring migration. Generally this peak was not dominated by resident ground granivores and was more evident when these species were removed (fig. 4). Late fall/early winter populations varied in the proportion of resident ground granivores. The White-crowned Sparrow, a ground granivore, was a common winter resident (fig. 4C). However, two foliage insectivores, the Ruby-crowned Kinglet and the Yellow-rumped Warbler, frequently were more abundant than resident ground granivores (fig. 4A and 4B).

Figure 4.
Avian abundance patterns at three perennial desert riparian
study sites surveyed between November 1977 and January
1979. Upper line on each graph includes all species; lower
line excludes selected ground granivores (e.g., California
Quail, Gambel's Quail, Mountain Quail, Chukar, Mourning
Dove, House Sparrow, House Finch, Blackthroated
Sparrow, Dark-eyed Junco, White-crowned Sparrow,
Golden-crowned Sparrow).
Discussion
Importance of Desert Riparian Systems
The presence of trees or arborescent shrubs in riparian and non-riparian desert systems is an important variable influencing the breeding avifauna. Although quantitative data on vegetation-type structure are not provided, increasing breeding bird diversity, density, and species richness
are related with increasing amounts of trees and arborescent shrubs. Among desert riparian vegetation-types, cottonwood/willow, willow, and palo verde/ironwood typically have the greatest overstory canopy and thus have a wider availability of perches, nest sites, and foraging substrates. These vegetation-types support more breeding species at higher densities and have higher breeding species diversities than vegetation-types with less overstory (table 2). California fan palms supply a vertical habitat component but do not have the dense canopy provided by trees and arborescent shrubs. To a lesser degree, desert scrub with overstory, dominated by Joshua tree or ocotillo, also has a vertical component, but lacks much of the structural complexity that provides foraging and nesting substrate.
Overstory canopy is also important in non-riparian desert vegetation-types during the breeding season. Overstory vegetation decreases in amount from desert woodland to desert scrub with overstory to desert scrub. The number of breeding species and their density and diversity decline in a similar manner (table 2).
During the winter, birds are less dependent on trees and arborescent shrubs for perching and nesting. At that time, density, diversity, and species richness in riparian and non-riparian systems are not as well correlated with presence or absence of overstory canopy. Among riparian vegetation-types, palm had the highest bird densities in winter; species richness values there were comparable to cottonwood/willow and exceeded all other desert vegetation-types. This observation may be explained by the fact that California fan palms are restricted to the Colorado Desert, which is warmer in the winter than the Mojave and Great Basin deserts. Among non-riparian vegetation-types, desert woodland and desert scrub with overstory supported nearly equal numbers of species; bird densities in desert scrub exceeded desert scrub with overstory.
In addition to the frequent presence of trees or arborescent shrubs, riparian conditions generally result in a total vegetation that is denser than that found in non-riparian desert areas. This denser vegetation contributes to the higher bird densities found in desert riparian systems during breeding and winter seasons by augmenting the food and cover provided by trees and arborescent shrubs.
Surface water is also an important habitat component influencing bird use, especially during the summer months. Smyth and Coulombe (1971) have shown that granivorous birds, including Gambel's Quail, Mourning Dove, White-winged Dove, House Finch, and Black-throated Sparrow, drank regularly at Upper Carrizo Spring in the Santa Rosa Mountains of southern California. Insectivorous and frugivorous birds were seen drinking less regularly or not at all. Our study found that 13 species of summer, winter, or year-round resident ground granivores accounted for a large majority of birds seen at 15 perennial riparian areas (fig. 4). Nine of these species were present during the summer, but only two (House Sparrow and House Finch) nested almost exclusively in riparian vegetation. The rest of these birds presumably were drawn to the spring largely for water during the hot, dry summer months.
The importance of desert riparian systems to birds is not limited to large sites. Relatively small sites are used by many species over the course of a year (fig. 1); this is especially true for sites with tree overstory. Small sites with tree overstory generally support more breeding species than sites with shrub vegetation only (fig. 2). However, the number of breeding species does not show the correlation with plot size found for total number of species (fig. 1). Cottonwood Spring, the smallest site with overstory vegetation, almost completely lacked shrub cover under its three cottonwoods and supported fewer breeding species than any other site. However, Horse Thief Springs, the next larger site with overstory, had numerous large cottonwoods and patches of tall dense shrubs. It supported among the highest numbers of breeding birds.
Small sites also can support large numbers of individuals. The Cottonwood Creek site is approximately five times larger than Horse Thief Springs (table 1). However, Horse Thief Springs supported numbers of birds comparable to Cottonwood Creek during spring and winter and considerably more in summer and fall (fig. 4). Local factors including surrounding habitat, geographic location, topography, and availability of water in adjacent areas may explain why some sites support more birds than would be expected considering size alone.
The species composition of bird populations in desert riparian systems is extremely dynamic. This is reflected in the species richness patterns illustrated in figure 3. The typical pattern has peaks in spring and fall with lows in summer and winter. Relatively few species are resident in desert riparian systems; most are either transients, migrants, or vagrants (table 4). Breeding species typically are present only during the spring when food and water are most available and temperatures are relatively mild. However, the peak in number of individuals frequently is composed of relatively few species (fig. 4). At nine of 15 sites, sampled biweekly, peak abundance preceded peak species by approximately one month.
Explanations for variations in species richness patterns (fig. 3, table 4) must be tentative since they are based on the data for one year collected at biweekly intervals. Four of five sites with lower species richness in the spring than in the late summer/fall were at high-elevation montane locations. These sites remain colder and less productive later in the spring and may be cooler and more moist, and support food longer than lower elevation and valley sites in the late summer/fall. These reasons could explain the observed pattern. However, two high-
elevation montane sites, Cottonwood Creek and Dove Spring, had the highest species richness in the spring and declined throughout the year to winter lows. The reasons for this pattern are less obvious, especially since the number of migrant species recorded at each site was nearly the same in spring and fall (table 4). Iron Mountain Pumping Station, a low-elevation valley site, also showed a low spring peak relative to the fall. However, this site had an extremely high fall peak which may have been due to migrants being attracted to this large, highly visible site for food and water.
Medium to large sites in valleys and low-elevation montane sites had nearly equal species richness peaks in late summer/fall and spring. Small, low-elevation sites situated in valleys had high spring peaks and relatively low fall species richness peaks. Low species richness in the late summer/fall may have been due to lack of resources at very small, hot sites and lower probability that migrating birds could find these oases. Elevation, size, and visibility appear to be variables affecting the number of bird species using desert riparian systems during migration.
In the breeding season, more visiting species are found in desert riparian systems than in cismontane riparian systems (table 2). This pattern probably is due to the isolated nature of desert riparian sites and the relative unsuitability of adjacent desert areas for many birds. Water, cover, and food usually are more available in areas surrounding cismontane sites than those surrounding desert riparian sites. In the desert, many species found in adjacent areas must visit riparian sites. In addition, most studies of birds at desert riparian sites census the entire vegetation at the site. In cismontane locations, the riparian vegetation frequently is more widely distributed; study plots usually include only part of the available vegetation. Therefore, a greater percentage of the species utilizing riparian vegetation may be censused at desert sites than at cismontane sites at any given time.
Management Implications
Riparian systems associated with permanent water are very limited in the desert. However, washes, which are included in the preceding analysis, are more numerous. As we and others have shown, riparian habitats play a vital role in the desert ecosystem by providing water, food, and cover for resident and transient riparian birds, migrating birds, and non-riparian birds, such as quail and doves.
There are, in addition to the intensive use made of these high-value, low-quantity desert riparian systems by wildlife, numerous common high-intensity non-wildlife uses. For example, livestock and feral burros often congregate or linger in riparian habitats to take advantage of shade, forage, and drinking water. Recreationists are attracted to riparian systems in the desert by the shade and high visual quality. Washes are used as vehicular thoroughfares because they lack major obstacles. Mining operations frequently use the water associated with riparian systems in mining or mineral processing. They are also focal points of cultural resources, such as historic and native American sites.
Many of these uses are potentially in conflict with wildlife or maintenance of the riparian community. Riparian systems are generally recognized as vital to desert resident and migrant birds. Understanding the importance of riparian systems is vital to land managers as they attempt to resolve use conflicts. Resolution of these conflicts should take into consideration the breadth of resources which are critical to birds. Among these are the availability of surface water and the presence of a tree overstory and dense groundcover. Proper management should be aimed at maintaining or enhancing all of the individual components making up the riparian zone.
The geographic distribution of riparian systems should also be taken into account when assessing conflicts. Species such as Brown Towhee and Lazuli Bunting breed only in riparian vegetation in the Mojave and Great Basin deserts. Others, including Verdin, Abert's Towhee, and Black-tailed Gnatcatcher, generally are restricted to riparian systems in the Colorado Desert. An adequate distribution of well-managed riparian systems is essential, especially to the maintenance of species with limited distributions in the California deserts.
