1—
BIOGEOGRAPHY AND DYNAMICS OF CHANGE IN CALIFORNIA RIPARIAN SYSTEMS
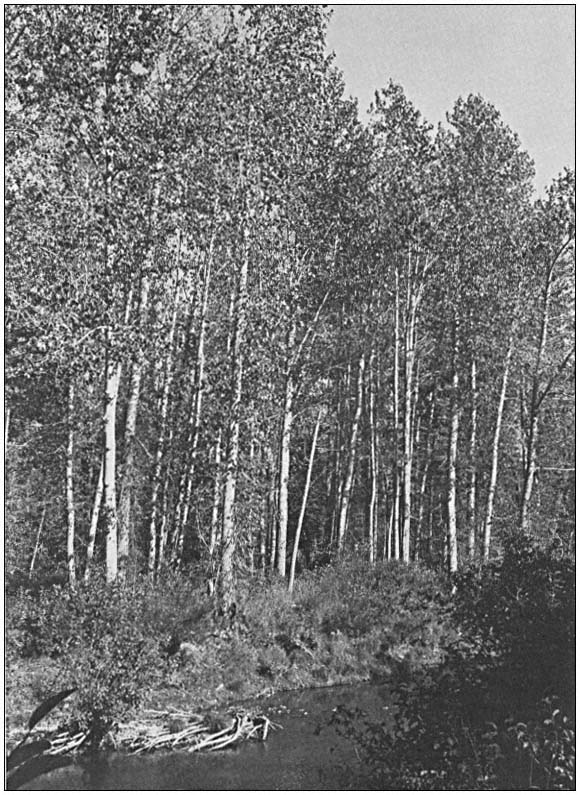
California Riparian Forests
Deciduous Islands in an Evergreen Sea[1]
Glen Holstein[2]
Abstract.—California riparian forests are dominated by deciduous trees and are thus anomolous in a state where most dominant woody plants are evergreen. Riparian zones provided refuges where riparian elements of the Arcto-Tertiary Geoflora could survive when its upland elements were decimated by the development of California's mediterranean-type climate. Water and nutrients imported to California's dry lowlands from wetter mountains by perennial streams permit high summer primary productivity in riparian communities while adjacent upland vegetation is severely drought stressed. High riparian productivity makes the cost of annual replacement of deciduous foliage affordable because such foliage is more photosynthetically efficient than that of evergreen upland dominants. Bird abundance and diversity in riparian communities are related to this high riparian productivity.
Introduction
Much of California has a mediterranean-type climate. In such climates rainfall and snowfall are maximal in winter, when minimal solar radiation limits plant growth. When the long days of summer potentially maximize growth, rainfall is minimal or nil and many plants are dormant or under severe drought stress. Thus moisture and solar radiation, two necessities for plant growth, are exactly out of phase (Major 1977).
Even near the moist northwest coast of California, fields of annual grasses in the hills above Redwood National Park (Humboldt County) are dead by late summer. Summer drought is also a major factor contributing to the uniqueness of California's alpine flora (Chabot and Billings 1972). Only at a few desert localities in California do some summer months have more rain than any single winter month, but here rainfall is so scanty and unpredictable at all times that vegetation is sparse and the flora limited to specialized drought resisters or evaders.
Most California vegetation is maximally productive in spring, when days are longer and warmer than in winter, and some moisture is still available. Stressful winter and summer conditions are thus both avoided. Productivity is less, however, than in ecosystems where light, warmth, and water are all simultaneously available in abundance. The productivity potential which is frequently unfulfilled in California because of summer drought stress is revealed by the increase in crop yield obtained there with irrigation, and by the productivity of the riparian vegetation which lines or once lined perennial streams. These streams carry the part of the winter water surplus which is slowly released from deep aquifers and melting mountain snow, making it available to lowland riparian vegetation in summer when little water is provided by the local climate. The resultant greater productivity and biomass of this vegetation is frequently obvious when contrasted with that of nearby communities which lack imported water. Riparian forests in central Asia ecologically similar to those of California's Central Valley are among the world's most productive natural ecosystems (Major 1977). When the current vacuum in California riparian research is filled, it is likely that riparian systems here will be found to be comparably productive.
Biogeography of Riparian Forest Components
Axelrod (1973) has provided compelling paleobotanical evidence that California's mediterranean-type climate is a relatively late phenomenon which first appeared in the upper Pliocene. This and other climatic perturbations caused the Arcto-Tertiary Geoflora, a zone of rich and diverse forest which was once continuous around the Northern Hemisphere, to retreat and become impoverished. Destructive impoverishment
[1] Paper presented at the California Riparian Systems Conference. [University of California, Davis, September 17–19, 1981].
[2] Glen Holstein is Lecturer, Botany Department, University of California, Davis.
of the Arcto-Tertiary Geoflora by spreading drought and cold was severe in western North America and Europe, but many of its elements survived in major refuges in eastern Asia, the Pontic region of southwest Asia, the Mexican highlands, and the southeastern United States. California's expanding mediterranean-type climate caused the replacement of many Arcto-Tertiary communities by drought-resistant vegetation known as the Madro-Tertiary Geoflora, which had long been adapted to local dry habitats (Axelrod 1975).
Riparian forests, as ecosystems in but not under the control of a mediterranean-type climate, seem likely refuges for Arcto-Tertiary elements within California, and Robichaux (1977) has shown in a review of their fossil record that most dominant California riparian forest taxa have modern ranges reduced from more widespread Tertiary distributions. These dominants all have relatives which are common in the Arcto-Tertiary derived deciduous forests of eastern North America (Axelrod 1960), and their dominance by deciduous trees and shrubs gives these vegetation types a similar aspect. Examination of the evolution and biology of the taxa dominant in California riparian forests provides further clues to the origin, evolution, and relationships of western North American riparian vegetation.
Acer (Maple)
This large genus, with 200 species of mostly deciduous trees, is one of the most important components of the temperate deciduous forests of the Northern Hemisphere, and its modern range coincides closely with those communities which are predominantly derived from the Arcto-Tertiary Geoflora (Hora 1981). It is by far the largest of the two genera in the Aceraceae and is the only one occurring naturally in North America, where it includes major upland dominants such as A . saccharum and widespread riparian species such as A . saccharinum and A . negundo .
The Aceraceae are part of the Sapindales (Cronquist 1968; Dahlgren 1975) or the essentially equivalent suborder Sapindineae of Thorne (1976), taxa which are otherwise largely dominated by entomophilous, evergreen, or drought-deciduous tropical to subtropical woody plants with compound leaves. The Hippocastanaceae are probably the closest relatives of the Aceraceae, and both of these families are unusual within the Sapindales because of their winter dormancy and largely north temperate distributions.
Acer consists mostly of winter-deciduous trees, but it is otherwise quite diverse and includes morphoclines both from compound to simple leaves and from flowers which are corollate and entomophilous to those which are reduced, apetalous, and wind pollinated. In both cases these cines reflect a shift from the primitive Sapindalean condition to a derived condition typical of the majority of dominant north temperate forest trees.
The four California species of Acer , A . glabrum , A . circinatum , A . macrophyllum , and A . negundo var. californicum , all can occur along streams, but only A . negundo (box elder) is primarily riparian. The other species are more common in mesic upland sites in the wetter parts of montane and coastal California, where gradients between riparian and upland vegetation are much more diffuse and less distinct than in the drier areas of the state.
A . macrophyllum is a particularly common and important tree throughout much of coastal and montane California, and it is listed by Roberts etal . (1977) along with Sequoiasempervirens , Umbellularia californica , and several more strictly riparian species as one of the common trees of California's north coastal riparian forests. In this region A . macrophyllum , S . sempervirens , and U . californica all occur from streambanks to the shaded, moist upland sites where they are most abundant.
A . negundo is frequent in riparian zones throughout the Mississippi basin and the Great Plains. Locally, it extends to the Atlantic coast and occurs along scattered streams and rivers in the southern Rocky Mountains, the Southwest, and California (fig. 1). Few other North American trees are transcontinental.
Within California A . negundo is locally common in riparian communities in the drier parts of the Coast Ranges and in the lower parts of the Sacramento and San Joaquin Valleys, where marine airflow through the Carquinez Straits somewhat moderates summer temperatures. Virtually nowhere in California, however, is it dominant. It is
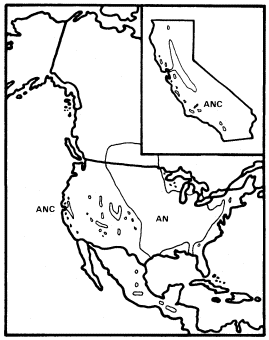
Figure 1.
Range of Acer negundo (AN) including A . negundo
var. californicum (ANC) (after Little 1971).
normally a shade-tolerant subordinate tree in dense riparian vegetation dominated by Populus fremontii , P . trichocarpa , Salixgooddingii , S . laevigata , S . lasiandra , or S . lasiolepis . Association with P . fremontii is especially frequent.
Dispersal of A . negundo is by wind dispersed samaras, but these are produced in smaller quantities and are less easily dispersed than the lighter, comose seeds of Salix and Populus . As a result, box elder is less efficient at colonizing the new riparian habitats which are frequently created on sandbars and along ditches and canals.
A . negundo appears to be in decline in California since it is a relatively poor competitor which has been restricted to the most highly competitive riparian zones. It may now be at an early stage of the process by which climatic vicissitudes eliminated (e.g., A . saccharinum and Ulmusamericana ) (Axelrod 1977) or almost eliminated (e.g., Juglanshindsii ) other riparian taxa from California whose relatives are still common in the much more extensive riparian systems of eastern North America, which receive summer rain.
A . negundo is taxonomically isolated among North American maples since it is the only member of section Negundo on this continent. This section is distinct enough to be segregated as the genus Negundo Boehm. by some (Willis and Airy Shaw 1973). Its other species are Asian, and it combines the putatively primitive character (within Acer ) of compound leaves with dioecy and inflorescences which are apetalous and anemophilous in A . negundo but corollate in at least some Asian species (Rehder 1940).
Alnus (Alder)
Alnus is a morphologically homogeneous genus of 35 species of deciduous trees and shrubs of the Northern Hemisphere and the Andes, and it has monoecious, anemophilous catkins and nutlets which vary among species in the degree of development of marginal wings and the resultant relative importance of wind, water, and gravity dispersal (Sudworth 1908; Fowells 1965).
It shares a distinctive pattern of ecological adaptation with some other important California riparian genera like Salix . These consist primarily of large, obligately riparian trees in warm temperate climates but are increasingly dominated by widespread shrubs which are only facultatively riparian in colder boreal and montane regions. Unlike other genera with large California riparian trees, however, Alnus contributes no important trees to North America's eastern deciduous forest. The commonest alder there is the shrubby A . serrulata . The adaptations needed by alders which are temperate riparian trees and those which are boreal and montane shrubs may not be greatly different since snowmelt frequently saturates soils of cold regions during the brief growing season and creates conditions similar to those found only along streambanks and lakeshores in warmer climates. Alders can symbiotically fix nitrogen, which otherwise may be limiting in forest environments and elsewhere (Spurr and Barnes 1980).
Alnus is a member of the Betulaceae, a family of mostly north temperate deciduous and anemophilous trees and shrubs which is in the Fagales (Cronquist 1968; Dahlgren 1975; Thorne 1976), an order it shares with the Fagaceae, to which it is linked by several intermediate genera (Corylus , Carpinus , Ostrya , and Ostryopsis ) sometimes placed in Betulaceae and sometimes in the segregate families Corylaceae and Carpinaceae (Willis and Airy Shaw 1973). The Fagales are the most important single order of angiosperm trees in temperate regions and most member taxa have Arcto-Tertiary distributions.
Alnus has four California species: A . sinuata , A . tenuifolia , A . rubra , and A . rhombifolia . The first two are largely shrubs of the boreal/montane type mentioned previously, but they can occasionally grow large enough along streams to be riparian trees (Sudworth 1908). The second two are among California's most important riparian trees. All California alders are in subgenus Alnus except A . sinuata which is in Alnaster .
Alnusrubra (A . oregona ), the red alder, is associated with the North Coastal Coniferous Forest (Munz 1959) from the Alaska panhandle to the coast of San Luis Obispo County (Little 1971; Griffin and Critchfield 1972). Within its range (fig. 2) it is frequently the dominant riparian tree. This largest of American alders (Elias 1980) is also very common on moist slopes, especially after conifers have been removed by logging operations, but it is much more likely to form dense and distinctive riparian gallery forests which it overwhelmingly dominates than are trees such as Acermacrophyllum , which are also found from moist slopes to streamsides. A fine example of such a gallery forest is protected along Prairie Creek in Humboldt County at Prairie Creek Redwoods State Park.
Alnusrhombifolia (white alder) forms similar gallery forests throughout much of the rest of California south and east of the range of A . rubra (fig. 2), but it much more obligately restricted to streamsides than its coastal relative. As a result, it is the most reliable indicator of permanent water among California's riparian trees (Jepson 1910). A . rhombifolia is the usual dominant in California's montane riparian forests up to about 1,600 m., but it also is dominant near sea level along Alameda Creek in Alameda County's Niles Canyon and numerous other similar places. It is most common along fast-flowing mountain streams west of the crest of the Sierra Nevada and near the coast south of Sonoma County (Griffin and Critchfield 1972). White alders are absent from much of the Central Valley floor but are common along the Sacramento River in Shasta County (ibid .) and further south (Conard et al . 1977). Such
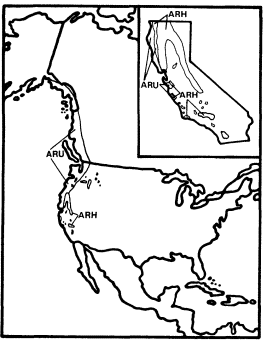
Figure 2.
Ranges of Alnus rubra (ARU) and A . rhombifolia
(ARH) (after Little 1971, 1976).
montane-coastal distributions suggest intolerance for summer heat (e.g., Populustrichocarpa ), but this is unlikely in the case of A . rhombifolia since it is common in the vicinity of Redding, where summer temperatures are as high or higher than in most of the area where it is absent. The ecological factor which most controls the distribution of A . rhombifolia seems to be a need for constant saturation of its root zone by cool, well-aerated water.
The total range of A . rhombifolia extends from southern California to central Washington in the Peninsular, Transverse, Coast, Sierra Nevada, Klamath, and Cascade ranges, with an extension through the Columbia River Basin to northwestern Idaho (Little 1976). It is most common and its range most continuous in the Sierra Nevada, Klamath Mountains, and northern Coast Ranges of California. A . rhombifolia and A . rubra are morphologically similar and closely related, but hybrids between them do not seem to have been reported. They apparently diverged from a common ancestor at an unknown time in the Tertiary or Quaternary Periods and adapted to wet and riparian sites within the Sierran-Klamath and north coastal forests, respectively.