Results from biweekly surveys indicate that late December and early January is the time of lowest species richness (fig. 3) and bird abundance (fig. 4) at desert riparian sites. Winter bird-population studies conducted primarily in January and February show that midwinter to late winter species richness and abundance is higher. Thus, if it is necessary to create a disturbance such as building a fence or installing a management facility, construction during late December and early January is likely to have less of an impact than at other times. Of course, activities which damage habitat will be harmful to birds regardless of the season.
Human development and artificial habitats can be beneficial to the avifauna. Results of biweekly surveys (table 4) show that the two sites with the highest overall species occurrence (Old Woman Springs and Iron Mountain Pumping Station) are to a large degree man-made systems. Old Woman Springs (122 species recorded) includes a reservoir, residences, gardens, and ornamental trees mixed with native vegetation. Iron Mountain Pumping Station (121 species recorded) is an entirely artificial site with residential areas, parks, and a reservoir. These two sites were also especially high in number of year-round resident, fall migrant, and vagrant species. In addition to their structural diversity, with tall trees, buildings, dense shrubbery, and open water, these
sites were exceptionally large (36.4 ha. and 24.8 ha. respectively). Their low elevation (975 m. and 290 m. respectively) made both sites conducive to winter residents and winter transients. Thus, if properly mixed with native vegetation, artificial components can create structural diversity and provide nest and perch sites otherwise not available.
There are a few sites in the desert which are unusually rich in bird species. These frequently become focal points for birdwatchers (e.g., Morongo Valley, Furnace Creek Ranch). Such sites are normally large and diverse and support a substantial tree overstory. However, as knowledge of these sites increases, the importance of smaller, less well-known riparian sites can become obscured. Large sites have special management significance not only for the rarity of some of the birds found in them but also for the high level of public interest in their integrity. However, these sites and their contributions should not overshadow the importance of numerous other riparian sites and the contribution they make to supporting the desert avifauna.
Acknowledgments
We thank the many biologists who conducted and published breeding bird surveys and winter bird-population studies used in this report. Scott P. Horton developed the biweekly data collection methodology, selected study sites, and conducted initial surveys. Steven W. Cardiff performed biweekly surveys at one site. Kristin H. Berry supervised the wildlife program of the BLM California Desert Plan Staff. The final and several draft manuscripts were typed by Tracy Cortez. Figures were prepared by Clara Stapp.
Literature Cited
American Ornithologists' Union. 1957. Checklist of North American birds. 691 p. American Ornithologists' Union, Baltimore, Md.
American Ornithologists' Union. 1973. Thirty-second supplement to the American Ornithologists' Union checklist of North American birds. Auk 90:411–419.
American Ornithologists' Union. 1976. Thirty-third supplement to the American Ornithologists' Union checklist of North American birds. Auk 93:875–879.
Boyd, R.L., and C.L. Cink (ed.). 1980. Thirty-second winter bird-population study. No. 29, 42. Am. Birds 34:25–41.
Cink, C.L., and R.L. Boyd (ed.). 1981. Thirty-third winter bird-population study. No. 23, 28, 29, 41. Am. Birds 35:21–45.
Franzreb, K.E. 1978. Breeding bird densities, species composition, and bird species diversity of the Algodones Dunes. West. Birds 9:9–20.
Gaines, D. 1974. A new look at the nesting riparian avifauna of the Sacramento Valley, California. West. Birds 5:61–80.
Gaines, D.A. 1977. The valley riparian forests: Their importance to bird populations. p. 57–85. In : A. Sands (ed.). Riparian forests in California: Their ecology and Conservation. Institute of Ecology Pub. 15, University of California, Davis. 122 p.
Johnson, D.H., M.D. Bryant, and A.H. Miller. 1948. Vertebrate animals of the Providence Mountains area of California. Univ. Calif. Publ. Zool. 48:221–376.
Kolb, H. 1965. The Audubon winter bird-population study. Aud. Field Notes 19:432–434.
Loery, G. (ed.). 1967. Thirty-first breeding bird census. No. 35. Am. Birds 21:611–675.
Miller, A. H. 1940. A transition island in the Mohave Desert. Condor 42:161–163.
Miller, A.H., and R.C. Stebbins. 1964. The lives of desert animals in Joshua Tree National Monument. 452 p. University of California Press, Berkeley.
Ryder, R.A. (ed.). 1972. Twenty-fifth winter bird-population study. No. 23. Am. Birds 26:658–688.
Ryder, R.A. (ed.). 1973. Twenty-sixth winter bird-population study. No. 40. Am. Birds 27:666–703.
Ryder, R.A. (ed.). 1974. Twenty-seventh winter bird-population study. No. 45, 46, 48, 49. Am. Birds 28:695–733.
Ryder, R.A., and A. Ryder (ed.). 1975. Twenty-eighth winter bird population study. No. 35. Am. Birds 29:751–788.
Ryder, R.A., and A. Ryder (ed.). 1976. Twenty-ninth winter bird-population study. No. 48–52, 54–57, 67. Am. Birds 30:1040–1075.
Ryder, R.A., and A. Ryder (ed.). 1978. Thirtieth winter bird-population study. No. 48–51. Am. Birds 32:22–48.
Ryder, R.A., and A. Ryder (ed.). 1979. Thirty-first winter bird-population study. No. 23–27, 39–42, 47–55, 58–61, 63–66, 68–94. Am. Birds 33:18–53.
Shannon, C.E. and W. Weaver. 1949. The mathematical theory of communication. 117 p. University of Illinois Press, Urbana, Ill.
Smyth, M. and H.N. Coulombe. 1971. Notes on the use of desert springs by birds in California. Condor 73:240–243.
Svensson, S. 1970. An international standard for a mapping method in bird census work recommended by the International Bird Census Committee. Aud. Field Notes 24:722–726.
Tomoff, C.S. 1977. The spring avifauna of the Colorado Desert of southeastern California . 12 p. plus appendix. USDI Bureau of Land Management, Riverside, Calif.
Van Velzen, W.T. 1972a. Breeding-bird census instructions. Am. Birds 26:1007–1010.
Van Velzen, W.T. (ed.). 1972b. Thirty-sixth breeding bird census. No. 58–60. Am. Birds 26:937–1006.
Van Velzen, W.T. (ed.). 1973. Thirty-seventh breeding bird census. No. 79–82, 100. Am. Birds 27:955–1019.
Van Velzen, W.T. (ed.). 1974. Thirty-eighth breeding bird census. No. 107, 108, 110, 111, 129. Am. Birds 28:987–1054.
Van Velzen, W.T. (ed.). 1977. Fortieth breeding bird census. No. 111, 113, 125, 128, 129. Am. Birds 31:24–93.
Van Velzen, W.T. (ed.). 1978. Forty-first breeding bird census. No. 94–98, 108, 110, 111, 116, 125, 128–130, 134–142, 145–147. Am. Birds 32:49–125.
Van Velzen, W.T. (ed.). 1979. Forty-second breeding bird census. No. 119, 120, 132–136, 139, 140, 142–157. Am. Birds 33:54–114.
Van Velzen, W.T. (ed.). 1980. Forty-third breeding bird census. No. 121, 133, 134, 142–145, 148, 149, 152. Am. Birds 34:41–106.
Van Velzen, W.T. (ed.). 1981. Forty-fourth breeding bird census. No. 161, 174, 175. Am. Birds 35:46-112.
Management Aspects of Relict Populations Inhabiting the Amargosa Canyon Ecosystem[1]
Jack E. Williams, Gail C. Kobetich, and Carl T. Benz[2]
Abstract.—Amargosa Canyon is one of three areas within the 264-km. Amargosa River drainage of Nevada and California which contains permanently flowing water. The remainder of the river has desiccated since pluvial times and is now a dry wash. The extensive riparian community and associated habitats of Amargosa Canyon contain a large number of relict populations, including invertebrates (insects and molluscs), fishes (Amargosa pupfish and speckled dace), an amphibian (Amargosa toad), birds (Yellow-billed Cuckoo and Least Bell's Vireo), and mammals (Amargosa pocket gopher and Amargosa vole). Although the USDI Bureau of Land Management has designated Amargosa Canyon a roadless area, the ecosystem is threatened by illegal off-road vehicle use, exotic species, upstream urbanization, and mining of groundwater aquifers. Surveys are needed to determine the status of certain resident invertebrates, amphibians and mammals.
Introduction
The Great Basin and Mojave Desert are large physiographic congeries comprised of numerous basins and ranges. These geological and biological provinces are products of a complex history spanning hundreds of millions of years. Although much of the pluvial flora and fauna of the area undoubtedly has been lost, where relict ecosystems have persisted some of these species remain. One such relict ecosystem is the Amargosa River drainage, of which Amargosa Canyon is a unique and fragile segment.
Amargosa Canyon lies approximately 65 km. north of the town of Baker, San Bernardino County, California. At the head of the canyon is the small community of Tecopa (fig. 1). At the southern extremity the canyon opens onto a large alluvial plain bordered on the east by Dumont Dunes. The Amargosa Canyon ecosystem includes the Amargosa River from Tecopa Hot Springs to Sperry; springs near Tecopa Hot Springs and Tecopa; and Willow Creek (fig. 2). The canyon varies in width from about 122 m. in the northern portion to approximately 610 m. as it opens up to the south. It is about 20 km. in length. The depth of the canyon is variable with a maximum near the nexus of Willow Creek and the Amargosa River, where canyon walls are approximately 293 m. high.

Figure l.
The Amargosa Canyon ecosystem from
Tecopa Hot Springs to Sperry, Inyo and
San Bernardino Counties, California.
[1] Paper presented at the California. Riparian Systems Conference. [University of California, Davis, September 17–19, 1981].
[2] Jack E. Williams is Staff Ichthyologist, Gail C. Kobetich is Field Supervisor, and Carl T. Benz is Staff Mammalogist at the Endangered Species Office, USDI Fish and Wildlife Service, Sacramento, California.
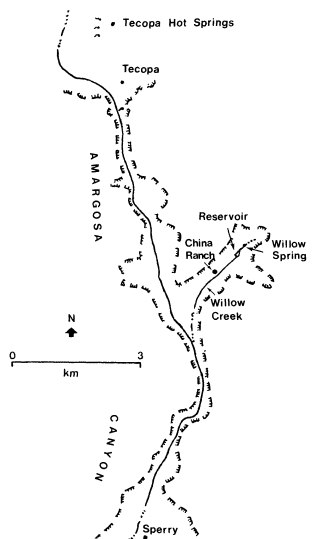
Figure 2.
The Amargosa River drainage, southwestern
Nevada and southeastern California.
The Amargosa Canyon ecosystem is an "ecological island". Isolated by geographical and climatic factors within the heart of the Mojave Desert, the extant flora and fauna have survived by adapting to the unique environmental conditions of their restricted habitats.
Tecopa Hot Springs is situated on lacustrine deposits of Pleistocene Lake Tecopa (Mason 1948). These spring waters flowing across the alkaline flat support a riparian vegetation characterized by bulrush (Scirpusolneyi ) and salt grass (Distichlisspicata ).
At the head of the canyon, and throughout it to the old mining site of Sperry, the native floral assemblage is representative of recent pluvial periods. Willow (Salixgooddingii ) and mesquite (Prosopis spp.) characterize thickets along the banks of the river. Cattail (Typhalatifolia ), bulrush, salt grass, rushes (Juncus spp.), and arrowweed (Plucheasericea ) create a moderate to dense vegetative barrier through which the river flows. The xeric upper slopes parallel to the river support salt bush (Atriplex spp.), buckwheat (Eriogonum spp.), creosote bush (Larreatridentata ), and others. Sawgrass (Cladiummariscus ) is found around many of the springs and seeps. Tamarisk (Tamarix spp.) is a major exotic component of the flora at the north end of the canyon and along Willow Creek.
Willow Creek is characterized by the richest botanical complex in the canyon system. Stands of cottonwood (Populus fremontii ) and thickets of mesquite, willow, and tamarisk dominate the visual scene, particularly along the creek and around Willow Spring and Willow Creek Reservoir. The spring and reservoir appear to be nearly overgrown with cattail.
Climate
The average annual precipitation during 1973 to 1980 was only 11.5 cm., with peaks occurring during winter and late summer months, as determined from a rain gauge at Shoshone. Extended periods with virtually no precipitation have been recorded during every season of the year.
During the period 1941 to 1970, January temperatures averaged 7.3°C., while July averaged 31.6°C. High summer temperatures, high rates of wind movement, and low humidity all interact to promote high rates of evapotranspiration. A maximum monthly evaporation rate of 502 mm. was recorded at Silver Lake, approximately 40 km. south of Amargosa Canyon (Pupfish Habitat Preservation Committee 1972).
Paleohydrology
The climate of western North America fluctuated widely during later Pleistocene and pluvial times. Pluvial periods were 4–8°C. cooler than at present and appreciably hydric. During these cooler and wetter periods, large lakes and extensive river systems occupied most basins, providing dispersal mechanisms for many aquatic and semi-aquatic organisms (Hubbs and Miller 1948; Mifflin and Wheat 1979; Miller 1946, 1948; Snyder et al . 1964).
The connections and extent of the Amargosa River drainage during later Pleistocene and pluvial times have been extensively studied; however, some differences of opinion still exist. In his studies of the fishes inhabiting southeastern California and southwestern Nevada, Miller (1946, 1948) proposed the name "Death Valley system" for what he believed was a large drainage system including the Amargosa, Mojave, and Owens Rivers, which all drained into Death Valley. Taylor (1980) disputed the existence of a Death Valley system based on his studies of the molluscan fauna. However, it appears clear that the current Amargosa River drainage at one time was occupied by three lakes, Lake Ash Meadows (16 km2 ), Lake Tecopa (254 km2 ), and Lake Manly (1,601 km2 ) (Snyder etal . 1964). At its maximum size, Lake Tecopa extended from north of
Shoshone through the Amargosa Canyon area. Lake Manly occupied most of the Death Valley floor, extending south to Saratoga Springs. Paleohydrographic evidence also indicates an occasional connection between the Mojave River and Amargosa River drainages near the vicinity of Silver Lake (ibid .). Wave-cut terraces, gravelbars, and deltas of pluvial origin provide easily visible evidence of the former water systems. The present Amargosa River drainage is but a shadow of its extent during late Pleistocene and pluvial times.
Hydrology
The headwaters of the Amargosa River occur in Oasis Valley north of the old mining town of Beatty, Nye County, Nevada (fig. 2). The Amargosa River drainage is anomalous in that the river flows southward from Oasis Valley, turns westward after flowing through Amargosa Canyon, and finally northward into Death Valley. The final sink of the 264-km. long river is at Bad Water, Death Valley. Much of the river flow is subterranean most of the year, particularly in summer. Three sections of the river wash—Oasis Valley, Amargosa Canyon, and a small (1.2-km.) section just northwest of Saratoga Springs—have perennial flows.
The largest permanent flow occurs through the Amargosa Canyon area (fig. 3). The river surfaces just north of Tecopa and continues through Amargosa Canyon for about 19 km. to the vicinity of Sperry. The entire ecological balance of the canyon, with few exceptions, depends on the natural surface water flows.
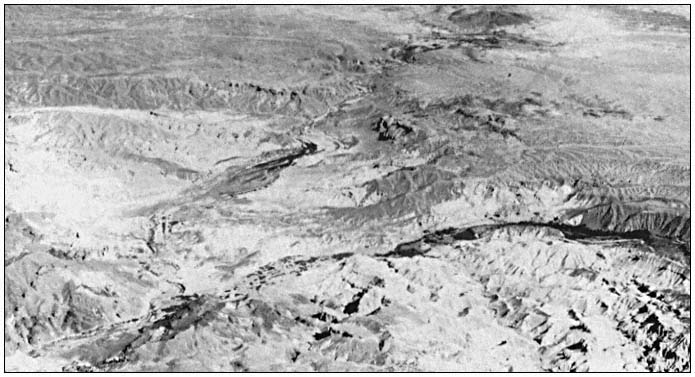
Figure 3.
Aerial view of Amargosa Canyon looking north. Willow Creek is the tributary canyon to the right.
The flow entering the canyon emanates from subterranean flows updrainage and springs in and adjacent to the community of Tecopa Hot Springs. The average discharge of the river at Tecopa during 1961 to 1968 was 2.21 cfs (USDI Geological Survey 1968). The Amargosa drainage occasionally experiences torrential floods, as in a 1965 storm that caused a maximum flow of 950 cfs.
In the northern half of the canyon, several minor springs and seeps feed the river. Willow Spring, the headwater of Willow Creek, flows all year. The surface flow of Willow Creek varies seasonally, and is often dry near its confluence with the Amargosa River during summer.
Total dissolved solids (TDS in milligrams per liter) vary considerably within the river because of differing water qualities of inflow springs. During a May 1981 survey, TDS varied from approximately 1,400 at the head of the canyon to 3,850 in the Amargosa River just above the inflow of Willow Creek.[3] The water quality
[3] Cindy Williams. California State University, Sacramento. Personal communication.
of Willow Creek is good; TDS is typically 700. The pH of the river water and inflow springs ranges from 8.0 to 8.4. Dissolved oxygen is high, approaching saturation throughout the river.