Betula (Birch)
Betula is the second genus in the Betulaceae when that family is narrowly defined to exclude the Corylaceae and Carpinaceae (Willis and Airy Shaw 1973). Its characters are similar to those of its sister genus, Alnus , which it resembles in its deciduous habit, its anemophilous catkins, its north temperate range, and its nutlets, which are more consistently winged and wind dispersed than those of Alnus . Betula also shares a similar range of adaptations with Alnus since it includes both temperate zone trees and arctic and montane shrubs, but Betula contributes many more important upland trees to the deciduous forests of eastern North America than Alnus , which is more important as a source of dominant trees in western riparian forests than Betula .
California has two species of Betula : B . occidentalis (B . fontinalis ) and B . glandulosa , but only the former reaches tree size. B . occidentalis (water birch) is a large shrub or small tree of riparian sites which is widespread in the cordilleran region of western North America (Little 1976) but is restricted to just a few parts of California. It is relatively frequent in the Klamath Mountains and on the east slope of the southern Sierra Nevada, but much less so in the Warner, White, and Panamint Mountains (fig. 3). The Klamath and Warner Mountains have an abbreviated summer drought because of their northern locations, and the southern Sierra Nevada's east slope and the White and Panamint Mountains all regularly receive summer thunderstorms of tropical origin. As a result, all California populations of B . occidentalis receive quantities of summer rain which are unusual for that state and which approach the greater amounts received by the much larger populations in states to the east and north. Consequently, lack of summer rain must be suspected as an ecological factor limiting the range of this species despite its adaptation to riparian zones. Non-riparian Pinus balfouriana and other taxa have similar distributions for similar reasons (Raven and Axelrod 1978). Seedlings can be much more sensitive than mature

Figure 3.
Range of Betula occidentalis (BO) (after Little 1976).
plants to environmental stresses like summer drought (Grime 1979).
B . occidentalis is in series Albae within Betula (Rehder 1940) and is thus a close relatie of the white briches such as B . papyrifera , B . pendula , and B . pubescens , which are very important early successional trees throughout the upland boreal forests of North America and Eurasia.
Cephalanthus (Button Bush or Button Willow)
Cephalanthusoccidentalis , an obligately riaprian small tree or shrub, is the single California representative of this genus of 17 species which is widespread in the warm regions of the world and is one of only three California genera in the very large (500 genera and 7,000 species) family Rubiaceae, best known in temperate regions for the large and usually herbaceous genus Galium . Most of the Rubiaceae, however, are understory trees and shrubs in tropical forests. The family is clearly of tropical derivation and its placement in the Gentianales by Dahlgren (1975) and Thorne (1976) and in the related Rubiales by Cronquist (1968) reflects considerable consensus concerning its evolutionary relationships. Cephalanthus is in the subfamily Cinchonoideae, which is largely woody and tropical, rather than in the Rubioideae, which includes most of the family's temperate herbs and its other California genera.
C . occidentalis is primarily a deciduous shrub and only rarely reaches tree size in California. Its flowers are small but corollate and probably entomophilous like those of most Rubiaceae, and the fruit is a dry schizocarpic mericarp which lacks obvious adaptations for dispersal.
Like A . negundo , C . occidentalis is found naturally in both Atlantic and Pacific coast states (fig. 4). It is widespread in the East and ranges south through Mexico to Honduras, but it is restricted to a few Arizona stations and to the floor and adjacent watershed of California's Central Valley in the West (Little 1976). In California and in most of the rest of its range it is limited to areas with mean July temperatures above 20 C where most of the root zone is reliably saturated with water throughout the year. Relatively poor dispersal has made it an inefficient colonizer of the banks of artificial ditches and canals, but it is still common along many permanent natural streams. In backwaters where still, poorly oxygenated water stands throughout the year, C . occidentalis is best developed and can be dominant (Conard etal . 1977), but such habitats in California have been almost entirely destroyed by water resource and agricultural development. Their Button Bush Swamp Forest vegetation type is thus among the rarest and most endangered in the state. A particularly fine example of this vegetation is still extant along the Cosumnes River in southern Sacramento County west of Galt, and its continued preservation should receive high priority from California's conservation community.
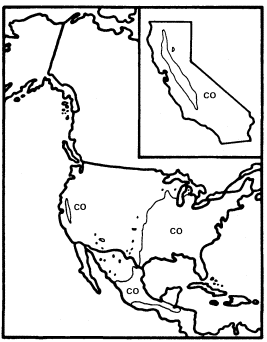
Figure 4.
Range of Cephalanthus occidentalis (CO) (after Little 1976).
It is clear that C . occidentalis in California is a relict which has survived the loss of a warmer and wetter climate because of the fortuitous juxtaposition of the hot Central Valley and the high mountains which surround it and keep it continuously supplied with abundant water.
Fraxinus (Ash)
This genus has a range which matches almost exactly that of the Arcto-Tertiary Geoflora, and it shows a range of adaptations including deciduousness, anemophilous catkins, and wind-dispersed samaras which is typical of the flowering trees in the modern forests derived from it. Fraxinus clearly evolved these characters by convergence, however, since its probable ancestors had few if any of them.
It is a member of the Oleaceae, whose placement in the Oleales by Dahlgren (1975) and Thorne (1976) and in the Scrophulariales by Cronquist (1968) only hints at the lack of consensus among plant evolutionists about its origin. Most other members of the family have entomophilous flowers with well-developed corollas, and many are tropical species with evergreen leaves and fleshy fruits adapted to internal dispersal by birds. Fraxinus itself includes a floral reduction series which suggests the mode of evolution of its apetalous, wind pollinated catkins since several species along the southern periphery of its range, including F . cuspidata in the southwestern United States and northern Mexico and F . ornus in southeastern Europe, have
fragrant entomophilous flowers with conspicuous corollas. Evergreen leaves are less common, but they occur in F . gooddingii of southern Arizona and northern Sonora (Elias 1980).
Most ash species like F . cuspidata and F . ornus which have primitive characters typical of the Oleaceae are included in section Ornus . Section Fraxinaster , however, includes many species of upland (F . americana , F . excelsior , F . quadrangulata ) and riparian (F . nigra , F . pennsylvanica ) trees which are important in north temperate deciduous forests and share many characters with the trees most highly adapted to that ecosystem in other families (Rehder 1940).
California is usually considered to have four Fraxinus species (F . dipetala , F . anomala , F . latifolia , and F . velutina ) (Munz 1959). F . dipetala is interesting among these as a Fraxinaster species with a corolla (Rehder 1940) and F . anomala for its frequently simple leaves, but only F . latifolia and F . velutina are important riparian trees in California (F . anomala is a riparian species of the Colorado Plateau with a few relict populations in the mountains of the eastern Mojave Desert). These are only nominally species, however, since the riparian ashes of California (fig. 5) are part of an attenuated but essentially continuous cine between more important ash populations in Arizona (F . velutina ) and in Oregon and Washington (F . latifolia ) along which species can be separated only artificially and arbitrarily (Griffin and Critchfield 1972).
The Pacific Northwest has a relatively short summer drought because of its northerly latitude, and Arizona regularly receives heavy summer thunderstorms of tropical origin, so both areas have much more summer rain than California. There ashes must rely almost entirely on riparian water during the growing season, and probably as a result, they are a very subordinate component of the state's riparian forests except near its northern border, where F . latifolia becomes more important as the climate becomes more like that of Oregon. In the rest of California F . latifolia /F . velutina occurs sparsely as a non-dominant riparian tree in the northern Coast Ranges, the Central Valley, the west slope of the Sierra Nevada, and in southern California, but it is rare or absent in the southern Coast Ranges, where summer drought is especially strongly developed and very few streams are naturally permanent. The Tehachapi Mountains and the western Transverse Range are conventionally used to separate these "species" in California (Griffin and Critchfield 1972).
The biology and distribution of California's riparian ashes suggest that they are declining relicts like Acer negundo var. californicum which are probably somewhat more tolerant of heat and low humidity but less tolerant of summer soil moisture deficits than that taxon. On a larger scale, Arizona and the Pacific Northwest appear to be relict nodes where populations of a once more widespread (Robichaux 1977) and probably continuously transcontinental riparian ash have successfully survived Quaternary climatic perturbations that eliminated it in much of the West and greatly reduced it in California. F . velutina and F . latifolia are, in fact, both similar enough to riparian F . pennsylvanica of the eastern United States (fig. 5) to be considered its subspecies (Miller 1955).
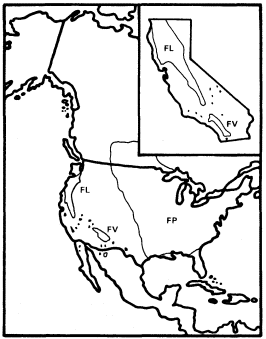
Figure 5.
Ranges of Fraxinus latifolia (FL), F. velutina (FV),
and F . pennsylvanica (FP) (after Little 1971, 1976).
Juglans (Walnut)
This genus of deciduous trees has a largely Arcto-Tertiary distribution like many of the other important California riparian genera, but its distribution within the Arcto-Tertiary zone is incomplete because of its absence from large areas, including much of Europe. It is best developed along the southern margins of this zone and extends far south of it to Argentina along the Andes.
Juglans and Carya are the only genera in their family, the Juglandaceae, which still include widespread and important north temperate forest trees. Both have distributions which suggest reductions from formerly more complete Arcto-Tertiary ranges. The other genera of the family, Pterocarya , Engelhardtia , Oreomunnea , Platycarya , and Alfaroa , are all restricted to much smaller warm temperate to tropical Arcto-Tertiary refuges in Middle America or Asia.
The Juglandaceae have often been treated as a distinctive order, the Juglandales, and associated (in the subclass Hamamelidae or Amentiferae)
with other families of temperate trees which share their characters of large-seeded woody fruits and anemophilous catkins (Cronquist 1968), but much current opinion (Dahlgren 1975; Thorne 1976) interprets these similarities as convergence and places the Juglandales close to or in the Sapindales/Rutales, the order of compound-leaved tropical trees from which the Aceraceae were also derived through a separate lineage.
The United States has six of the world's 15 species of Juglans , but J . cinerea is the only strongly distinctive species among these six. The other five include J . nigra , an important upland to weakly riparian forest tree of the eastern deciduous forest (Fowells 1965), and four species of various refuge areas in the West (fig. 6). The western species closely resemble J . nigra and suggest the same pattern of Late Tertiary to Quaternary reduction in the range of a formerly transcontinental species which we have seen in Fraxinus . These four include the two California species of Juglans , J . californica and J . hindsii , both of which are endemic to the state.
J . californica is a mostly non-riparian tree of southern California which is depauperate relative to J . nigra and J . hindsii but is not greatly different from them morphologically. It is restricted to deep, friable Tertiary marine shales with high water-holding capacity which permit it to survive as a local dominant on upland sites since the warm spring temperatures of southern California allow summerwet conditions to be simulated earlier in the year wherever soil storage capacity is adequate to hold surplus water from winter rains.
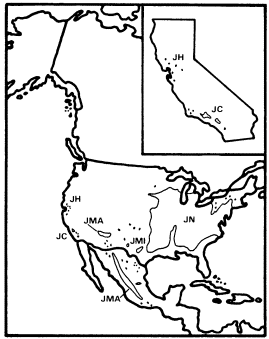
Figure 6.
Ranges of Juglans hindsii (JH), J . californica (JC),
J . major (JMA), J . microcarpa (JMI), and
J . nigra (JN) (after Little 1971, 1976).
J . hindsii was apparently restricted to a very few sites in central California when European settlement began there, and at least some of these sites were riparian (Griffin and Critchfield 1972). Since this species was probably derived from ancestors which were adapted to a summer-wet climate and were only weakly riparian, it is likely that J . hindsii was escaping a mediterranean-type climate to which it was completely unadapted in a riparian zone to which it was poorly adapted. Its large, nutrient-rich seeds in heavy nuts with little obvious capacity for dispersal are a more appropriate adaptation for reproduction in a stable forest (Grime 1979) than in the highly unstable, frequently floodprone riparian environment that existed in California before most of its streams were dammed.
J . hindsii was clearly on the verge of natural extinction when California was first settled by Europeans and was at the midpoint on a continuum between those taxa with similar histories such as Nyssa and Ulmus which are now known only from California's fossil record (Axelrod 1973) and those such as Acernegundo and Fraxinuslatifolia which survived until the settlement period with greater but still declining ranges and abundances (Robichaux 1977). Ironically, since European settlement, J . hindsii has been widely planted and subsequently commonly naturalized in California's now largely stabilized riparian systems at a time when its once much more abundant congener J . californica is declining rapidly because of the urban expansion of Los Angeles.
Platanus (Sycamore)
Platanus , even more than Juglans , is an example of an old, declining Arcto-Tertiary genus, since it is now largely restricted to warm temperate to tropical refuges along the southern periphery of the Arcto-Tertiary zone despite an extensive and diverse fossil record from as far north as Greenland (Engler and Melchior 1964). Only P . occidentalis of the eastern United States is still an important forest tree in a major north temperate forest biome.
Platanus is traditionally placed in the monotypic family Platanaceae, which is widely agreed to belong in the order Hamamelidales (Cronquist 1968; Dahlgren 1975; Thorne 1976), a relationship which links it to several other old families of Arcto-Tertiary trees and shrubs. The Hamamelidales show ancient tendencies toward the deciduous tree habit, unisexual wind pollinated flowers, and wind dispersed fruits, characters which are typical of the modern dominants of north temperate forests and of all 10 living species of Platanus , a taxon which culminates one of probably several floral reduction series within the order and its relatives (Thorne 1976).
Platanus in the United States includes P . occidentalis and two closely related species of the Southwest, P . wrightii of Arizona, New Mexico, and northwestern Mexico, and P . racemosa of California (fig. 7). All three species are strongly riparian, but the two southwestern species are more similar to P . orientalis , a riparian tree native from southeastern Europe to the Himalayas, than to the geographically closer P . occidentalis (Hsiao 1973). This may reflect ancient relationships and patterns of extinction within Platanus , but the very close relationship between P . racemosa and P . wrightii suggests they were separated relatively recently when expanding deserts separated woodlands that were continuous between Arizona and California in the Miocene (Axelrod 1975; Raven and Axelrod 1978).

Figure 7.
Ranges of Platanus racemosa (PR) and
P . wrightii (PW) (after Little 1976).
P . racemosa is a common riparian tree in northwestern Baja California, southern California, the southern Coast Ranges, the southern Sierra Nevada foothills, and the Sacramento Valley, but it is scarce in the San Joaquin Valley and absent from the northern Coast Ranges, where much seemingly suitable habitat occurs (Griffin and Critchfield 1972). P . racemosa is an important secondary component of the mixed riparian forests of the Sacramento Valley, where it is often associated with sites higher and drier than those where dominant Populusfremontii is found (Conard et al . 1977), but it is particularly conspicuous as frequently the single dominant tree along the intermittent streams of the southern Coast Ranges and southern California. Very large sycamores form an open woodland along such streams, and fine examples of this distinctive vegetation-type can be seen along Pacheco Creek in southern Santa Clara County and along Orestimba Creek in Stanislaus County.
Intermittent streams are general in southern California and the southern Coast Ranges because of their mediterranean-type climate and lack of extensive highland snowfields to provide summer runoff, and sycamore woodlands are particularly characteristic of those with beds of coarse, porous sand and gravel. Such substrates are common in the region where sycamore woodlands occur because it has been subjected to very rapid and recent Plio-Pleistocene uplift which may still be continuing and to massive erosion which has inevitably followed (Page 1981). The small, comose achenes of P . racemosa are easily carried long distances by wind, enabling the rapid reestablishment of these woodlands after the flood damage which was frequent before California streams were dammed.
The obligate restriction of P . racemosa to riparian zones indicates a need for access to groundwater within its root zone, but its preference for dry, porous sites within riparian zones suggests that for a riparian species it also has a rather high requirement for aeration of at least part of its root zone. The reasons for its complete absence from the northern Coast Ranges are not obvious since seemingly suitable intermittent streams with coarse beds are fairly common there. Along some of these streams on the dry east slope of the northern Coast Ranges native riparian trees are replaced by halophytic graminoids and an introduced Tamarix species, probably because their flows are made brackish by salts leached from the Cretaceous marine sediments which dominate their watersheds,[3] but many other northern Coast Ranges streams have fine riparian gallery forests along their banks. The clay-rich Franciscan Formation dominates the northern Coast Ranges, but the same formation yields enough sand and gravel to support major stands of sycamore woodland in parts of the southern Coast Ranges. Temperature does not provide a simple explanation for the absence of sycamores from the northern Coast Ranges because summers there are not necessarily hotter or cooler nor winters colder or milder than those at places well within the range of P . racemosa .
A possibly significant factor limiting the capacity of sycamores to invade the northern Coast Ranges is that region's cool, wet spring. The collective mean precipitation for May is 43 mm. among the climatic stations of both the northern Coast Ranges and the Sacramento Valley, but that month is 1ºC warmer at the Valley sta-
[3] A high Mg/Ca ratio may be important in these streams as well since many have much serpentinite in their watersheds. This ratio is 1.6 in Cache Creek, which has many tributaries which have always lacked riparian trees. The Mg/Ca ratio, electrical conductivity, and dissolved Na are, respectively, 87%, 628%, and 975% greater in Cache Creek than in the Sacramento River into which it flows.
tions. In the southern Coast Ranges the collective May mean temperature is also 1°C warmer than in the northern Coast Ranges, but the collective mean precipitation for that month is 28 mm. less (US Department of Commerce 1970). Anthracnose (Gnomoniaplatani ) is a very serious and prevalent disease which can cause complete spring defoliation of Platanus species including P . racemosa , and it is known to be promoted by cool and wet spring weather (Fowells 1965; Collingwood etal . 1974; Pirone 1970). This fungus is currently severely stressing wild populations of P . racemosa in Alameda and Contra Costa Counties at the northwestern limit of the range of that species, and it must be suspected of limiting the further expansion of sycamores northwestward in California.
Populus (Cottonwood)
Populus is the most important riparian genus in California, and one of the two major genera of the Salicaceae, probably the most important riparian family in the world. This family was traditionally placed close to the Hamamelidales and Fagales in the artificial taxon Amentiferae because of its unisexual catkins, but it is now recognized to be misplaced there. Its characters, which include capsular fruit with many seeds, suggest a much closer relationship to the small, largely halophytic Tamaricales and to the large and diverse assemblage of plants variously known as the Violales or Cistales. It is distinctive enough, however, to be retained in its own monotypic order, the Salicales (Cronquist 1968; Dahlgren 1975; Thorne 1976).
The Salicaceae, which include the large and widespread genus Salix and the monotypic East Asian Chosenia in addition to Populus , are deciduous woody plants which are dioecious and have very light and easily wind dispersed comose seeds. They share the pattern seen in the Betulaceae of adaptation to riparian zones in temperate climates (as well as tropical in the case of Salix ), with much wider extension into upland habitats in boreal, montane, and arctic climates where late snowmelt saturates the soil during part or all of the growing season. Unlike Alnus , Betula , and Salix , however, Populus consists entirely of trees. Unlike Salix , which is secondarily entomophilous (Thorne 1976), it is entirely wind pollinated.
Populus consists of 35 species and ranges throughout the North Temperate Zone into parts of the Arctic. Four species (P . tremuloides , P . trichocarpa , P . fremontii , and P . angustifolia ) are native to California. P . tremuloides (aspen) is a largely upland species which is widespread in the boreal and montane parts of North Amnerica, and extends south to some of the higher California mountains. P . angustifolia is a riparian species of the Rocky Mountains, which occurs in a few colonies in the area east of the southern Sierra Nevada crest where summer thunderstorms of tropical origin also permit larger but still relict populations of Betulaoccidentalis to survive.
P . tremuloides is the most taxonomically distinctive Populus species in California, and is set off from the others in section Leuce . P . trichocarpa and P . angustifolia are close enough to share placement in section Tacamahaca , but even though P . fremontii is set off from these species in section Aegeiros (Rehder 1940), it can hybridize with P . trichocarpa (Little 1953). Such hybrids seem to be rare in California, but hybrids between P . angustifolia of Tacamahaca and P . sargentii of Aegeiros are abundant in the Great Plains (Elias 1980).
P . fremontii (Fremont cottonwood) and P . trichocarpa (black cottonwood) are the two principal riparian species of Populus in California, and P . fremontii is the single most important riparian species in the state since it dominates the great riparian forests of the Central Valley (Conard etal . 1977) as well as many of those elsewhere in cismontane and transmontane California (Roberts etal . 1977). Most of these magnificent forests have been destroyed (Thompson 1977), but small good examples have been preserved by the Nature Conservancy on the Kern River, by the California Department of Parks and Recreation at Caswell State Park, and by a few other groups and agencies elsewhere. Because of their great ecological significance (Sands 1977; Hehnke and Stone 1978) every effort should be made to preserve what remains of these rapidly vanishing natural communities. Particular attention should be given to those in the Sacramento and lower San Joaquin Valleys, where preservation opportunities are greatest, and to what remains of those along the Colorado River, where destruction has been most complete.
P . trichocarpa is one of the largest broad-leaved trees in North America, but it is a less conspicuous riparian tree than P . fremontii in California because it is most common in the cooler, wetter parts of the state, where it frequently associates with highly competitive riparian species such as Alnusrhombifolia , and often grows near upland forests dominated by giant conifers. Magnificent riparian forests dominated by P . trichocarpa do occur in coastal and montane California, however, and a fine example remains along the Carmel River in Monterey County.
The California ranges of P . trichocarpa and P . fremontii overlap (Griffin and Critchfield 1972), and individuals of the two species frequently grow sympatrically without hybridization. P . trichocarpa is essentially limited to those parts of California with July mean temperatures cooler than 25°C, while California populations of P . fremontii grow at sites with a range of July means approximately between 17°C and 36°C. The cooler P . fremontii sites are limited to coastal areas around San Francisco Bay and from San Luis Obispo County south where winters are mild and the growing season long.
The total ranges of these species and their closest relatives outside California (fig. 8 and 9) reflect a pattern similar to that of their ecological relationships in the state. P . trichocarpa is common throughout the cool, wet Pacific Northwest north to southern Alaska and east to the northern Rocky Mountains (Little 1971), and its closest North American relatives are P . angustifolia of the Rocky Mountains and P . balsamifera , a frequently upland species which is widespread in the continent's boreal regions (Rehder 1940). P . trichocarpa is considered conspecific with the latter by Eckenwalder (1980).
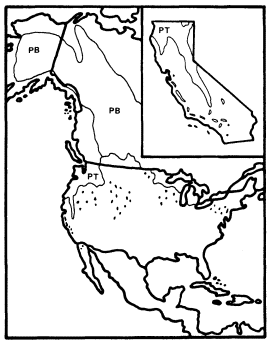
Figure 8.
Ranges of P . trichocarpa (PT) and
P . balsamifera (PB) (after Little 1971).

Figure 9.
Ranges of Populus fremontii (PF), P . deltoides
(PD), and P . sargentii (PS) (after Little 1971).
P . fremontii , in contrast, is found east to Trans-Pecos Texas in riparian sites throughout the Southwest, and it is most closely related to the riparian species P . arizonica of the Southwest, P . deltoides and P . sargentii of warm temperate eastern North America, and P . nigra of warm temperate Europe (Axelrod 1975). Macrofossils intermediate between P . fremontii and P . deltoides are known from the then warmer and wetter Miocene Ellensburg Flora of Washington, where neither species occurs today (Robichaux 1977).
These relationships suggest that both P . trichocarpa and P . fremontii are western derivatives of formerly transcontinental ArctoTertiary entities within Populus . P . trichocarpa and its relatives within section Tacamahaca are derivatives of a cool temperate to boreal species complex, and P . fremontii and the other species of section Aegeiros are of warm temperate ancestry.
Quercus (Oak)
This huge genus of 450 species dominates the upland deciduous forests of the North Temperate Zone, but it also includes many riparian, shrubby, and evergreen species, and extends south through the American tropics as far as the Colombian Andes. Quercus is in the Fagaceae, which it shares with several other major tree genera, and thus in the Fagales, which it shares with the Betulaceae, Corylaceae, and Carpinaceae (Willis and Airy Shaw 1973).
Quercus and other Fagaceae are wind pollinated and frequently deciduous like the Betulaceae, but their heavy, nutrient-rich fruits, like those of Juglans , are poorly dispersable and better adapted to germination in mature forests than in the regularly disturbed riparian zones to which the light, easily wind dispersed fruits of Alnus and Betula are well adapted (Grime 1979).
The 16 California species of Quercus include most of the range of morphological diversity in the genus, and all of its three subgenera (Quercus , Erythrobalanus , and Protobalanus ), but Q . lobata (valley oak) is the state's only major riparian oak. The other species are primarily or exclusively upland trees and shrubs, and even Q . lobata is somewhat more common in upland than in riparian zones. In the upland oak woodlands of the Coast Ranges it is common in sites which have heavy, poorly aerated soils with high water-holding capacity. Such ecological conditions are similar to those of the riparian sites where it occurs since these tend to be both drier and less well aerated than sites dominated by the other principal riparian trees.
In addition to its occasional dominance of upland oak woodland in the Coast Ranges when ecological conditions are suitable, Q . lobata can dominate two kinds of riparian communities: 1) riparian forest adjacent to streams when aeration is too poor for Populusfremontii , the typical riparian forest dominant. Q . lobata can form gallery forests in a matrix of treeless grassland in such situations, which were once common in the area of excessively heavy soil in the Sacramento Valley where rice is now extensively grown. The grasslands have almost entirely been converted to rice fields in this area, and many gallery forests of Q . lobata lost as well, but a fine example is still extant along Honcut Creek in northern Yuba County; 2) forest, woodland, and savanna on alluvial plains and terraces, usually above and toward the upland edge of typical riparian forest dominated by Populus fremontii (Conard etal . 1977). In these communities oaks must have access to water throughout the growing season, and their field relationships suggest that the accessibility of their water supply determines their density. Closed forests overwhelmingly dominated by Q . lobata can occur where water is abundant at relatively shallow depths, but progressively more open woodland and savanna communities in which oaks are scattered in a matrix of grassland are found as water apparently becomes more limiting. One of the very few field studies of the water relations of California oaks was done at such a Q . lobata community in Monterey County. It suggested that while Q . lobata had access to a reliable water table, Q . douglasii of adjacent uplands probably did not (Griffin 1973). However, since Q . lobata can also occur on upland sites similar to those of Q . douglasii (Griffin 1977), it should not be assumed that all its populations have the ready access to groundwater of its alluvial communities.
California's alluvial Q . lobata communities were once fairly common in parts of the Central Valley and in many Coast Ranges valleys as well, but since they were indicators of some of the world's best agricultural soils, most have long since been converted to farmland (Rossi 1980). A very few small but good examples of California's alluvial Q . lobata forests are still extant in Mendocino, Butte, Yolo, and Sacramento Counties, and perhaps elsewhere. Every effort should be made to permanently preserve them while the opportunity still exists.
All oaks need water, and several other upland species share some of Q . lobata's tolerance of poor soil aeration, so it is not surprising that they occasionally dominate riparian communities as well. Riparian Q . douglasii occurs along Mitchell Creek in Contra Costa County; riparian Q . engelmannii is found along Pala and other similar creeks in San Diego County; and Q . agrifolia forms a fine but very unusual riparian forest along the lower Mokelumne River on the floor of the Central Valley. Since the latter species is ordinarily restricted to the Coast Ranges and southern California, it must be assumed that marine airflow through the Carquinez Straits permits its survival in this part of the lower San Joaquin Valley. It is hoped that this rare and unstudied natural community will also soon receive adequate protection.
Q . lobata is a distinctive species endemic to California (fig. 10) of subgenus Quercus , the only one which extends to Eurasia (Tucker 1980). This subgenus is quite diverse and includes many large deciduous and evergreen trees as well as a number of evergreen shrubs in the Southwest and northern Mexico, but Q . lobata does not seem to be particularly closely related to any of these. As a large deciduous tree it is superficially similar to Q . garryana , but it stands somewhat apart from the series of increasingly drought-adapted species which Q . garryana forms with Q . douglasii and Q . engelmannii . It can occasionally hybridize with each of these, however. Surprisingly, it naturally hybridizes most abundantly with the shrub Q . dumosa (ibid .), and it is interesting but perhaps not significant that several other small white oaks of the Southwest have similarly elongated acorns with shallow cups. Since it is not particularly close to any of the eastern North American white oaks either, it is perhaps best to view Q . lobata as a distinctive derivative of subgenus Quercus which shares a complex pattern of interrelationships with other species of that taxon, and which has been a recognizable entity at least since the Miocene, when it was more widespread in western North America (Robichaux 1977).