Geology
The geologic history of Amargosa Canyon is not totally understood. The canyon formed as a result of the Amargosa River cutting through an east-west trending uplift along the major axis of Sperry Hills. A Pleistocene lake, Lake Tecopa, was an ephemeral feature just north of the canyon. The outflow channel of Lake Tecopa is presumably the present-day Amargosa Canyon.
Various geological formations, the result of past geologic processes, are presently visible in Amargosa Canyon. At the north end, lacustrine deposits of Lake Tecopa, with associated vertebrate fossils, are visible (Mason 1948). Further down the canyon, erosional forces have exposed very coarse gravel and cobble (fanglomerates), thought to have been deposited contemporaneously with or after the Lake Tecopa sediments (ibid .). Upstream of the mouth of Willow Creek, rocks of volcanic origin such as tuffs, breccias, and undifferentiated volcanic flows of later tertiary origin have been exposed (Troxel 1961). South of the volcanics lie the China Ranch Beds, a series of light-colored, mainly white to brilliantly pink saline claystones with some interbedded gypsum layers. To the south of Willow Creek the geological sequence is reversed.
Downcutting by the river near the inflow of Willow Creek has exposed Precambrian rock, which is the probable basement for much of the canyon. From Tecopa to approximately 4.5 km. south of the Willow Creek confluence, moderate amounts of water flow at the surface throughout the year. Downstream of this area, water usually flows on the surface throughout the winter and spring months and occasionally during the summer. This suggests that the relatively impervious Precambrian rocks lie very close to the surface throughout the northern reaches of the canyon and are responsible for raising the subterranean water flow.
Relict Populations
Aquatic habitats in the desert obviously provide a special environment for plants and animals. Species diversity in such oases is often several times that of the surrounding desert. For example, a weekend bird count during April 1972 yielded 86 species within Amargosa Canyon (Pupfish Habitat Preservation Committee 1972). Since that time the number of bird species utilizing the canyon area has been found to be over 240.[4] The avifauna of the surrounding Mojave Desert is depauperate by comparison.
In addition to the great number of species utilizing desert riparian systems, the isolated nature of such systems as well as their relative scarcity often result in a biota containing species with greatly restricted ranges. A high degree of endemism often characterizes the fauna of isolated aquatic systems in the desert.
The fauna of the Amargosa Canyon ecosystem is notable in possessing many taxa of rare occurrence. These animals were often present over a much greater range during recent geologic history (e.g., the range of the pupfish has decreased from pluvial times) or their ranges have recently been reduced by habitat alteration (e.g., the range of the Yellow-billed Cuckoo has been reduced by destruction of riparian vegetation). These animal populations, which we refer to as relicts, are well represented in the canyon ecosystem, and include various invertebrates (insects and molluscs), three fishes (speckled dace and pupfishes), one amphibian (Amargosa toad), two birds (Yellow-billed Cuckoo and Least Bell's Vireo), and two mammals (Amargosa pocket gopher and Amargosa vole). Any management activities in the canyon area should consider the welfare of these representatives of the Amargosa Canyon fauna. The following population accounts provide information on identification, distribution within the canyon area, and any special considerations, such as the need for surveys, on the relict populations.
Invertebrates
A large degree of endemism occurs in the invertebrates of the Amargosa River drainage in Nevada and California. Endemism is especially notable in tiger beetles (Cicindelidae), creeping water bugs (Naucoridae), giant water bugs (Belostomatidae), assimineid snails (Assimineidae), hydrobiid snails (Hydrobiidae), and littoridinid snails (Littoridinidae). Members of these invertebrate families are either aquatic or restricted to water-related environments and have therefore been subjected to long periods of isolation from related species in other parts of the Amargosa River drainage and elsewhere. This isolation, and subsequent adaptation to the available habitat-types, has resulted in increased speciation and endemism.
The invertebrate fauna of Amargosa Canyon has not been as thoroughly scrutinized as that of the upper Amargosa River near Beatty, Nevada, or of Saratoga Springs. However, enough information is available to elucidate the distinctive nature of the fauna within the Amargosa Canyon area. The works of Rumpp (1956, 1957) have identified a variety of localized tiger beetles in the Amargosa River including Cicindeladenverensis propinqua , C . nevadica nevadica, and C . haemorrhagicahaemorrhagica , all from Tecopa and Tecopa Hot Springs. Cicindelawillistoni praedicta was described from the Amargosa River bed 5.6 km. south of Shoshone and may occur further downstream (Rumpp 1956). The Cicindela are colorful beetles usually found on damp sand or alkali beds along flowing stretches of rivers,
[4] Tarble, Jan. Los Angeles Audubon Society. Personal communication.
or in nearby grasses. The tiger beetles within the Amargosa River drainage are notable in their extreme reduction in maculation (spots and markings) (Rumpp 1956).
The most localized species of creeping water bugs in the California and Nevada region are Ambrysusfunebris , recorded only from Cow Creek in Death Valley, and A . amargosus , known only from Point-of-rocks Springs in Ash Meadows, Nevada (La Rivers 1953). So far neither of these species has been recorded from Tecopa or Amargosa Canyon. However, a more widespread naucorid waterbug, A . californicus , has been found in Willow Creek (Pupfish Habitat Preservation Committee 1972). Lethocerusangustipes , a giant water bug otherwise known only from Mexico, has been recorded from Saratoga Springs, just downstream from Amargosa Canyon (Usinger 1956).
The molluscan fauna of the Amargosa River drainage is diverse and includes a high proportion of localized endemics (67%) (Taylor 1980). The known fauna of 35 species within the California portion of the Amargosa River drainage represents approximately one-quarter of the entire freshwater molluscan fauna in California. Taylor (ibid .) reported two undescribed snails in the Tecopa area, the minute Amargosa spring snail, Fontilicella sp. (Hydrobiiddae), from Shoshone Springs and springs near Tecopa, and the Amargosa tryonia snail, Tryonia sp. (Littoridinidae), in the lower Amargosa River drainage from Ash Meadows to Saratoga Springs.
The primary threats to the Amargosa Canyon area invertebrate fauna include dewatering of the springs, surface alteration of spring and river habitats, and water pollution. Surveys are needed to document the invertebrate fauna and to assist in taxonomic investigations.
Fishes
Speckled dace and two subspecies of Amargosa pupfish are native to the Amargosa Canyon ecosystem. The speckled dace (Rhinichthysosculus ) occurs in a wide variety of habitats throughout most drainage basins in the western United States. Several isolated populations in the Great Basin and elsewhere have been described as subspecies (Hubbs et al . 1974). The population inhabiting Amargosa Canyon has been referred to as the subspecies nevadensis , originally described from specimens taken in Ash Meadows, Nevada (Gilbert 1893). However, the speckled dace in Amargosa Canyon are readily distinguishable from the small dace in Ash Meadows and probably deserve a new subspecific designation. The speckled dace also occurs in the upper Amargosa River near Springdale and Beatty, Nevada, but is absent from the Amargosa system below Amargosa Canyon.
Within Amargosa Canyon, the speckled dace occurs in Willow Creek, Willow Creek Reservoir, and sporadically throughout the Amargosa River. Recent surveys have found it to be rare throughout the canyon, comprising only 1.3% of the fish fauna.[3] The exotic mosquitofish (Gambusia affinis is partly responsible for low numbers of speckled dace.
Two subspecies of Amargosa pupfish (Cyprinodon nevadensis ) are native to the Amargosa Canyon area. The Tecopa pupfish (C . n . calidae ) is endemic to springs near Tecopa Hot Springs. The subspecies occurred in crenons, of approximately 32 to 40°C., below thermal springs that can exceed 42°C. at their sources (Miller 1948). Unfortunately, mining of underground aquifers and surface alteration of habitats caused the extirpation of the Tecopa pupfish during the 1970s. The second subspecies, C . n . amargosae , is known only from the Amargosa River near Tecopa and Tecopa Hot Springs, the Amargosa Canyon, and from the small perennial stretch of the Amargosa River northwest of Saratoga Springs. No pupfish are known from the Amargosa River near Springdale and Beatty, Nevada. Surveys conducted in 1980 and 1981 found the Amargosa pupfish in abundance—58% of the 11,485 fish sampled—throughout the canyon, but absent in Willow Creek.[3] The Amargosa pupfish occurs in a variety of river habitats within the canyon and often reaches a large size (greater than 60 mm. total length).
The primary threat to the Amargosa pupfish within the canyon area is the establishment of exotic species. The introduced mosquitofish probably competes with the pupfish for food, cover, and other requirements. The bullfrog (Rana catesbeiana ) often preys on pupfish within the canyon.