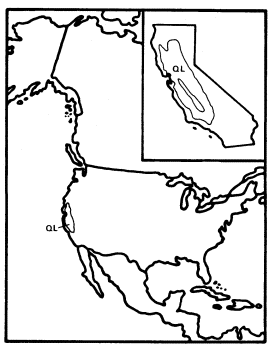
Figure 10.
Range of Quercus lobata (QL) (after Little 1971).
Salix (Willow)
Salix , the second major genus of the Salicaceae and Salicales, consists of about 500 species of trees and shrubs. The trees are very important components of riparian communities of the North Temperate Zone, and have invaded similar habitats in the tropics and South Temperate Zone as well, but the shrubs are largely restricted to the Northern Hemisphere and tend to be smaller and more associated with upland zones as high latitudes and altitudes are approached, a pattern of adaptation similar to but more welldeveloped than that seen in Alnus and Betula . Willows are typically deciduous and share the dioecious catkins and easily dispersed comose seeds of other Salicaceae, but they are usually secondarily entomophilous (Thorne 1976), and can be almost evergreen in the tropics (e.g., S . bonplandiana ).
California has about 32 Salix species (Munz 1959, 1968); the exact number is uncertain because of the difficult and controversial taxonomy of the genus. Which of these species should be considered trees is almost as controversial since the state's willow species are a continuum between large trees and dwarf shrubs, and several of the species which are usually shrubs can develop into small trees when conditions are favorable. The California willows which most frequently reach tree size are S . exigua , gooddingii , hindsiana , hookeriana , laevigata , lasiandra , lasiolepis , rigida (mackenziana ), scouleriana , sitchensis (coulteri ), and tracyi . All of these are riparian, but S . hookeriana , rigida , scouleriana , and tracyi are small local or northern species largely limited to riparian zones within montane or northwest coast coniferous forests, and are of little importance in the riparian communities of the rest of California.
Salixgooddingii is the most important willow of the great riparian forests of California's Central Valley, and it frequently shares dominance there with Populus fremontii , particularly at intermediate successional stages. It is a better pioneer than P . fremontii , if not as good as S . hindsiana , and it usually dominates the new riparian forests which often form in the Valley along neglected ditches and canals. Since it is generally more weedy and tolerant of stress than P . fremontii as long as water is abundantly available, it is particulaly important in the depauperate riparian forests along the lower San Joaquin River, where high salinity and poor development of natural levees have probably long limited maximal riparian community development (Kahrl 1979). S . gooddingii is limited to riparian zones of the Central Valley, southern California, and the desert Southwest (Little 1976; Elias 1980), a distribution which suggests a need for long, hot growing seasons as well as abundant groundwater (fig. 11). The relationships of this species are not in doubt since it is so similar to S . nigra , the most important large willow of eastern North America, that it is still some-times included within it as S . nigra var. vallicola (Little 1953, 1976).
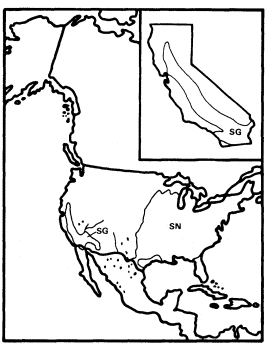
Figure 11.
Ranges of Salix gooddingii (SG) and
S. nigra (SN) (after Little 1971, 1976).
S . lasiandra and S . laevigata are similar enough to one another that Hoover (1970) doubted their distinctness. Their ecological niche seems similar as well since both are large riparian willows which grow along streams in the Coast Ranges and the lower foothills of the Sierra Nevada. S . laevigata is reported to prefer well-aerated, rapidly flowing streams (Elias 1980), and it has been mapped as absent from most of the Central Valley by Little (1976), who also excludes S . lasiandra from most of the San Joaquin Valley. Conard etal . (1977) and Roberts etal . (1977) report both species to be common components of Central Valley riparian forests, however. What is clear about these two species at this time is: 1) they are more common along streams in the highlands surrounding the Valley than along the Valley floor; 2) they occur on the Valley floor; and 3) the details of their distribution and ecological relationships in California need to be much better understood. S . lasiandra may have less tolerance for habitats along intermittent streams than S . laevigata and thus may have a greater need for permanent water, but this observation needs verification.
The total ranges of these species do suggest that S . laevigata may be the more drought-adapted of the two since S . lasiandra extends down the mountains and coast of California from a wide range in the cool and wet parts of the Pacific Northwest, the northern Rocky Mountains, western Canada, and central Alaska while S . laevigata is restricted to mediterranean Cali-
fornia and a few relict stations in Arizona, Nevada, and Utah (fig 12 and 13) (Little 1976). S . lasiandra is reported to be closely related to S . lucida of the boreal forests of eastern North America (Rehder 1940), and S . laevigata is similarly reported to be related to S . bonplandiana , a semi-evergreen species of southern Arizona and tropical western Mexico (Elias 1980), but a critical reexamination of their relationships to each other and to other species which they resemble would be desirable.

Figure 12.
Ranges of Salix lasiandra (SL) and
S . lucida (SLU) (after Little 1976).
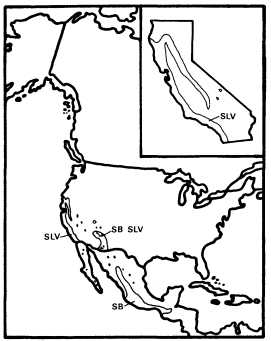
Figure 13.
Ranges of Salix laevigata (SLV) and
S. bonplandiana (SB) (after Little 1976).
S . hindsiana and S . exigua (fig. 14) are largely shrubs, but they are both major components of California riparian vegetation because they are usually the first woody plants to colonize sandbars and other newly-formed riparian habitats when these are relatively fine-grained and shallow to groundwater. The dominance of such sites by these willows produces a distinctive and common riparian shrub community (Conard etal . 1977), but Baccharisviminea and B . glutinosa can become more important when alluvium is coarser and the water table deeper.
S . hindsiana is largely cismontane and endemic to the California Floristic Province while S . exigua is mostly transmontane in California and widespread in the rest of North America (fig. 14) (Little 1976). Both are ecologically and morphologically similar, however, and difficult to distinguish when sympatric (Hoover 1970; Smith 1970). They are part of a complex of closely related and ecologically similar western willows which also includes S . fluviatilis , S . sessilifolia , and S . melanopsis .
S . lasiolepis is a common small willow of much of the California Floristic Province, and it also occurs at scattered, possibly relict stations throughout the West (fig. 15) (Little 1976). It becomes an important vegetation component in the fog belt of the California coast, however, because there it dominates a distinctive forest community on alluvial bottomlands and in dune slacks. Myricacalifornica is an important associate in these forests, and a rare thistle, Cirsium loncholepis , is entirely restricted to them. Most of this apparently previously undescribed community was lost early
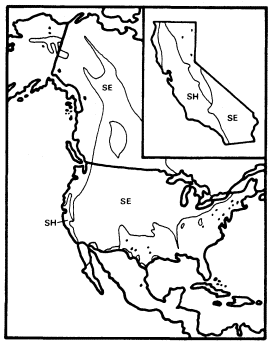
Figure 14.
Ranges of Salix hindsiana (SH)
and S . exigua (SE) (after Little 1976).
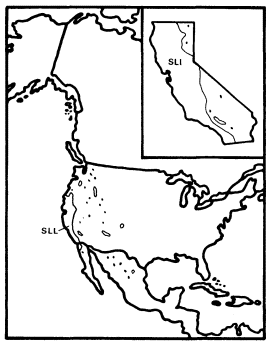
Figure 15.
Range of Salix lasiolepis (SLL) (after Little 1976).
to agriculture since it frequently dominated wide coastal plains in the Arroyo Grande, Oso Flaco, and Santa Maria Valleys, which are now rich vegetable districts. A small remnant of it is now protected at Pismo State Beach in San Luis Obispo County, and a larger stand, which includes critical habitat of the endangered Unarmored Threespine Stickleback (Gasterosteusaculeatus williamsoni ), could be saved along San Antonio Creek in Santa Barbara County.
S . lasiolepis is closely related to S . hookeriana and S . tracyi (Elias 1980), two small trees of north coastal riparian communities, and probably to a number of northern and montane shrubs as well. It is less close to S . sitchensis , a small tree which also grows along fog belt streams as far south as Santa Barbara County (Smith 1976) but seldom forms extensive forests.
Riparian Origins
When the Arcto-Tertiary Geoflora was decimated throughout western North America in the Late Tertiary and Quaternary by spreading drought and cold, it is clear that some of its elements which were already strongly adapted to riparian conditions were able to survive along California's permanent and intermittent streams (Axelrod 1977). Their descendents dominate the state's riparian forests today. Some Arcto-Tertiary elements which were weakly adapted to riparian conditions such as Juglans hindsii were also able to precariously survive to the present in riparian refuges. Riparian forests are not among California's major refuges for Arcto-Tertiary and other relicts, however (Stebbins and Major 1965). This is partly because most riparian taxa are still too successful to be considered relictual but primarily because riparian environments are too competitive and frequently disturbed to promote high plant species richness and thus the survival of many marginal, relict species. Grime (1979) has shown that plant species density is highest in environments of intermediate productivity in which neither competition nor stress are excessive.
Before California streams were dammed, floods periodically disturbed riparian communities, renewing nutrients and understory light in an environment where warmth and soil moisture were already ideal for maximal productivity. Such conditions of regular but relatively infrequent disturbance when resources are not limiting are conducive to maximum plant competition and thus low species richness (ibid .). In contrast, California's concentrations of relictual species are in areas of intermediate productivity where plant species density is not limited by extremes of either stress or competition.
Riparian Dominance and Environment
Populusfremontii is the most common dominant of central California's riparian forests, but as discussed previously, environmental factors can shift dominance to other species. The most obvious of these factors are frequency of disturbance, air temperature, root zone aeration, and depth to groundwater, but others are probably important as well. It has been shown that when disturbance is high, dominance is shifted to Salix hindsiana and when somewhat less severe to S . gooddingii . Cool growing seasons favor P . trichocarpa , and hot environments sites with ahigh water table and low root aeration promote Cephalanthus occidentalis , but when turbulent, well-aerated water is close to the surface, Alnusrhombifolia can become dominant. When water tables are relatively deep, Platanusracemosa is the usual dominant when aeration of the intervening soil is high and Quercuslobata when it is low.
Q . lobata , the only major California riparian tree which is probably not of riparian origin, is most frequently dominant in riparian systems when two stressing factors, deep groundwater and low soil oxygen, are both present. This suggests that it is a relatively more stress-tolerant competitor than other riparian dominants. It is interesting that this species is probably derived from drought-stressed upland climax forests and woodlands, where trees with the stress-tolerant competitor adaptive strategy would be expected to dominate, rather than from riparian communities, which are more frequently dominated by trees with a simply competitive strategy (Grime 1979).
Deciduousness and Productivity
Mediterranean-type climates, with their mild, wet winters and dry summers, are well known for their sclerophyllous evergreen vegetation (Mooney etal . 1977; Cody and Mooney 1978; Walter 1979), but the presence of seemingly anomalous winter-deciduous riparian vegetation well within such a climate in California has generated relatively little comment. Air temperature obviously does not exclude evergreens from the Central Valley since they are common there in non-riparian zones. This led Stebbins (1974) to suggest that winter-deciduousness is favored when a highly productive growing season alternates with a cool season which is much less favorable but not necessarily extremely cold. He further postulated that it arose in riparian zones because wet roots aggravate winter cold stress and thus deciduousness.
The first hypothesis is undoubtedly correct, but the second is unlikely since evergreen woody angiosperms are more common in riparian than in upland zones on the Atlantic Coastal Plain in South Carolina, where summers are hot, wet, and highly productive, and winters are about as cold as those of the Central Valley. Evergreen angiosperm trees common in some riparian zones on the South Carolina Coastal Plain include Gordonia lasianthus , Ilexcassine , I . coriacea , I . myrtifolia , I . opaca , I . vomitoria , Magnolia virginiana , Myricacerifera , M . heterophylla , Perseaborbonia , P . palustris , and Quercuslaurifolia (Elias 1980).
In January Columbia, South Carolina, at the inner edge of the coastal plain, has a mean temperature of 7°C and a mean minimum temperature of 1°C. Sacramento in January has the same mean temperature and a mean minimum of 3°C. Despite the similarity of these temperatures, however, Sacramento has a mean frost-free growing season of 307 days and Columbia of only 248. Charleston, on the coast at the outer edge of the coastal plain, has milder winters than Columbia or Sacramento since it has a January mean of 9°C and mean minimum of 3°C, but even here the mean frost-free growing season is only 285 days, fully 22 days shorter than at Sacramento (US Department of Agriculture 1941).
The shorter growing season and greater spread between winter mean and mean minimum temperatures of these South Carolina cities relative to Sacramento are caused by the much more frequent penetration of outbreaks of cold arctic air to the coastal plain than to the Central Valley, which is usually protected from them by the Rocky Mountains and the Sierra Nevada and Cascade Ranges. The riparian evergreens of South Carolina must cope with these regular cold outbreaks as well as with their mean winter climate, so it is unlikely that the winter-deciduousness of the California riparian community is a general phenomenon which occurs wherever winter cold and wet soil interact.
Rundel (1980), in a comparative study of the adaptive strategies of mediterranean-climate oaks, supported Stebbins' view that winter-deciduousness is favored when highly productive summers alternate with winters which are much less productive but not necessarily highly stressful. Rundel assembled evidence which showed that deciduous oak leaves are considerably more photosynthetically efficient than those of evergreen oak species. For this extra efficiency to be profitable, however, a growing season which is sufficiently long, warm, and stress-free must be predictably present to compensate for the energetic cost of producing a new crop of leaves each year.
There is every reason to believe that Rundel's generalizations about the adaptive strategies of oaks apply equally well to those of other taxa. Deciduousness is clearly an example of the ability to rapidly respond to environmental change, a characteristic which Grime (1979) considered central to the competitive adaptive strategy. As discussed above, riparian environments, with their high productivity, minimal stress, and regular but infrequent disturbance, provide just the conditions which Grime predicted would most favor this strategy.
Major (1963) has shown that the activity of upland plant communities can be estimated from readily obtainable climatic data by using Thornthwaite's water balance concept (Thornthwaite 1948; Thornthwaite and Mather 1955). Thornthwaite's potential evapotranspiration (PE) is a function of temperature and day length and thus of warmth and light. His actual evapotranspiration (AE) predicts the evapotranspiration which can take place at a site if no more water is available to it than that received from precipitation. Since AE is a function of warmth, light, and precipitation (as well as soil water storage) and these are three of the most essential ingredients of plant productivity, Rosenzweig (1968) pointed out that AE can provide a reasonably good estimate of this as well.
In mediterranean-type climates AE is depressed far below PE because lack of summer rain causes severe drought stress and suppresses plant productivity just at the time when warmth and light are maximal. At Sacramento, for example, in the heart of California's mediterranean-type climate, annual PE is 815 mm. but annual AE is only 458 mm. (Mather etal . 1964). AE and PE are both 15 mm. in January, but while PE climbs as high as 140 mm. in July, AE peaks at 72 mm. in May and then declines because of summer drought. If less soil moisture storage were assumed than the 300 mm. used to calculate these figures, AE would peak even earlier and its annual sum would be even less.
Grassland is the dominant upland vegetation type at Sacramento. Upland broad-leaved trees are sparse and consist almost entirely of two species, evergreen sclerophyllous Quercus wislizenii and deciduous but semi-sclerophyllous Q . douglasii .
At Columbia, South Carolina, however, summer rain is abundant, and the annual AE of 915 mm. almost matches the annual PE of 952 mm. Both AE and PE are 13 mm. in January, and both rise to similar peaks in July, when PE is 172 mm. and AE is 162 mm. Columbia is surrounded by a rich upland forest which can be dominated by pines or by several species of deciduous broad-leaved trees.
When rivers flowing from distant mountains import far more water to an area than would be available from local precipitation, keeping soil saturated at shallow depths throughout the year, AE, in effect, becomes equal to PE. This is exactly what happens in the riparian forests of California's Central Valley. As a result their defacto AE, their activity cycle, their productivity, and even their physiognomy are all very similar to those of the upland deciduous forests of South Carolina's coastal plain.
It can be seen that a gigantic natural experiment has confirmed Stebbins' and Rundel's hypothesis. Deciduousness is promoted wherever a long, very productive growing season is paired with a minimally productive but not necessarily very stressful cool or cold season. Like human beings with high incomes and low expenditures, deciduous trees can afford to rest during the season when their income would otherwise be lowest. The poorer evergreens do not have this luxury.
Quercuskelloggii (black oak) is found from San Diego County to central Oregon and is one of California's most abundant broad-leaved trees (Bolsinger 1980). Since Q . kelloggii is a non-riparian tree with deciduous, nonsclerophyllous leaves, theory predicts that it should occur where the local climate promotes maximal productivity during a well-defined growing season. Table 1 validates this prediction. Maximum monthly AE, and thus seasonal productivity, is considerably higher within the black oak's California range than in those parts of the state where it does not occur.
|
AE is high within the range of Q . kelloggii because this species is limited to mountain slopes too low and too far inland to have cool summers but of relief sufficient for high orographic precipitation. Summers are dry, but where soil storage is good, surplus water from winter and early spring rains can promote high productivity during the warm days of late spring and early summer and thus favor this upland tree's deciduous habit.
Mineral Nutrition
Monk (1966) found that forests in north-central Florida are much more likely to be dominated by evergreen than deciduous trees when mineral nutrients and pH are low, and he generalized that relative soil sterility promotes evergreenness. Rundel (1980) also noted that evergreen leaves are more nutrient-use efficient. It is clear that nutrient losses from the regular shedding of deciduous leaves represent a cost which can only be made up during a long and productive growing season in a reasonably fertile environment. Since nutrient deficiencies lower productivity (Kramer and Kozlowski 1979) they, in effect, lower AE and promote evergreenness indirectly as well as directly.
California riparian forests receive imported nutrients as well as water from their rivers and streams (or did before dams stopped floods and became nutrient traps). Thus their productivity and deciduousness are doubly promoted. It is interesting that the Sacramento and the Stanislaus, the two Central Valley rivers with the most limestone in their watersheds and thus the most calcium, are also those with the best developed riparian forests along the banks of their lower reaches.
It was noted earlier, however, that some riparian communities on the Atlantic Coastal Plain are evergreen while surrounding upland communities are deciduous. These riparian communities occur because the low relief of the Coastal Plain does not permit rapid drainage of the heavy precipitation which it receives. They are nutrient-poor because their water is of local, meteorological origin, and can only leach and carry away mineral nutrients, not deposit them (Wharton and Brinson 1979). In this climate, where abundant summer rain makes AE maximal, the water available to riparian communities can not raise their productivity much above that of adjacent upland vegetation. It can only lower it through leaching. The result is evergreen vegetation in riparian communities on the Coastal Plain when the water is of local origin, but deciduous riparian forests dominated by species of Salix , Populus , Quercus , Platanus , and Fraxinus similar to those of California occur along the major rivers. These flow out of the Appalachians and import nutrients to the Coastal Plain to replace those lost by local leaching (Braun 1950; Hosner 1962; Wharton and Brinson 1979).
Productivity and Community Ecology
California's riparian communities are its most productive because they receive abundant water during hot, cloudless summers which are
ideal for maximum photosynthesis. Their high PE thus becomes a high AE. Everywhere else in California except on the highest mountain summits summer drought suppresses AE below PE and plant productivity below its potential. Outside of alpine areas AE approaches PE most closely among California's upland communities in the coastal redwood belt of Del Norte and northwestern Humboldt Counties, but summers are too cool and cloudy there for productivity and either AE or PE to be extremely high. Crescent City, in the center of this area, has an AE of 597 mm. and a PE of 650 mm. while Davis, in the Central Valley, has an upland AE of 420 mm. and a PE and thus riparian AE of 810 mm. (300 mm. soil storage assumed).[4]
The riparian systems of California are clearly far more productive than any of that state's communities which are dependent on their local climate can be. Maximal riparian productivity more closely approaches that of eastern deciduous forests in summer and of tropical rain forests throughout the year. It is not surprising, then, that California's riparian forests share some features with exotic ecosystems which are absent, rare, or poorly developed elsewhere in the state.
Herbs with tropical affinities, such as Hibiscus californicus and Fimbristylisvahlii , occur in California riparian communities, and riparian Vitis californica is a well-developed liana, a growth form 90% confined to tropical forests (Walter 1979) and very poorly developed in upland California. Abundant warmth and water are apparently essential to large lianas, and the inability to tolerate even a brief winter rest period is probably what confines most taxa to the tropics. Notoriously high root pressures have undoubtedly helped Vitis cope with this problem and survive in seasonally highly productive temperate habitats like California riparian zones (Kramer and Kozlowski 1979).
Despite their small overall area California's riparian forests are especially well known for the abundance and diversity of their bird fauna (Small 1974; Gaines 1977). Their breeding avifauna is particularly important because it includes many species which occur in virtually no other California habitat. Gaines (ibid .) has shown that these birds are very frequently insectivorous foliage gleaners which winter in tropical forests and have vicariant populations in eastern deciduous forests, two habitats which share the high productivity of western riparian communities.
Insects, as poikilotherms which are largely primary consumers, are expected to increase in abundance with increasing warmth and primary productivity, and in California upland vegetation insect biomass does, in fact, peak in spring and closely fluctuate with primary productivity throughout the year (Cody etal . 1977). Comparable data do not seem to be available for any California riparian community, but the extremely high summer productivity of such communities undoubtedly induces similarly high summer peaks of insect biomass. These in turn act as magnets for insectivorous migratory birds. Gaines (1977) was ambivalent about this since at more than one point he noted the connection between riparian insect and insectivorous bird abundance but also yielded to Willson's (1974) view that bird density is not dependent on habitat productivity. Willson reached this conclusion after field work in what were apparently mostly various successional stages of upland forest and woodland vegetation within a single local climate in east central Illinois. Her studies showed that avian biomass was similar at all these successional stages and bird density greatest at intermediate ones. Willson concluded that her data showed these parameters of bird populations are unrelated to either plant or insect productivity since she assumed plant productivity is highest in early successional stages and insect productivity highest in late stages. Her first assumption ignored the very important stem and root components of primary productivity, however, and the second was the result of a literature review which, in effect, assumed that vegetation in different climates—and thus productivity regimes—can represent stages of and thus show the ecological effects of a single successional sequence. Her data do not support her conclusion that bird populations are unrelated to community productivity, but they do suggest that bird density may be highest at intermediate successional stages. These are just the successional stages when primary productivity is greatest (Larcher 1975).
Bird abundance does appear to be positively related to community productivity, and riparian bird populations can be expected to be augmented most relative to those of upland habitats when contrasts between upland and riparian productitivity are greatest. Such contrasts occur whenever perennial streams reliably bring water to arid or semiarid lands. In deserts with summer rainy seasons, however, vegetation along ephemeral streams which merely carry away the floodwaters of rare storms may actually be less productive of plants and animals than nearby uplands which are less disturbed and no drier (Wauer 1978). The biotic effects of riparian environments are much less conspicuous in areas such as the Atlantic Coastal Plain where upland vegetation is highly productive, but even here the variety of drainage conditions typical of riparian zones produces edaphic diversity and resultant habitat and species diversity. (Hosner 1962; Hair etal . 1974).
Bird populations are not simply a function of primary productivity, however, since highly productive irrigated cropland supports bird populations which are less diverse and frequently less abundant than those of natural riparian
[4] Major, J. No date. Water balances for California climatic stations. Unpublished manuscript.
communities within the same local climate (Hehnke and Stone 1978). MacArthur and MacArthur (1961) demonstrated that bird species diversity is greatest in tall, highly stratified vegetation, and this can only occur when community phytomass is high. High phytomass is not universal in productive environments, but it is limited to them (Walter 1978).
It is tragic that the rich bird fauna of California's riparian communities has declined drastically within just the last few decades. Gaines (1977) cited reports which attribute this decline to brood parasitism by the recently introduced Brown-headed Cowbird, but he also noted that its introduction to Arizona occurred long before a similar decline in the riparian avifauna there. The cowbird has been especially frequently linked to the virtual extirpation of the riparian and insectivorous Bell's Vireo from California and Arizona, and yet these two species coexist in abundance in the less agricultural Rio Grande Valley of Texas (Wauer 1977). The riparian communities of Califorina and Arizona are frequently surrounded by agricultural areas where massive quantities of insecticides are used, but there seems to have been little comment about or investigation of their potential impact on a largely insectivorous riparian avifauna.
Literature Cited
Axelrod, D. 1960. The evolution of flowering plants. p. 227–305. In : S. Tax (ed.). Evolution after Darwin. Volume 1: the evolution of life. 629 p. University of Chicago Press, Chicago, Ill.
Axelrod, D. 1973. History of the mediterranean ecosystem in California. p. 225–277. In : F. diCastri and H. Mooney (ed.). Mediterranean type ecosystems: origin and structure. 405 p. Springer Verlag, New York, N.Y.
Axelrod, D. 1975. Evolution and biogeography of Madrean-Tethyan sclerophyll vegetation. Ann. Missouri Bot. Garden 62:280–334.
Axelrod, D. 1977. Outline history of California vegetation. p. 139–193. In : M. Barbour and J. Major (ed.). Terestrial vegetation of California. 1002 p. John Wiley, New York, N.Y.
Bolsinger, C. 1980. Oaks in California's commercial forests—volume, stand structure, and defect characteristics. p. 101–106. In : T. Plumb (tech. coord.). Proceedings of the symposium on the ecology, management, and utilization of California oaks. [Claremont, Calif., June 26–28, 1979]. USDA Forest Service GTR-PSW-44. 368 p. Berkeley, California.
Braun, L. 1950. Deciduous forests of eastern North America. 596 p. Hafner, New York, N.Y.
Chabot, B., and W. Billings. 1974. Origins and ecology of the Sierran alpine flora and vegetation. Ecol. Monog. 42:163–199.
Cody, M., E. Fuentes, W. Glanz, J. Hunt, and A. Moldenke. 1977. Convergent evolution in the consumer organisms of mediterranean Chile and California. p. 144–192. In : H. Mooney (ed.). Convergent evolution in Chile and California mediterranean climate ecosystems. 224 p. Dowden, Hutchinson & Ross, Stroudsburg, Penn.
Cody, M., and H. Mooney. 1978. Convergence versus nonconvergence in mediterranean climate ecosystems. Ann. Rev. of Ecol. and Syst. 9:265–321.
Collingwood, G., W. Brush, and D. Butcher. 1974. Knowing your trees. 374 p. American Forestry Association, Washington, D.C.
Conard, S., R. MacDonald, and R. Holland. 1977. Riparian vegetation and flora of the Sacramento Valley. p. 47–55. In : A. Sands (ed.). Riparian forests in California: their ecology and conservation. Institute of Ecology Pub. No. 15. 122 p. University of California, Davis.
Cronquist, A. 1968. The evolution and classification of flowering plants. 396 p. Houghton Mifflin, Boston, Mass.
Dahlgren, R. 1975. A system of classification of the angiosperms to be used to demonstrate the distribution of characters. Bot. Notiser. 128:119–147.
Eckenwalder, J. 1980. Populus . p. 420–421. In : J. Kartesz and R. Kartesz (ed.). A synonymized checklist of the vascular flora of the United States, Canada, and Greenland. Volume II: the biota of North America. 500 p. University of North Carolina Press, Chapel Hill, N.C.
Elias, T. 1980. The complete trees of North America: field guide and natural history. 948 p. Van Nostrand Reinhold, New York, N.Y.
Engler, A., and H. Melchior. 1964. Syllabus der pflanzenfamilien, Band II. 666 p. Gebruder Borntraeger, Berlin-Nikolassee.
Fowells, H. 1965. Silvics of forest trees of the United States. USDA Forest Service Agriculture Handbook No. 271. 762 p.
Gaines, D. 1977. The valley riparian forests of California: their importance to bird populations. p. 57–85. In : A. Sands (ed.). Riparian forests in California: their ecology and conservation. Institute of Ecology Pub. No. 15. 122 p. University of California, Davis.
Griffin, J. 1973. Xylem sap tension in three woodland oaks of central California. Ecology 54:152–159.
Griffin, J. 1977. Oak woodland. p. 383–415. In : M. Barbour and J. Major (ed.). Terestrial vegetation of California. 1002 p. John Wiley, New York, N.Y.
Griffin, J., and W. Critchfield. 1972. The distribution of forest trees in California. USDA Forest Service Research Paper PSW-82. 114 p.
Grime, J. 1979. Plant strategies and vegetation processes. 222 p. John Wiley, New York, N.Y.
Hair, J., G. Hepp, L. Luekett, K. Reese, and D. Woodward. 1979. Beaver pond ecosystems and their relationships to multi-use natural resource management. p. 80–92. In : R.R. Johnson and J.F. McCormack (tech. coord.). Strategies for protection and management of floodplain wetlands and other riparian ecosystems. [Callaway Gardens, Georgia, December 11–13, 1978]. USDA Forest Service GTR-WO-12. 410 p. Washington, D.C.