Amphibians
The Amargosa toad (Bufonelsoni ) is a small toad (maximum total length 72.5 mm.) about one-half the size of the western toad (Bufo b . boreas ) (Linsdale 1940). Distinctive features of the Amargosa toad include a narrow, wedge-shaped head, especially when viewed from below; smooth skin with weakly developed warts; and small feet with reduced webbing (ibid .). The preferred habitat of the species is freshwater marshes and areas along rivers in otherwise arid regions. The known distribution of the Amargosa toad includes the upper Amargosa River near Springdale and Beatty, Nevada, as well as the White River area of eastern Nevada. In his original description of B . nelsoni , Stejneger (1893) also recorded specimens from Resting Springs, northeast of Tecopa, and from Morans and Lone Pine in Owens Valley, California. Since that time no specimens have been recorded from California; however, we suspect that the species also occurs in the Amargosa Canyon area based on the abundance of available habitat and the proximity of recorded populations. A survey is needed to document the Amphibia of the area.
Birds
Localized breeding populations of Yellow-billed Cuckoo and Least Bell's Vireo occur in the Amargosa Canyon area. The historical breeding range of the Yellow-billed Cuckoo (Coccyzus americanusoccidentalis ) in California extended along the coastal areas from the Mexican border through the San Francisco Bay area, throughout the Sacramento and San Joaquin valleys, along the Colorado River, and in isolated areas of eastern California (Gaines 1974). However, the destruction of riparian vegetation has severely decreased the breeding range of this species. Gaines (ibid .) reported on the extensive tracts of riparian vegetation that appear to be requisite to nesting. These requirements include riparian vegetation at least 300 m. long and 100 m. wide, open water within 100 m., and dense understory vegetation and thickets of willows. Due to the removal of this essential habitat, the primary remaining breeding areas of the Yellow-billed Cuckoo occur along the Sacramento River between Red Bluff and Sacramento, and along the Colorado River (ibid .). The Yellow-billed Cuckoo has also fared well in Amargosa Canyon. Individuals typically arrive in the canyon in June. Breeding pairs have been observed at the north end of the canyon, at China Ranch, 0.8 km. west of Willow Spring, and at the confluence of Willow Creek and the Amargosa River (Gaines 1977; Tarble[4] ). The preferred habitat appears to be willows and cottonwoods within extensive areas of riparian vegetation.
The decline of the Least Bell's Vireo (Vireo belliipusillis ) is even more dramatic than that of the Yellow-billed Cuckoo. Until about 1930 the Least Bell's Vireo was common throughout a large portion of California including the Sacramento and San Joaquin valleys, coastal valleys, the entire southwestern part of the state, and the Owens Valley (Grinnel and Miller 1944). In 1944 Grinnell and Miller still considered the bird common although they noticed a considerable decline in numbers throughout southern California and in the Sacramento and San Joaquin valleys (ibid .). This decline continued throughout the known range of the Least Bell's Vireo. Goldwasser et al . (1980) determined that no other passerine in California has declined so dramatically and attributed the decline to a loss of riparian vegetation and to parasitism by Brown-headed Cowbirds (Molothrusater ). Least Bell's Viroes are often seen in the China Ranch area of Amargosa Canyon, especially near Willow Spring.[4] Sightings have also been made near the north end of Amargosa Canyon. The vireos always appear to nest near running water within the canyon area.[4]
Mammals
Two subspecies of mammals are endemic to the Amargosa River area near Shoshone and Tecopa. The Amargosa pocket gopher (Thomomysbottaeamargosae ) is known only from the Amargosa River near Shoshone and Tecopa. The type specimen was collected from the Amargosa River at Shoshone (Hall 1981). However knowledge of the subspecies near Tecopa is sparse. Annie Alexander's field notes, housed at the Museum of Vertebrate Zoology, University of California, Berkeley, indicated the presence of pocket gophers in sandy washes near Tecopa during a 1936 study. The Amargosa pocket gopher was one of 18 species of mammals recorded from the Amargosa Canyon area during a 1972 survey (Pupfish Habitat Preservation Committee 1972). The occurrence was presumed by the presence of distinctive mounds, rather that by trapping. Jim Patton[5] has trapped Amargosa pocket gophers near Shoshone High School and believes that they occur throughout the Amargosa Canyon area. However, a survey is needed to document the distribution and current status of the Amargosa pocket gopher. This subspecies burrows into moist sand within riparian vegetation which provides suitable cover and forage.
The Amargosa vole (Microtuscalifornicus scirpensis ) was described from specimens collected in 1900 from a spring near Shoshone. Habitat destruction was thought to have caused the extinction of this small marsh-dwelling rodent until a small population was discovered during the 1930s (Allen 1942; Bleich 1979). Subsequent surveys during 1978 and 1979 documented the presence of Amargosa voles just southwest of Tecopa Hot Springs and near Tecopa (Bleich 1980). The populations near Tecopa Hot Springs and Tecopa may be substantial; however, they are restricted to very small areas and are therefore susceptible to habitat changes. The primary threats to the vole are continued mining of underground water supplies and destruction of Scirpus marshes. Amargosa voles occur in bulrush marshes on flat (0% slope) to steep (20% slope) areas usually containing open water (ibid .). Marsh habitats within Amargosa Canyon proper need to be investigated to determine the presence of the vole.
Management
Management of any ecosystem should consider its fragility, its uniqueness, and its past, present, and future uses. The Amargosa Canyon ecosystem with its perennial water supply, its fragile soils, and its isolation, as well as its long history of human use, demands special treatment by modern man because of his ability and proclivity to inadvertently or intentionally destroy such natural treasures. Special protective status should be the first management strategy in any efforts to protect the Amargosa Canyon ecosystem.
Past Uses
This ecosystem has been in constant use by man for almost 10,000 years (Rogers 1939). Several types of lithic tools that represent at
[5] Jim Patton. University of California, Berkeley. Personal communication.
least four separate cultural groups are evident in Amargosa Canyon and its immediate vicinity. These cultures existed in sequential order from about 10,000 B.C. to the advent of Caucasian man. This is the only area in the Mojave Desert where these four cultures overlapped (ibid .). This, among other things, points out the uniqueness and importance of the Amargosa Canyon area. Even today the archeological work has not been thorough—much remains to be done. Any management scheme should be especially cognizant of the necessity to preserve this significant archeological study area.
The effects of prehistoric cultures on the Amargosa Canyon ecosystem can only be surmised from the scant clues left behind. We presume from their artifacts that the people were hunter/gatherers. We can surmise that they might have engaged in primitive agriculture, though there is no direct evidence for this. The "metates" and "manos" found in the area probably were used to grind seeds gathered from the wild. There are remains of sleeping circles, circle houses, trails, and figures and arrangements in the desert pavement. Regardless of the evidence for use of the Amargosa Canyon by prehistoric man, his influence on the ecosystem was minimal.
This unobtrusive use came to an abrupt end in the early nineteenth century when a route from the Rio Grande Valley in New Mexico (to become known as the Old Spanish Trail) was pioneered by Antonio Armijo. The trail was developed for the purpose of trading woolen goods to the California missions while donkeys and other livestock were driven back to New Mexico. On both legs of the trip, Amargosa Canyon, with its water and forage for livestock, was a welcome stopping place after the rigors of the surrounding desert.
The effects on the ecosystem of a large number of grazing animals is not known, but it must have been considerable. One herd of animals driven along the Old Spanish Trail numbered 4,150. As more people became familiar with Amargosa Canyon and the surrounding area, some mining activities began. No mines of any consequence were ever worked in Amargosa Canyon, though several small mines still exist on some of the drainages into the canyon. The major effect of this mining was indirect, though no less dramatic.
With the advent of the discovery of gold at Tonopah and Goldfield, Nevada, and borax in Death Valley, the economics of developing a transportation system to move materials to and from these mining centers improved sufficiently to allow for the construction of a railroad. Therefore, in 1905 Francis Marion Smith considered the task of constructing a railroad which would run from Tonopah to San Diego and become known as the Tonopah and Tidewater Railroad. Eventually Smith constructed the T & T Railroad from Ludlow, California, to Tonopah, Nevada. At Ludlow the T & T joined the Atchinson, Topeka, and Sante Fe Railroad. The route of the T & T from Ludlow to Tonopah was through Fort Soda, Silver Lake, Amargosa Canyon, Death Valley Junction, Beatty, and Goldfield. One of the major problem areas during construction of the T & T was Amargosa Canyon. The route through the canyon required much grading and building of several major trestles. The T & T operated profitably for some 25 years, but as the mines and ore bodies failed, so did the economics of running the T & T. In the late 1930s the T & T ceased operation, and near the beginning of World War II the Defense Department confiscated the rails and the T & T ceased to exist.