Hehnke, M., and C. Stone. 1979. Value of riparian vegetation to avian populations along the Sacramento River system. p. 228–235. In : R.R. Johnson and J.F. McCormack (tech. coord.). Strategies for protection and management of floodplain wetlands and other riparian ecosystems. [Callaway Gardens, Georgia, December 11–13, 1978]. USDA Forest Service GTR-WO-12. 410 p. Washington, D.C.
Hoover, R. 1970. The vascular plants of San Luis Obispo County, California. 350 p. University of California Press, Berkeley.
Hora, B. (ed). 1981. The Oxford encyclopedia of trees of the world. 288 p. Oxford University Press, New York, N.Y.
Hosner, J. 1962. The southern bottomland hardwood region. p. 296–333. In : J. Barrett (ed.). Regional silviculture of the United States. 610 p. Ronald, New York, N.Y.
Hsiao, J. 1973. A numerical taxonomic study of the genus Platanus based on morphological and phenolic characters. Amer. Journ. Bot. 60:678–684.
Jepson, W. 1910. The silva of California. University of California Mem. Vol. 2. 480 p. University of California, Berkeley.
Kahrl, W. 1979. The California water atlas. Prepared by the Governor's Office of Planning and Research in cooperation with the California Department of Water Resources. 118 p. Sacramento, Calif.
Kramer, P., and T. Kozlowski. 1979. Physiology of woody plants. 811 p. Academic Press, New York, N.Y.
Larcher, W. 1975. Physiological plant ecology. 252 p. Springer Verlag, New York, N.Y.
Little, E., Jr. 1953. Check list of native and naturalized trees of the United States (including Alaska). USDA Forest Service Agriculture Handbook No. 41. 472 p.
Little, E., Jr. 1971. Atlas of United States trees. Volume 1: conifers and important hardwoods. USDA Forest Service, Misc. Publ. No. 1146. 9 p., 200 maps.
Little, E., Jr. 1976. Atlas of United States trees. Volume 3: minor western hardwoods. USDA Forest Service, Misc. Publ. No. 1314. 13 p., 210 maps.
MacArthur, R. and J. MacArthur. 1961. On bird species diversity. Ecology 43:594–598.
Major, J. 1963. A climatic index to vascular plant activity. Ecology 44:485–498.
Major, J. 1977. California climate in relation to vegetation. p. 11–74. In : M. Barbour and J. Major (ed.). Terestrial vegetation of California. 1002 p. John Wiley, New York, N.Y.
Mather, J., D. Carter, and F. Hare. 1964. Average climatic water balance data of the continents. Part VII: United States. C.W. Thornthwaite Associates Lab. Climatol. Pub. Climatol. 17:419–615.
Miller, G. 1955. The genus Fraxinus , the ashes in North America, north of Mexico. Cornell Agri. Exp. Sta. Mem. 335. 64 p.
Monk, C. 1966. An ecological significance of evergreenness. Ecology 47:504–505.
Mooney, H., J. Kummerow, A. Johnson, D. Parsons, S. Keeley, A. Hoffman, R. Hays, J. Giliberto, and C. Chu. 1977. The producers—their resources and adaptive responses. p. 85–143. In : H. Mooney (ed.). Convergent evolution in Chile and California mediterranean climate ecosystems. 224 p. Dowden, Hutchinson & Ross, Stroudsburg, Penn.
Munz, P. 1959. A California flora. 1681 p. University of California Press, Berkeley.
Munz, P. 1968. Supplement to a California flora. 224 p. University of California Press, Berkeley.
Page, B. 1981. The southern Coast Ranges. p. 329–417. In : W. Ernst (ed.). The geotectonic development of California. 706 p. Prentice-Hall, Englewood Cliffs, N.J.
Pirone, P. 1970. Diseases and pests of ornamental plants. Fourth edition. 546 p. Ronald, New York, N.Y.
Raven, P., and D. Axelrod. 1978. Origin and relationships of the California flora. Uniiversity of California Publ. in Botany. Volume 72. 134 p. University of California Press, Berkeley.
Rehder, A. 1940. Manual of cultivated trees and shrubs. Second edition. 996 p. Macmillan, New York, N.Y.
Roberts, W., J. Howe, and J. Major. 1977. A survey of riparian forest flora and fauna in California. p. 3–19. In : A. Sands (ed.). Riparian forests in California: their ecology and conservation. Institute of Ecology Pub. No. 15. 122 p. University of California, Davis.
Robichaux, R. 1977. Geologic history of the riparian forests of California. p. 21–34. In : A. Sands (ed.). Riparian forests in California: their ecology and conservation. Institute of Ecology Pub. No. 15. 122 p. University of California, Davis.
Rosenzweig, M. 1968. Net primary productivity of terrestrial communities: prediction from climatological data. Amer. Nat. 102:67–74.
Rossi, R. 1980. History of cultural influences on the distribution and reproduction of oaks in California. p. 7–18. In : T. Plumb (tech. coord.). Proceedings of the symposium on the ecology, management, and utilization of California oaks. [Claremont, Calif., June 26–28, 1979]. USDA Forest Service GTR-PSW-44. 368 p. Berkeley, California.
Rundel, P. 1980. Adaptations of mediterranean-climate oaks to environmental stress. p. 43–54. In : T. Plumb (tech. coord.). Proceedings of the symposium on the ecology, management, and utilization of California oaks. [Claremont, Calif., June 26–28, 1979]. USDA Forest Service GTR-PSW-44. 368 p. Berkeley, California.
Sands, A. (ed.). 1977. Riparian forests in California: their ecology and conservation. Institute of Ecology Pub. No. 15. 122 p. University of California, Davis.
Small, A. 1974. The birds of California. 310 p. Collier, New York, N.Y.
Smith, C. 1976. A flora of the Santa Barbara region, California. 331 p. Santa Barbara Museum of Natural History, Santa Barbara, Calif.
Spurr, S., and B. Barnes. 1980. Forest ecology. Third edition. 687 p. John Wiley, New York, N.Y.
Stebbins, G. 1974. Flowering plants: evolution above the species level. 399 p. Belknap Harvard, Cambridge, Mass.
Stebbins, G., and J. Major. 1965. Endemism and speciation in the California flora. Ecol. Monog. 34:1–35.
Sudworth, G. 1908. Forest trees of the Pacific Slope (1967 reprint of 1908 edition). 455 p. Dover, New York, N.Y.
Thompson, K. 1977. Riparian forests of the Sacramento Valley, California. p. 35–38. In : A. Sands (ed.). Riparian forests in California: their ecology and conservation. Institute of Ecology Pub. No. 15. 122 p. University of California, Davis.
Thorne, R. 1976. A phylogenetic classification of the Angiospermae. p. 35–106. In : M. Hecht, W. Steere, and B. Wallace (ed.). Evolutionary biology. Volume 9. Plenum, New York, N.Y.
Thornthwaite, C. 1948. An approach toward a rational classification of climate. Geogr. Rev. 38:55–94.
Thornthwaite, C., and J. Mather. 1955. The water balance. Drexel Inst. Technol. Pub. Climatol. 8:1–104.
Tucker, J. 1980. Taxonomy of California oaks. p. 19–29. In : T. Plumb (tech. coord.). Proceedings of the symposium on the ecology, management, and utilization of California oaks. [Claremont, Calif., June 26–28, 1979]. USDA Forest Service GTR-PSW-44. 368 p. Berkeley, California.
US Department of Agriculture. 1941. Climate and man: yearbook of agriculture. 1248 p. US Department of Agriculture, Washington, D.C.
US Department of Commerce. 1970. Climate of California. In : Climates of the states: climatography of the United States, 60–4. 57 p. US Depatrment of Commerce, Washington, D.C.
US Department of Interior, Geological Survey. 1977. Water resources data for California. Water year 1977. Volume 4. USGS Water-data Report CA-77-4.
Walter, H. 1979. Vegetation of the earth and ecological systems of the geo-biosphere. Second edition. 274 p. Springer Verlag, New York, N.Y.
Wauer, R. 1977. Significance of Rio Grande riparian systems upon the avifauna. p. 165–174. In : R.R. Johnson and D.A. Jones (tech. coord.). Importance, preservation, and management of riparian habitat: a symposium. [Tuscon, Ariz., July 9, 1977]. USDA Forest Service GTR-RM-43. 217 p. USDA Forest Service Range and Experiment Station, Fort Collins, Colorado.
Wauer, R. 1978. The breeding avifauna of Isla Tiburon, Sonora, Mexico. Unpublished manuscript. 75 p. USDA Fish and Wildlife Service, Washington, D.C.
Wharton, C, and M. Brinson. 1979. Characteristics of southeastern river systems. p. 3240. In : R.R. Johnson and J.F. McCormack (tech. coord.). Strategies for protection and management of floodplain wetlands and other riparian ecosystems. [Callaway Gardens, Georgia, December 11–13, 1978]. USDA Forest Service GTR-WO-12. 410 p. Washington, D.C.
Willis, J., and H. Airy Shaw. 1973. A dictionary of the flowering plants and ferns. Eighth edition. 1245 p. Cambridge University Press, London.
Willson, M. 1974. Avian community organization and habitat structure. Ecology 55:1017–1029.
A Brief History of Riparian Forests in the Central Valley of California[1]
Edwin F. Katibah[2]
Abstract.—Riparian forests once occupied substantially greater areas in the Central Valley of California than they do today. This paper explores the hydrologic influences which allowed the original riparian forests to establish themselves, the extent and reasons for the decline of the pre-settlement forests, as well as an estimate of the extent of today's remaining forests.
Introduction
One hundred and fifty years ago, California's Central Valley was endowed with a natural environment the scope and magnitude of which it is difficult, if not impossible, to fully comprehend today. Two major river systems, the Sacramento and the San Joaquin, drained the Valley. Flooding in the winter and spring, these rivers and their tributaries formed vast flood basins and huge, shallow seasonal lakes. Marsh vegetation (primarily Scirpus spp. and Typha spp.) occupied these wetter sites. Extensive perennial grassland (Stipa spp.) and scattered valley oak (Quercuslobata ) woodlands were found on the drier uplands, while the southern end of the Valley had large areas of saltbush (Atriplex spp.) desert. Through all of these vegetation communities, along the major river and stream systems, were strips of dense forest. These riverine, or riparian, forests developed on the natural levees of river-deposited silt, lining most of the Valley's drainages.
Riparian forests are structurally and floristically complex vegetation communities. These forests are difficult to characterize, for they occur in many different forms throughout the Valley. Under ideal conditions, these forests consist of several layers with dense undergrowth, similar in some cases to tropical jungles (Holmes etal . 1915). Fremont cottonwood (Populusfremontii ), California sycamore (Platanusracemosa ), willow (Salix spp.), and valley oak are common upper canopy species found throughout the Valley. Such species as box elder (Acernegundo subsp. californicum ), Oregon ash (Fraxinus latifolia ), and various species of willow generally occur in intermediate layers. Vines (lianas) are characteristic of many riparian forests, with wild grape (Vitiscalifornica ), poison oak (Rhus diversiloba ), Dutchman's pipe vine (Aristolochiacalifornica ), and wild clematis (Clematis spp.) growing through the various layers. Riparian forest undergrowth has a very diverse flora which varies widely throughout the Valley. Too many characteristic undergrowth plant species occur to mention but a few: mugwort (Artemisia douglasiana ), mulefat (Baccharisviminea ), wild rose (Rosacalifornica ), and blackberry (Rubus spp.).
Riparian forests have been greatly reduced or eliminated throughout much of the Valley. Ecologically they continue to play an important role with many plant and animal species dependent on them. Riparian forests are popular recreation sites, providing a wide range of beneficial values for the Valley's populace. These facts, among others, have recently aroused an interest in riparian forest ecology and management by both the general public and various Federal, State, and local agencies. This new interest has prompted questions as to why these forests occurred more along some river systems than others; how extensive the pre-settlement forests were; what caused their decline; and how many of these forests remain today. This paper attempts to provide a brief, informative look into these questions.
Hydrology of the Central Valley
There is significant hydrologic diversity throughout the Central Valley, and it was this diversity which was in part responsible for differences between individual riparian forests. For example, the Valley has two major riverine hydrologic systems: that of the Sacramento Valley component in the north and of the San Joaquin Valley component in the south. The influences of these major hydrologic systems on the nature of
[1] Paper presented at the California Riparian Systems Conference. [University of California, Davis, September 17–19, 1981].
[2] Edwin F. Katibah is an Associate Specialist, Department of Forestry and Resource Management, University of California, Berkeley.
the riparian forests associated with them were profound. Figure 1 depicts the Central Valley and its major surface hydrology as it may have appeared under pre-settlement conditions.
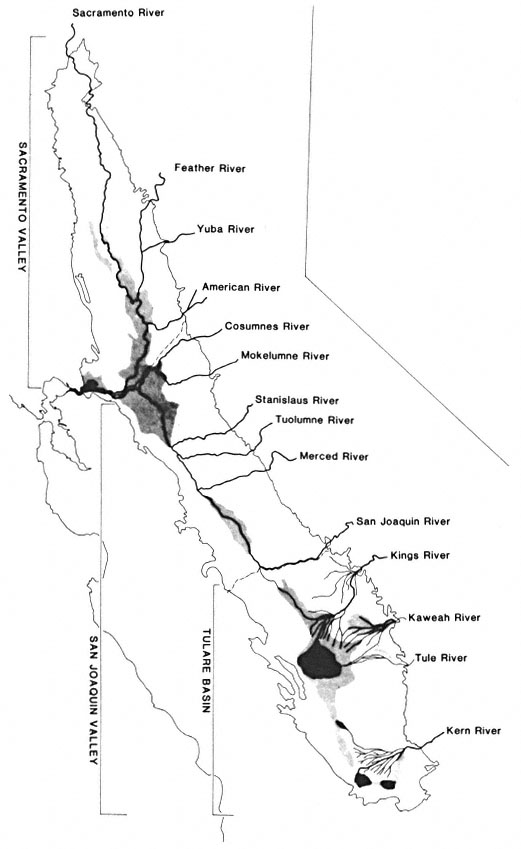
Figure l.
Surface hydrology of the Central Valley as it may have appeared
around 1850. Areas in black within the Tulare Subbasin represent
seasonal lakes. Shaded areas, shown throughout the Valley,
indicate flood basins and freshwater marshes.