During its existence however, the railroad probably brought to Amargosa Canyon the most abrupt change that had ever occurred there. The grading of the route through the canyon, the building of the trestles, and the concomitant increase in general use of the area, especially during the construction phase, probably resulted in increased siltation, overgrazing of pasturage, and depletion or localized extirpation of larger animals. It was to be many years before the effects of this enterprise began to be ameliorated, and will be many more years before all vestiges of it will be gone. While there are some who may regret the passing of the T & T, the closing of the railroad and subsequent removal of the rails was undoubtedly one of the best defacto management decisions that could have been made for the good of Amargosa Canyon. That decision, if we can follow through with future wise management, signalled the end of intensive and disruptive use of the canyon.
Present Uses
Other human uses, beyond building and operation of the T & T Railroad, arose in the later nineteenth and early twentieth centuries. China Ranch was established in 1903 along Willow Creek. While the ranch is small and not terribly disruptive of the natural system, it represents a level of use not present before. The establishment of settlements in the vicinity of Tecopa Hot Springs and Shoshone brought downstream disruption from siltation associated with land disturbance and from decreased water flows associated with water use. These communities, according to the U.S. Bureau of Census (1973), accounted for approximately 1,000 people in the Amargosa Canyon area. However, these figures represent only the permanent residents—they do not indicate the significant increase in population during the winter when relatively large numbers of transients arrive to escape harsh northern winters and relax in the hot mineral baths at Tecopa Hot Springs. The effects of these winter visitors are not known, but could be considerable due to their additional demand on the limited water supply.
Human population in the area is likely to increase in the future. A large residential and industrial development is planned for the Ash Meadows area, upstream on the Amargosa River drainage. It is not known what effects this development will have on Amargosa Canyon, but we can surmise that the first effect could be a
decrease in subterranean water flows down the Amargosa River drainage, evidenced in decreased surface flows in Amargosa Canyon. For this reason, monitoring water quantity and quality will be of particular importance. Increased use from a larger human population will have to be scruti- nized for its effects on the canyon. Use of privately-owned lands within the canyon will also have to be monitored.
Altogether, there are four inholdings, totalling 623 ha. (1,538 ac.), of privately-owned property in Amargosa Canyon. Two inholdings, a 437-ha. (1,080-ac.) parcel and a 32-ha. (80-ac.) parcel, are owned by mining companies. The other two, the 88-ha. (218-ac.) China Ranch and a 65-ha. (160-ac.) parcel, are owned by local interests. To date the mining companies have not begun to develop their holdings. The owners of China Ranch and the 65-ha. inholding are managing these properties as they have in the past, with minimal disturbance to the natural system. However, if management of private lands should change or if extraction of minerals becomes economically feasible, the delicate riparian systems of the Amargosa Canyon could be disrupted. Therefore, as a sensible management tool, these private inholdings should be preserved in their present state by cooperative agreements, conservation easements, or any other mutually agreeable method.
Other than the 622 ha. of privately-owned land, the canyon is held in public trust by the USDI Bureau of Land Management (BLM). The BLM is therefore the major land manager within the canyon. In 1973, after being petitioned by interested conservationists, the BLM set aside its part of Amargosa Canyon as a roadless natural area. The resultant closure of the canyon to vehicular traffic has allowed the damage incurred from indiscriminate vehicular use to begin to be ameliorated. Access to the north end of the canyon and the canyon at China Ranch is effectively barricaded. However, at the more open southern end of the canyon, access is still possible, and off-road vehicle use, with its concomitant damage, is still occurring. However, the management provided by BLM should, with minor modifications, result in preservation of most of the canyon, and BLM is to be commended for its concern.
Future Use
The future for the relatively undisturbed nature of that part of Amargosa Canyon in public ownership appears to be good. The closure of the area to vehicular traffic by BLM must be maintained. There is always the possibility of disruptive use of resources on private land in the canyon. Continued increases in the population of the area will have an impact if there is excessive use of groundwater. Most of the adverse impacts generated by these possible uses can be held at to an acceptable level by proper management.
Management Recommendations
The management of an area such as Amargosa Canyon would be relatively simple if all land were in public ownership and if all upstream activities that could affect the system were controllable. Barring those desired circumstances, management recommendations will have to be formulated that will, if implemented, help safeguard the system. With that in mind, the following recommendations are offered for management of the Amargosa Canyon ecosystem. It is hoped that these recommendations would contribute significantly to the long-term protection of this beautiful desert aquatic/riparian system.
1. Erect an effective barrier to vehicular access at the south end of the canyon. This is a recommendation that needs immediate attention, as recent vehicular use is evident from tire tracks extending several miles northward from where the canyon opens onto the alluvial plain.
2. Secure the safety of the canyon by preventing the development and use of resources on the private land which would adversely impact the natural ecosystem. This could be done by cooperative agreement, conservation easement, purchase of mineral rights, fee acquisition, or other available mutually agreeable means.
3. Institute a water monitoring program to track on a regular schedule the flows of water in Amargosa Canyon at a point that would give the most meaningful data on water flows. A USDI Geological Survey stream flow monitoring station would be ideal for this purpose.
4. Monitor the quality of the water on a regular basis. Samples should be taken at least every two years. The frequency could be increased if samples indicated a significant deviation from the expected results or if increased use of the resources is evident.
5. Status surveys are needed to determine the presence and distribution of invertebrates, Amargosa toad, Amargosa pocket gopher, and Amargosa vole in the canyon.
6. Population status surveys of the two remaining fishes, the speckled dace and the Amargosa pupfish, should be conducted at two-year intervals to detect any long-term decline. These surveys should be carried out annually if water monitoring programs show significant changes or if other habitat changes, such as introduction of exotic species, occur.
7. A breeding bird survey should be conducted at two-year intervals to detect any change in population levels. Surveys could be carried out annually if there is reason to suspect a deleterious change.
8. BLM should maintain the status of Amargosa Canyon as a "natural area" and should maintain the closure to vehicular use. The added protection of declaring Amargosa Canyon an "Area
of Critical Environmental Concern" under the Desert Protective Plan is a welcome addition to the protective management decisions made by BLM.
Acknowledgments
The authors would like to gratefully acknowledge the help and cooperation of the following individuals: Ted Rado, BLM, Barstow; Jan Tarble, Los Angeles Audubon Society; Jim Patton, University of California, Berkeley; Chris Nagano, Los Angeles County Museum of Natural History; Bernie Troxel, University of California, Davis; Judy Maudlin, USDI Fish and Wildlife Service, Sacramento; and Larry Foreman, BLM, Riverside. Phil Pister, California Department of Fish and Game, Bishop, graciously let us use his photograph of Amargosa Canyon. We would especially like to express our appreciation to Cindy Williams, California State University, Sacramento, for typing the manuscript, but most especially for her scientific and editorial comments and her freely contributed data on the aquatic ecosystem of Amargosa Canyon.
Literature Cited
Allen, G.M. 1942. Extinct and vanishing mammals of the Western Hemisphere with the marine species of all the oceans. Am. Comm. Int. Wild. Prot. Spec. Publ. 11:1–620.
Bleich, V.C. 1979. Microtus californicus scirpensis not extinct. J. Mammalogy 60:851–852.
Bleich, V.C. 1980. Amargosa vole study. Final report to the California Department of Fish and Game. 8 p.
Gaines, D. 1974. Review of the status of the Yellow-billed Cuckoo in California: Sacramento Valley populations. Condor 76:204–209.
Gaines, D. 1977. Current status and habitat requirements of the Yellow-billed Cuckoo in California. Unpublished manuscript. 94 p.
Gilbert, C.H. 1893. Report on the fishes of the Death Valley expedition collected in southern California and Nevada, with descriptions of new species. No. Am. Fauna 7:229–234.
Goldwasser, S., D. Gaines, and S.R. Wilbur. 1980. The Least Bell's Vireo in California: a de facto endangered race. American Birds 34:742–745.
Grinnell, J, and A.H. Miller. 1944. The distribution of the birds of California. Pacific Coast Avifauna 27. 608 p.
Hall, E.R. 1981. The mammals of North America. Volumes I, II. 1181 p. Wiley-Interscience Publications.
Hubbs, C.L., and R.R. Miller. 1948. The zoological evidence: correlation between fish distribution and hydrographic history in the desert basins of western United States. p. 17–166. In : The Great Basin with emphasis on glacial and postglacial times. Bull. Univ. Utah Vol. 38.
Hubbs, C.L., R.R. Miller, and L.C. Hubbs. 1974. Hydrographic history and relict fishes of the north-central Great Basin. Calif. Acad. Sci 7:1–259.
La Rivers, I. 1953. New gelastocorid and naucorid records and miscellaneous notes, with a description of the new species Ambrysusamargosus (Hemiptera:Naucoridae). Wassman J. Biol. 11:83–96.
Linsdale, J.M. 1940. Amphibians and reptiles of Nevada. Proc. Am. Acad. Arts Sci. 73:197–257.
Mason, J.F. 1948. Geology of the Tecopa area, southeastern California. Geol Soc. Amer Bull. 59:333–352.
Mifflin, M.D., and M.M. Wheat. 1979. Pluvial lakes and estimated pluvial climates of Nevada. Nevada Bur. Mines Geol. 94:1–57.
Miller, R.R. 1946. Correlation between fish distribution and pleistocene hydrography in eastern California and southwestern Nevada, with a map of the Pleistocene waters. J. Geol. 54:43–53.
Miller, R.R. 1948. The cyprinodont fishes of the Death Valley system of eastern California and southwestern Nevada. Misc. Publ. Mus. Zool., Univ. Mich. 68:1–155.
Pupfish Habitat Preservation Committee. 1972. Amargosa Canyon-Dumont Dunes proposed natural area. Report to the USDI Bureau of Land Management. 118 p. plus appendices.
Rogers, M.J. 1939. Early lithic industries of the lower basin of the Colorado River and adjacent desert areas. San Diego Mus. Pap. No. 3.
Rumpp, N.L. 1956. Tiger beetles of the genus Cicindela in southwestern Nevada and Death Valley, California, and descriptions of two new species. Bull. So. Calif. Acad. Sci. 55:131–144.
Rumpp, N.L. 1957. Notes on the Cicindelapraetextata -californica tiger beetle complex. Description of a new subspecies from Death Valley, California (Coleoptera: Cicindelidae). Bull So. Calif. Acad. Sci. 56:144–154.
Snyder, C.T., G. Hardman, and F.F. Zdenek. 1964. Pleistocene lakes in the Great Basin. USDI Geological Survey, Misc. Geol. Investigation, Map I-416.
Stejneger, L. 1893. Annotated list of the reptiles and batrachians collected by the Death Valley expedition in 1891, with descriptions of new species. No. Am. Fauna 7:220–221.
Taylor, D.W. 1980. Endangered and threatened freshwater mollusks of Amargosa drainage, California-Nevada. Proposal submitted to USDI Fish and Wildlife Service. 18 p.
Troxel, B.W. 1961. Reconnaissance geologic maps of parts of the Leach Lake, Quail Mountains, and Silurian Hills quadrangles, California, scale 1:62,500. California Division of Mines and Geology.
U.S. Bureau of Census. 1973. Census of population, 1970. Volume I: characteristics of the population. Part 6: California, Section 1. U.S. Government Printing Office, Washington, D.C.
USDI Bureau of Land Management. 1980. The California desert conservation area. Final environmental impact statement and proposed plan. 173 p. California State Office, Sacramento.
USDI Geological Survey. 1969. Water resources data for California. Part I: surface water records. Volume 1: Colorado River Basin, Southern Great Basin, and Pacific Slope Basins excluding Central Valley. 498 p. USDI Geological Survey, Menlo Park, California.
Usinger, R.L. 1956. Aquatic Hemiptera. p. 182–228. In : R.L. Usinger (ed.). Aquatic insects of California. University of California Press, Berkeley.
The Decline of Ash Meadows, a Unique Desert Ecosystem[1]
Cynthia D. Williams[2]
Abstract.—Ash Meadows is a unique hydric ecosystem of the Amargosa Desert located in southwestern Nye County, Nevada, and southeastern Inyo County, California. The approximately 162-km2 area is dominated by over 30 springs and seeps that support a wide variety of plant and animal life, including nearly 30 endemics. Ash Meadows was apparently heavily used by several Indian cultures and later mined for peat and clay around 1910 to 1930. Since the late 1960s, the area has been subjected to a variety of agricultural, industrial, residential, and mineral development schemes, the most recent of which threatens to cause the demise of the ecosystem.
Introduction
Ash Meadow is a unique and biologically rich ecosystem, consisting of approximately 16,200 ha. in southwestern Nevada and southeastern California. It is located in the Amargosa Desert about 64 km. east of Death Valley and 145 km. northwest of Las Vegas (fig. 1). More than 30 springs and seeps discharge a total of 17,000–20,000 acrefeet of water annually in the Ash Meadows area, creating an oasis in the otherwise arid Amargosa Desert. Many of the springs are large, with headwater pools 6–10 m. in diameter (fig. 2). Water temperatures range from 26°C. to 38°C. The large spring discharge creates significant marsh areas for waterfowl and other migrating birds. At least 20 of the springs contain native fish, and most, if not all, contain endemic species of molluscs or insects. Additionally, several rare plants depend on the moist areas surrounding the springs for their survival.
As with much of the Great Basin, the Ash Meadows area was considerably wetter during the late Pleistocene, supporting Lake Ash Meadows as an ephemeral feature (Snyder etal . 1964). Because of elevational differences, the springs of Ash Meadows were isolated in three stages by the post-pluvial desiccation of Lake Ash Meadows. Devil's Hole, at approximately 732 m., was the first aquatic habitat to become isolated as the waters of Lake Ash Meadows receded. The period of isolation has been estimated at upwards of 30,000 years.[3] The mid-level springs, including Scruggs, Indian, Marsh, School, and Mexican springs, became isolated 4,000–10,000 years before present (YBP). Isolation of the lower elevation springs, including Big, Point-of-Rocks, Jack Rabbit, Forest, Bradford 1, Bradford 2, Bradford 3, Crystal, Longstreet, Rogers, and Fairbanks springs, occurred more recently and is still somewhat tenuous.
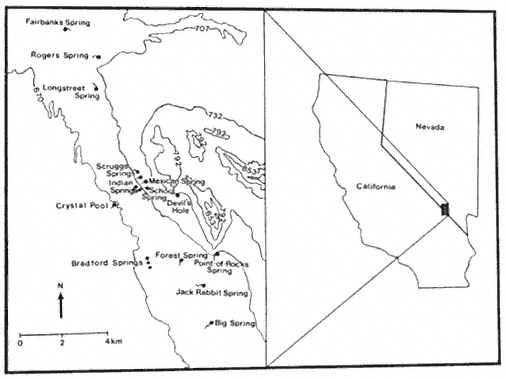
Figure l.
Location of Ash Meadows, Nye County,Nevada, and Inyo
County, California (modified from Soltz and Naiman 1978).
[1] Paper presented at the California Riparian Systems Conference. [University of California, Davis, September 17–19, 1981].
[2] Cynthia D. Williams is Graduate Teaching Assistant, Department of Biological Sciences, California State University, Sacramento.
[3] Deacon, J.E. Professor of Biology, University of Nevada, Las Vegas. Personal communication.

Figure 2.
Crystal Spring is one of the largest springs in the Ash Meadows area, with a discharge rate
of 10,741 LPM (Mifflin 1968). Note the extensive riparian zone surrounding the spring.
Species Accounts
Ash Meadows has the highest amount of biological endemism of any area in the United States. More than 25 organisms—including eight plants, two insects, 10 or more molluscs, five fishes, and one mammal—are endemic to Ash Meadows (table 1). Only one other arid region of North America, the Cuatro Cienegas Basin of northeastern Mexico, supports a greater diversity of endemic organisms (Minckley 1969).
Three of the endemic plants, Astragalusphoenix , Mentzelialeucophila , and Nitrophila mohavensis , are official candidates for inclusion on the federal list of threatened and endangered species. An additional two species and several varieties of plants are also endemic to the Ash Meadows area.
There are more than 10 snail species, including four species of an undescribed "Nevada Spring Snail" genus and approximately six species of Tryonia , endemic to springs in Ash Meadows. These snails are all candidates for federal listing. Ambrysusamargosus , a naucorid restricted to Ash Meadows, is also a candidate species.
Five fishes are endemic to Ash Meadows. The Ash Meadows killifish, Empetrichthysmerriami , was last seen in 1948 (Soltz and Naiman 1978). Apparently, E . merriami was a casualty of competition and predation by exotic organisms. Historically, this species occupied most of the large, lower-elevation springs. Three pupfish are endemic to Ash Meadows. The Devil's Hole pupfish, Cyprinodondiabolis , is restricted to Devil's Hole, a disjunct part of Death Valley National Monument, which encompasses 1.6 ha. (4 ac.) of the Ash Meadows system. This species of pupfish has undergone significant evolutionary changes (e.g., loss of pelvic fins) during its long period of isolation and is very distinct from its closest relative. In recognition of the precarious existence of C . diabolis , it is listed as endangered by the USDI Fish and Wildlife Service (FWS). The two Ash Meadows subspecies of Amargosa pupfish, Cyprinodonnevadensispectoralis and C . n . mionectes , inhabit the mid-level and lower springs, respectively. Cyprinodon n . pectoralis is listed as endangered by the FWS, and C . n . mionectes is an official candidate. A form of speckled dace, Rhinichthysosculusnevadensis , which inhabits many of the lower-
|
elevation springs is also a candidate to the list of federally protected species.[4]
The flora and fauna of Ash Meadows represent a unique and threatened biota, including two extinct and over 25 extant endemics. Many of the extant endemics have received official recognition of their precarious status, and such recognition is pending for many more.