Hydrology of the Sacramento Valley
The Sacramento Valley is bordered by the mountains of the Coast Ranges to the west, the Klamath and Cascade Ranges to the north, and the Sierra Nevada to the east. To the south, the Sacramento Valley joins the San Joaquin Valley at the Sacramento/San Joaquin River Delta. The comparatively dry interior Coast Range mountains have no large rivers draining into the Valley, only streams, some of the larger being Stony, Cache, and Putah Creeks. The Sacramento River originates in the Klamath Mountains and is joined by two rivers, the McCloud and the Pit, in what is now Shasta Lake. The Sierra Nevada mountains to the east provide the greatest number of rivers and major streams draining into the Sacramento Valley—the Feather, Yuba, Bear, and American Rivers, and Butte and Big Chico Creeks.
Numerous other streams also flowed into the Sacramento Valley from the surrounding mountains. Not all of these streams actually reached the Sacramento River. Historically, natural levees and naturally occurring flood basins prevented some streams from reaching the main rivers. Instead, these streams spread out "through a welter of distributaries" (Thompson 1961) on the Valley floor. These distributaries typically ended in "sinks" of tule marsh. Putah, Cache, and Butte Creeks are among those streams which never joined the main river network in the Sacramento Valley.
The Sacramento Valley and its surrounding foothills, unlike the San Joaquin Valley region, receive substantial rainfall in the winter and early spring. This resulted in Sacramento Valley rivers experiencing maximum flows from December through March instead of May and June as is characteristic of most western rivers, including those in the San Joaquin Valley (Fortier 1909). Snowmelt fortified the river flow in the Sacramento Valley through the late spring. Annual summer drought brought the low flow rates found in these rivers through late fall.
During the peak flows of the Sacramento Valley rivers, the flood basins were filled by sediment-carrying waters. The natural levees dividing the flood basins from the major rivers were initially developed and then augmented by this annual flood cycle. Impressive natural levees along the Sacramento River, ". . . from 5 to 20 feet above the flood basins . . ." and 1.6–16 km. (1–10 mi.) in width, averaging 4.8 km. (3 mi.), ". . . formed corridors of generally dry land during times of flooding . . ." (Thompson 1961). The other major Sacramento Valley rivers and streams also formed well-developed natural levees.
Hydrology of the San Joaquin Valley
The San Joaquin Valley is bounded by the flat relief of the Sacramento/San Joaquin River Delta to the north, the mountains of the Sierra Nevada to the east, the Coast Ranges to the west, and the Tehachapi Mountains to the south.
The Coast Ranges and the Tehachapi Mountains bordering the San Joaquin Valley are very arid. Thus, the streams which originate from these mountains were characteristically intermittent in flow. Probably the most notable of these intermittent streams was Los Gatos Creek, whose alluvial fan helped form the Tulare Subbasin, a major influence in the hydrology of the San Joaquin Valley.
Numerous Sierra Nevada rivers and streams flowed into the San Joaquin Valley, including the Cosumnes, Mokelumne, Calaveras, Stanislaus, Tuolumne, Merced, Chowchilla, Fresno, San Joaquin, Kings, Kaweah, Tule, White and Kern Rivers.
The San Joaquin Valley is itself divided into two distinct hydrologic subbasins: the San Joaquin and the Tulare. The San Joaquin Subbasin is drained by the San Joaquin River; the Tulare Subbasin has no perennial surface outlet.
The Tulare Subbasin was formed at the south end of the San Joaquin Valley by the merging of alluvial fans from the Kings River to the east and Los Gatos Creek to the west (Cone 1911). Water originating from the major Tulare Subbasin rivers—the Kings, Kaweah, Tule, White, and Kern—flowed into this subbasin and found no normal outlet to the sea. Instead, large inland lakes formed—the Tulare, Buena Vista, Kern, and Goose. These largely temporary lakes, extremely shallow as they flooded the nearly flat landscape, rose dramatically as winter and spring runoff filled them. As the seasonal lakes filled beyond capacity they flowed into one another, finally rising above the natural alluvial barriers which divided the Tulare and San Joaquin Subbasins, sending tremendous quantities of water down the Fresno Slough into the San Joaquin River.
Later in the season, after the overland flow of water had ceased, substantial quantities of water were still drained from the Tulare Subbasin into the San Joaquin River via subsurface flow. This underground accession may have doubled the San Joaquin River's volume (Irrigation in California 1873). This undoubtedly helped to maintain the flow of the San Joaquin River in its southern reaches during the long, dry California summers.
The San Joaquin Valley rivers, whose waters were primarily snowmelt, tended to reach maximum flow in May and June. In contrast, peak flow of the Sacramento was usually in March, although some of the major peak flow rainfloods have occurred much earlier in the winter (1955–56 flood—December and January; 1964–65 flood—December and January; 1970 flood—January). In addition, the San Joaquin River's flow into the Delta in its peak flow period was less than onehalf the discharge rate of the Sacramento River during its usual peak flow period in March. Despite this difference in peak flow timing, the two rivers discharged approximately equal amounts of water into the Delta.
San Joaquin Valley rivers and streams in some instances did not produce the large, natural levees characteristic of the Sacramento Valley. Peak water flows in San Joaquin Valley rivers and streams were typically less than those in the Sacramento Valley, thus limiting their ability to pick up and carry sediment for great distances. Natural levees did form along the major northern San Joaquin Valley rivers—the Tuolumne, Stanislaus, Merced, Mokelumne, Cosumnes, and northern San Joaquin.
The southern (upper) reaches of the San Joaquin River developed natural levees only poorly, and only as the river entered the Valley floor. Never a particularly big river, it ranked third in peak flow after the Tuolumne and Kings Rivers (Cone 1911). Relatively low-energy peak flows resulted in suspended sediment deposition and natural levee formation only where it first entered the Valley. From there until it reached Fresno Slough, the San Joaquin River received no surface tributaries. At that point it received the surface floodwater flows through the Fresno Slough from the Tulare Subbasin and the underground flow through the extensive Tulare Subbasin aquifer.
Both of these flows were substantial, but both lacked significant sediment content. The overland flow through Fresno Slough had already deposited its sediment load in the shallow Tulare Subbasin lakes. The subsurface waters had been filtered of any sediment long before they joined the San Joaquin River. Thus while the southern San Joaquin River gained a large water accession, especially during the peak spring flood, it was unable to build any significant natural levees because of the low sediment load. With no natural levees to contain its waters, the San Joaquin River spread out over the flat Valley floor, sustaining the large freshwater marshes still found there today. The first major sediment-carrying waters to reach the San Joaquin River for many miles occurred at its confluence with the Merced River. From here to the Delta, substantial natural levees were built along the San Joaquin River.
The Tulare Subbasin rivers developed natural levees where these rivers first entered the Valley. The shifting courses of these rivers undoubtedly allowed many miles of levees to be formed, though they were quite narrow and confined compared to the levees of the Sacramento Valley rivers.
Extent of Pre-Settlement Riparian Forests
While the largest and most diverse riparian forests occurred on rivers having natural levees, well-developed riparian systems were found along virtually all watercourses in the Central Valley. Most riverine floodplains supported riparian vegetation to about the 100-year flood line. Virtually all watercourses supported dense vegetation from the water's edge to the outer edge of the riparian (moist soil) zone, whether or not natural levees were present. The overall presettlement riparian vegetation pattern was one of stringers or corridors of dense, mesic, broadleaf vegetation of varying widths bounding the watercourses, the widths being determined by local hydrologic and landform characteristics.
According to various accounts, the Sacramento Valley had approximately 324,000 ha. (800,000 ac.) of riparian forest remaining after 1848 (Smith 1977; Roberts etal . 1977). No comparable estimate for riparian forests is available for the San Joaquin Valley. However, based on a map compiled by J. Greg Howe (ibid .) showing presumptive original riparian forest distribution, and estimates by this author, it is
conservatively estimated that the Central Valley had greater than 373,000 ha. (921,000 ac.) of riparian forest under pre-settlement conditions.
Howe's map is based on early soil maps and covers an area in the Central Valley from the Sacramento River at Redding in the north to the Merced River in the south. I measured for areal extent the presumptive riparian forests shown on Howe's map. This estimate, presented in table 1, yields a value of 312,400 ha. (771,600 ac.) of pre-settlement riparian forest. This value must be considered conservative for that area, as Howe's map depicts only the large, contiguous riparian forests. The many smaller areas of riparian-indicator soil-types were below the mapping level of the historic soil maps used in the presumptive-riparian-forest map preparation.
In addition, Howe's map excluded the southern rivers of the San Joaquin Valley—the San Joaquin below its confluence with the Merced; and the Kings, Kaweah, Tule, and Kern. The above figure reflects that exclusion. I judged the riparian systems associated with those rivers to have totalled an estimated 20,200 ha. (50,000 ac.) (table 1). Furthermore, I estimated approximately 40,500 ha. (100,000 ac.) to account for the riparian forest vegetation present along the small streams, sloughs, lakes, ponds, and marsh borders throughout the entire Central Valley (table 1). These estimates are undoubtedly quite conservative and subject to considerable refinement.
|
|
Decline of Central Valley Riparian Forests
"No natural landscapes of California have been so altered by man as its bottomlands" (Bakker 1972). The once-lush riparian forests, forming natural vegetation corridors along many of the Central Valley's watercourses, are mostly gone today. These forests were, in Thompson's words, ". . . modified with a rapidity and completeness matched in few parts of the United States" (Thompson 1961).
The reasons for the rapid decline of this once extensive ecosystem are not hard to find; one needs only to review the cultural history of the Central Valley for the last 150 years.
Prior to 1822 the land known as California was claimed and ruled by Spain. Little development occurred during this period, and at the cessation of Spanish rule in 1822 only about 30 ranches or farms had been granted in California (Fortier 1909). Mexico assumed control of California until 1848. By ". . . 1846 no less than eight hundred large tracts containing some of the best land in the State had been given away" (ibid .). The character and size of the large Mexican land grants had a profound influence on the social, commercial, and agricultural development of the Central Valley (ibid .), development which would ultimately and adversely affect riparian vegetation.
With the annexation of California to the United States in 1848, rapid development of the Central Valley began. The Gold Rush, beginning in 1849, exerted enormous land use pressures and led to rapid and often unplanned development of the Valley.
Riparian vegetation removal was one of the first significant losses in the natural environment. The large number of immigrants seeking their fortunes in the gold-bearing Mother Lode rivers and streams soon found that agriculture provided a much more stable and practical existence. The riparian forests, often the only significant woody vegetation on the Valley floor, were utilized by the growing agricultural community for fencing, lumber, and fuel (Thompson 1961). Steamships using the Sacramento River were also heavy users of local wood fuel. Knight's Landing on the Sacramento River was a site where cordwood was loaded onto these ships. It has been speculated that this wood came from the Cache Creek and Sacramento River riparian forests because Knight's Landing is adjacent to the treeless Yolo flood basin (ibid .). This supplying of fuel wood to the numerous woodburning vessels on the Sacramento River must have made a significant contribution to the early destruction of the local riparian forests (ibid .).
As early as 1868 the general scarcity of woody vegetation was noted in the Valley by some of its inhabitants (ibid .). The pressures on riparian forest vegetation continued as farmers found that the soil on the natural levees was highly fertile, easily managed, and not subject to the seasonal flooding of nearby lower-lying ground (ibid .). As agriculture expanded in the Central Valley, water demand began to exceed water supply. Farmers also found that the Valley had too much water in the winter and spring and not enough in the summer. Water development and reclamation projects were started, primarily for agriculture and community flood protection, and rapidly eliminated many of the Valley's native wetland systems.
With agricultural expansion, cities grew to support the new industry. Many Valley towns and cities were built in flood basins and upon active floodplains, and were subject to seasonal flooding. The city of Sacramento suffered a tremendous flood in 1850, and its response, the buil-
ing of levees around the town, ". . . set the course for Valley development over the next several generations" (Karhl 1979). To promote the reclamation of the tule marsh and floodplain lands, the Arkansas Act of 1850 was applied in California. This act gave the State of California millions of acres of federally owned floodplains, provided that the State drain and reclaim these lands. The Arkansas Act of 1850 stipulated that all manmade levees were to be constructed along natural drainage systems. The Green Act of 1868, passed by the California Legislature, however, freed the reclamation process of most controls. The effects of the Green Act were devastating to riparian forests. Levees were built for the convenience of landowners with little or no regard for the natural hydrologic systems. Remaining riparian forests, occupying natural levees along river courses, were destroyed in the quest to protect lands from flooding.
As in the Sacramento Valley, artificial levees were built along major San Joaquin Valley rivers. San Joaquin Valley agriculture faced different water-related problems. Winter and spring rainfall there is substantially less than in the Sacramento Valley, thus San Joaquin Valley land needed to be irrigated if it was to reliably produce crops. With the Green Act as guiding legislation, more than 1,600 km. (1,000 mi.) of irrigation canals were developed by 1878 in Fresno County alone (ibid .).
In the following years, and continuing up to the present time, numerous and controversial water projects have been the hallmark of Central Valley development. The demand for water, so tied to the agricultural, commercial, and urban development of the Valley, was, at least indirectly, responsible for the degradation of many of the remaining riparian forests. Artificial levees, river channelization, dam building, water diversion, and heavy groundwater pumping were among the factors which reduced the original riparian forest to the small, scattered remnant forests found today.
Present Extent of Remnant Riparian Forests
In 1979, the Geography Departments of California State University, Chico, and California State University, Fresno, under contract to the California Department of Fish and Game, compiled riparian vegetation distribution maps for the Central Valley (Nelson and Nelson 1983). This mapping effort provided an essentially complete inventory of all extant riparian vegetation (not just mature forest) in the Central Valley.[4]
Using these maps, the areas and lengths of riparian systems were calculated on an individual map and county basis.[5] Even though there is no explicit riparian forest category on these maps, applicable classifications were determined which should represent riparian forests. Using this approach, it was determined that approximately 41,300 ha. (102,000 ac.) of riparian forest remain in the Central Valley today (Katibah etal . 1983). Of the 41,300 ha. of forest, approximately 19,800 ha. (49,000 ac.) are in a disturbed and/or degraded condition based on the riparian mapping category code. Approximately 21,500 ha. (53,000 ac.) were identified as mature riparian forest, with no indication of condition. However, based on recent research findings (Katibah etal . in press), it can be surmised that the majority of these 21,500 ha. of mature riparian forest have been and are currently being heavily impacted by human activities.
Conclusions
The complex hydrologic systems found in the Central Valley of California under pristine conditions are gone. The original riparian forests, dependent on the diverse Valley hydrology, are likewise gone for the most part. Today's riparian forests are in a precarious position as the demand for greater land utilization by the agricultural industry and the spread of urbanization threaten the remaining forest tracts.