Threats to the Ecosystem
Prior to the coming of the white man, Ash Meadows was apparently heavily utilized by several Indian cultures. This unobtrusive use of Ash Meadows came to an abrupt end at the beginning of the twentieth century. The first detrimental use of the area by man occurred from 1910 to 1930 when Carson Slough, an extensive marsh system in the western part of Ash Meadows, was drained for mining peat and clay. The mid-1940s saw man's continuing impact on the Ash Meadows ecosystem in the introduction of bullfrogs and crayfish to springs in Ash Meadows. Several shifts in abundance of native fishes at that time have been attributed to those introductions (Miller 1948). Since the 1940s a variety of tropical fish and a snail, Melanoidestuberculatum , have been added to the growing list of exotic species in the area (Hardy 1980).
During the late 1960s, Spring Meadows Ranch began extensive groundwater pumping for irrigation, and the water level in Devil's Hole began to decline, reaching an all-time low in 1972. The USDI National Park Service (NPS) began formal court proceedings in 1971 in an effort to protect both the Devil's Hole pupfish and the special geologic character of the limestone cavern in
[4] By emerging rule, the FWS declared Ash Meadows pupfish and the Ash Meadows speckled dace to be endangered species on 10 May 1982. This temporary ruling is in force for 240 days.
which the species resides. A preliminary injunction in 1973 established a minimum level below which water in Devil's Hole could not fall. The case was finally heard in the US Supreme Court, which, on 7 June 1976, established a minimum water level that is considered consistant with the continued survival of the pupfish (Deacon and Deacon 1979).
Subsequent to the Supreme Court decision the Spring Meadows Ranch was put up for sale, and was purchased by Preferred Equities Corporation. Preferred Equities currently owns about 5,665 ha. in the heart of Ash Meadows and most of the water rights. Plans have been completed for three major developments that include parcels for high density trailer lots, single family residences, and commercial areas.
In addition to the current threat from Preferred Equities Corporation, Ash Meadows is now facing pressure from mineral development. In 1980, Anaconda Copper Company purchased property near Big Spring with plans to expand a zeolite mining operation. The zeolite deposit extends northward from the currently worked area toward the center of Ash Meadows. Further to the northwest, Tenneco is mining and processing borax.
Management
Without a doubt, the recent industrial, residential, and mineral development schemes threaten to bring about the demise of the Ash Meadows ecosystem. The planned developments would greatly increase the number of people living and working in the area. In 1980, approximately 15 people lived in Ash Meadows. Preferred Equities' plans for 33,000 homes, and a high density trailer court would dramatically increase that number.
Increased population would result in a variety of direct and indirect impacts. A large amount of land would have to be cleared and leveled to provide homesites and many new roads would have to be built. Several springs would be physically altered for recreational purposes. Plans exist to build a health spa around Crystal Spring and a reservoir over Point-of-Rocks Springs. The incidence of exotic species introduction can be expected to increase with the growing human population. Similarly it can be expected that the native aquatic organisms will be transferred from spring to spring without regard to their historical ranges. Groundwater depletion could once again become a critical problem. Development of the magnitude envisioned by Preferred Equities Corporation would require vast amounts of water for which an adequate supply is questionable.
Ash Meadows presents the opportunity for one of the very few "all or nothing" management decisions. We must make the philosophical decision of whether or not Ash Meadows and its inhabitants are worth saving. If we decide that the Ash Meadows ecosystem should be preserved in perpetuity, federal or state acquisition and subsequent incorporation into a federal or state refuge becomes the only alternative. A vehicle for this action exists in the form of Senate Bill 41, introduced in the ninety-seventh Congress by Senator Alan Cranston. If passed this bill would authorize the establishment of a Desert Pupfish National Wildlife Refuge in Ash Meadows. Sound management of a refuge thus established could allow for protection of the ecosystem with leeway for some mineral development. Regardless of the outcome of S.B. 41, the protections, inherent in the National Monument System, which are now being applied to Devil's Hole should continue to be fully applied.
Acknowledgments
The manuscript was reviewed by Jack E. Williams of the FWS, who is thanked for his most helpful comments. James E. Deacon of the University of Nevada, Las Vegas, provided information on paleohydrology, but perhaps more importantly instilled in me an appreciation of the Ash Meadows ecosystem.
Literature Cited
Deacon, J.E., and M.S. Deacon. 1979. Research on endemic fishes in the National Parks with special emphasis on the Devils Hole pupfish. USDI National Park Service Trans. and Proc. Ser. 5:9–19.
Hardy, T. 1980. The Inter-basin area report—1979. Proc. Desert Fishes Council 11:5–21.
Mifflin, M.D. 1968. Delineation of ground-water flow systems in Nevada. Desert Res. Inst., Center for Water Resources Research Tech. Rep. H-W, No. 4. University of Nevada, Reno. 110 p.
Miller, R.R. 1948. The cyprinodont fishes of the Death Valley system of eastern California and southwestern Nevada. University of Michigan, Mus. Zool. Misc. Publ. 68:1–155.
Minckley, W.L. 1969. Environments of the bolson of Cuatro Cienegas, Coahuila, Mexico. University of Texas, El Paso Sci. Ser. 2:1–65.
Snyder, C.T., G. Hardman, and F.F. Zdenek. 1964. Pleistocene lakes in the Great Basin. USDI Geological Survey, Misc. Geol. Investigations, Map I-416.
Soltz, D.L., and R.J. Naiman. 1978. The natural history of native fishes in the Death Valley system. Nat. Hist. Mus. of Los Angeles Co., Sci. Ser. 30:1–76.
A Manager's Perspective of Riparian Areas in the California Desert[1]
Bruce Ottenfeld[2]
Ask 12 different people what riparian areas mean to them, and one will more than likely get 12 different answers. Even though riparian areas make up less than 19% of the desert and often have known names, people's perceptions will vary according to their interests. Here are a few of my perceptions. Riparian areas are:
1. areas of conflict;
2. rich in cultural values;
3. of religious significance to native Americans;
4. often found along historic transportation routes;
5. essential for survival;
6. areas of critical environmental concern;
7. essential to the livestock industry;
8. often essential for mining;
9. home for many rare plants, animals, and fish;
10. essential for a good share of human habitation in the desert;
11. vital to some agricultural development;
12. centers which attract the recreational user;
13. washes which provide routes of travel;
14. vital for the survival of the burro.
Are my perceptions anything like yours?
I wanted to point out my perceptions to show the many conflicts that arise almost daily in the riparian areas of the California desert. Riparian areas, more than any other zone in the desert, play a key role in its multiple use and management. By law they must be protected, and by law certain uses must be permitted. I believe these precious resources can be managed to permit uses and preserve their assets.
Let me give you an example. Corn Springs is a riparian area in eastern Riverside County for which a management plan has been prepared to give protection to a unique assemblage of resource values. Resource values present are vegetative, wildlife, cultural, scenic, and overall heavy recreational use. The plan provides for protection of these important resources while permitting a quality recreation experience. Some of the major proposed actions of the plan include:
1. move portions of existing camping areas away from critical vegetative and wildlife zones. This will directly benefit wildlife, vegetation, and scenic resource values;
2. remove one evergreen tamarisk and monitor the effect on the spring. This is intended to benefit wildlife, vegetation, and scenic quality;
3. install a sign and barrier in front of petroglyphs and nominate Corn Springs to the National Register. This will benefit cultural values;
4. increase USDI Bureau of Land Management (BLM) presence by rangers and other personnel. This will affect all resource values;
5. control vehicle use;
6. install nesting boxes and wildlife drinkers away from redesigned camping facilities.
7. institute a monitoring program to measure the effects of the management plan on the health of Corn Springs.
Corn Springs is but one riparian area in the desert for which we plan to develop a management plan. Each area will have a plan developed through interdisciplinary approval, and each plan will be unique depending on the conflicts. All plans will be implementable and will reflect the BLM's multiple-use mission.
[1] Paper presented at the California Riparian Systems Conference. [University of California, Davis, September 17–19, 1981.]
[2] Bruce Ottenfeld is Assistant District Manager, California Desert District, USDI Bureau of Land Management, Riverside, Calif.