Offsetting this trend, however, is a greater apreciation of the values (economic and noneconomic) of riparian forests by Valley landowners and the general public. Riparian forests are present in some of the finest and most popular parks in the Central Valley. These forests provide habitat for many of the Valley's wildlife species. They also contain numerous and diverse native plant species.
These values, among others, must compete with the most complex and controversial issue of all: water. In California as in the rest of the West, water equals development, and California does not have adequate water to meet its anticipated future demands. How the remaining riparian forests will fare in the future is not known. As interest in and knowledge about this resource develops, and as hindsight provides an understanding of the past, it is hoped that a reasonable compromise can be achieved between this unique and valuable resoure and the needs of society.
[4] Central Valley riparian mapping project. 1979. Interpretation and mapping systems. Report prepared by the Riparian Mapping Team, Geography Department, California State University, Chico, with the Department of Geography, California State University, Fresno. Unpublished report to the Califronia Department of Fish and Game, Planning Branch, Sacramento. 24 p.
[5] Katibah, E.F., N.E. Nedeff, and K.J. Dummer. 1980. The areal and linear extent of riparian vegetation in the Central Valley of California. Final report to the California Department of Fish and Game, Planning Branch. Remote Sensing Research Program, Department of Forestry and Resource Management, University of California, Berkeley.
Literature Cited
Bakker, Elna S. 1972. An island called California. 357 p. University of California Press, Berkeley, California.
Cone, Victor M. 1911. Irrigation in the San Joaquin Valley, California. USDA Office of Experiment Stations, Bulletin 239. 62 p. Government Printing Office, Washington, D.C.
Fortier, Samuel. 1909. Irrigation in the Sacramento Valley, California. USDA Office of Experiment Stations, Bulletin 207. 99 p. Government Printing Office, Washington, D.C.
Holmes, L.C., J.W. Nelson, and party. 1915. Reconnaissance soil survey of the Sacramento Valley, California. USDA Publication. Government Printing Office, Washington, D.C.
Irrigation in California: the San Joaquin and Tulare Plains, a review of the whole field. 1873. 22 p. Record Steambook and Job Printing House, Sacramento, California.
Kahrl, William L. 1979. The California water atlas. Prepared by the Governor's Office of Planning and Research in cooperation with the California Department of Water Resources. 113 p. Sacramento, California.
Katibah, Edwin F., Nicole E. Nedeff, and Kevin J. Dummer. 1983. A summary of the riparian vegetation areal and linear extent measurements from the Central Valley riparian mapping project. In : R.E. Warner and K.M. Hendrix (ed.). California Riparian Systems. [University of California, Davis, September 17–19, 1981]. University of California Press, Berkeley.
Katibah, Edwin F., Kevin J. Dummer, and Nicole E. Nedeff. In press. Evaluation of the riparian vegetation resource in the Central Valley of California using remote sensing techniques. Proceedings of the ASP and ACSM fall technical meeting. [San Francisco, California, September 9-11, 1981].
Nelson, Charles W., and James R. Nelson. 1983. Central Valley riparian mapping project. In : R.E. Warner and K.M. Hendrix (ed.). California Riparian Systems. [University of California, Davis, September 17–19, 1981]. University of California Press, Berkeley.
Roberts, W.G., J.G. Howe, and J. Major. 1977. A survey of riparian forest flora and fauna in California. p. 3–19. In : A. Sands (ed.). Riparian forests in California: their ecology and conservation. Institute of Ecology Publication No. 15. 122 p. University of California, Davis.
Smith, F.E. 1977. A short reveiw of the status of riparian forests in California. p. 1–2. In : A. Sands (ed.). Riparian forests in California: their ecology and conservation. Institute of Ecology Publication No. 15. 122 p. University of California, Davis.
Thompson, K. 1961. Riparian forests of the Sacramento Valley, California. Annals Assoc. of Amer. Geog. 51:294–315.
The Importance of Riparian Systems to Amphibians and Reptiles[1]
John M. Brode and R. Bruce Bury[2]
Abstract.—California has a rich herpetofauna, including about 120 native species. Riparian systems provide habitat for 83% of the amphibian and 40% of the reptile species. Amphibians and reptiles utilize these systems to varying degrees and can be classified according to the type of use. Riparian systems provide corridors for dispersal and also allow certain species to use otherwise unsuitable environments. Amphibians and reptiles may be abundant in riparian systems where they may outnumber other taxa. Harvesting timber and creating reservoirs are detrimental to amphibians and reptiles in the zone of influence of such activities. These activities have their greatest effects upon reptiles and amphibians whose entire life histories occur in the riparian zone.
Introduction
California has a rich herpetofauna, including about 120 native species. Amphibians and reptiles represent important ecological components of riparian communities, where they may reach high densities. In California, we estimate riparian systems provide habitat for 83% of the amphibians and 40% of the reptiles. Many species are permanent residents of the riparian zone, while others are transient or temporal visitors.
In many (if not most) natural communities, nongame species constitute the greatest portion of vertebrate species, individuals, and biomass; and they are energetically critical elements in the functioning of ecosystems (Bury etal . 1980). Based on figures compiled by Bury etal . (ibid .) 88% of the vertebrate species (fish excluded) in California are nongame.
Much emphasis has been placed on the loss of California's Central Valley riparian forests (Sands 1977). However, there are many other riparian systems in California that have suffered substantial degradation. Logging has proved detrimental to certain animal species that depend on cool, shaded streams. Reservoirs have been created on many streams in California, eliminating the original riparian environment and much of the herpetofauna, while providing habitat for nonnative species which are usually managed more intensely than the original fauna. Many of the species lost, especially the amphibians and reptiles, are endemic to California.
In this paper, we present background information on species diversity and abundance, review the habitat requirements of California amphibians and reptiles, and suggest use classifications for species using riparian systems. Lastly, we review the effects of logging on selected species and discuss the effects of reservoirs on amphibians and reptiles, presenting preliminary data from two preimpoundment studies.
Species Diversity and Abundance
Species diversity and abundance of amphibians and reptiles may be dramatic in riparian systems. For example, the riparian system of Corral Hollow Creek, San Joaquin County (fig. 1), supports 7 species of amphibians and 21 species of reptiles, including 13 species of snakes (Stebbins 1966; Sullivan 1981). Burton and Likens (1975) estimated there were 2,950 salamanders per ha. within the Hubbard Brook Experimental Forest, New Hampshire, and concluded there were more salamanders than either birds or small mammals. In biomass, salamanders were 2.6 times greater than birds and approximately equal to mammals. Burton and Likens were surprised at this result as most ecologists have ignored amphibians in ecosystem energy flow and nutrient cycling studies while considering birds and mammals in detail.
[1] Paper presented at the California Riparian Systems Conference. [University of California, Davis, September 17–19, 1981].
[2] John M. Brode is a Herpetologist, Endangered Species Program, California Department of Fish and Game, Rancho Cordova, Calif. R. Bruce Bury is a Research Zoologist, Ecology Section, Denver Wildlife Research Center, USDI Fish and Wildlife Service, Fort Collins, Colo.
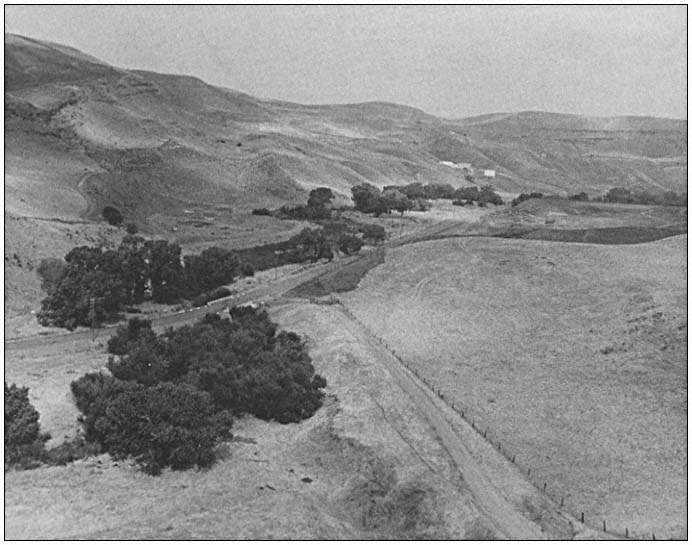
Figure 1.
Corral Hollow Creek, San Joaquin County, California. This riparian system supports
7 species of amphibians and 21 species of reptiles. Photo by John E. Hummel.
Other workers have obtained similar results regarding amphibian abundance. Nussbaum[3] estimated the density of the Siskiyou Mountain salamander (Plethodon stormi ) to be 0.27 per m2 (2,700 per ha.) in optimal habitat. Murphy and Hall (1981) reported in certain streams, Pacific giant salamander (Dicamptodonensatus ) was the dominant vertebrate in both biomass and frequency of occurrence and made up as much as 99% of the total predator biomass in some sites. The population density of an eastern stream salamander (Desmognathusfuscus ) was estimated at 0.4 to 1.4 per m2 (400 to 1,400 per ha.) (Spight 1976); in certain areas, male D . ochrophaeus occur at densities of 4.4 per m2 (Tilley 1974). Western pond turtle (Clemmysmarmorata ) may reach densities of 425 per ha. in California ponds and streams (Bury 1979). Fitch (1975) estimated densities of 1,000 to 1,500 ringneck snakes (Diadophispunctatus ) per ha. Sullivan (1981) reported a density of 22.4 snakes per km. along an 11-km. road transect in Corral Hollow.
Use of Riparian Systems by Amphibians and Reptiles in California
Amphibians that utilize riparian systems in California can be placed in one of three classifications, according to their dependency upon
[3] Nussbaum, R.A. 1974. The distributional ecology and life history of the Siskiyou Mountain salamander, Plethodon stormi , in relation to the potential impact of the proposed Applegate Reservoir on this species. 52 p. Report submitted to the US Army Corps of Engineers, Portland, Ore.
aquatic environments and the extent to which they utilize terrestrial riparian systems (table 1). All amphibians in California, except lungless salamanders of the family Plethodontidae, require aquatic environments to complete their life cycle. Certain frogs (Rana , Ascaphus ) and salamanders (Rhyacotriton , some Batrachoseps ) frequent the riparian zone throughout their lives. Other salamanders and newts (Ambystoma , Taricha ) and some toads (Bufo ) utilize riparian systems primarily for breeding, spending most of their adult life in upland areas. Lungless salamanders are more generalized in their habitat requirements, but many species utilize
|
riparian systems. Wide-ranging plethodontid salamanders (Ensatina ) have generalized habitat requirements in the mesic environments of northern California, but tend to associate with riparian systems in xeric environments.
Reptiles that utilize riparian systems in California can also be placed in one of three categories (table 2). Turtles (Clemmys ) and most garter snakes (Thamnophis ) depend on aquatic environments and occur primarily in the riparian zone throughout their lives. Some lizards (Gerrhonotus ) and snakes (Contia ) have rather general habitat requirements but become riparian obligates in arid portions of their range. The remaining reptiles that occur in riparian systems (Cnemidophorous , Pituophis , Lampropeltis ) are more generalized in their habitat requirements, but they frequent ecotones and water bodies associated with riparian areas.
The riparian zone also provides corridors of dispersal and islands of habitat for many species of amphibians and reptiles, especially in arid climates. The Gilbert's skink (Eumecesgilberti ) and ringneck snake (Diadophis punctatus ) are foothill species that extend their ranges into the Central Valley along the American River and other riparian corridors. The desert slender salamander (Batrachoseps aridus ) and Inyo Mountains salamander (B . campi ) are restricted to the narrow riparian zones of desert seeps and springs.
Historically, riparian corridors probably facilitated the maintenance of genetic continuity between populations. Now, due to habitat disruption, certain populations are isolated. The wide-ranging California slender salamander (Batrachoseps attenuatus ) was probably once common in the southern Sacramento Valley. Now, in the Valley this species is restricted to a few isolated remnants of valley oak woodland while still common elsewhere. Ultimate consequences of habitat disruption include local extinctions, reduction in species diversity, and loss of population heterogeneity.
Examples of Activities Detrimental to Amphibians and Reptiles in Riparian Systems
Timber Harvest
The tailed frog (Ascaphustruei ), the most primitive frog in North America, is highly specialized for life in cool, fast-flowing waters. The southern terminus of the species in the United States is in small streams along the north coast and in the North Coast Range of California (Bury 1968). Larval Ascaphus prefer temperatures at or below 15°C., and avoid waters over 22°C. (deVlaming and Bury 1970); such behavior is unlike any other native frog, and underscores the dependence of Ascaphus on a cool, shaded habitat. Removal of timber by lumbering or fire results in the disappearance of tailed frogs, apparently due to increased temperatures of the exposed stream-bed (Noble and Putnam 1931; Bury 1968).
Similarly the Olympic salamander (Rhyacotriton olympicus ) frequents cool ravines and rivulets in northwestern California. This species is absent in open (postlogging) habitat; logging apparently eliminates populations even in wet, coastal redwoods (Bury in prep.).
The Siskiyou Mountain salamander (Plethodonstormi ) inhabits shaded talus slopes in canyons and along stream courses above the floodplain. Nussbaum3 considered the gradual elimination of the overstory vegetation by clearcutting to be a serious threat to this species.
Reservoirs
Barrett and Cordone (1980) counted 1,272 reservoirs in California. Out of these, 926 (73%) are "mixed" or "warm water" types, those most commonly found on foothill and mid-elevation streams and rivers. Reservoirs have adverse effects on amphibians and reptiles by flooding their habitats. They often result in bodies of water with fluctuating levels, which prevents reestablishment of natural riparian communities. In addition, reservoirs are usually managed for human activities.
Specific examples of the effects of reservoirs on amphibians and reptiles are few. Nussbaum3 estimated that the Applegate Reservoir in Oregon and California would cover 1.06% of the total known range and 2.1% of the estimated total population of the Siskiyou Mountain salamander. He further stated that although the construction of Applegate Reservoir in itself will pose no threats to the continued existence of P . stormi , the effects of Applegate Reservoir added to numerous other man-caused effects could seriously threaten the existence of the species.
We do not have data regarding the numbers of individual amphibians and reptiles that may have been affected by previous reservoir construction in California, but information on two proposed reservoir projects will serve as examples of what may be lost.
Los Vaqueros Reservoir
The primary effect of the proposed Los Vaqueros Reservoir will be on Kellogg Creek, Contra Costa County. Preliminary investigations by the Department of Fish and Game (DFG) indicate that the Kellogg Creek area supports at least 6 species of amphibians and 12 species of reptiles. The reservoir, as proposed, would inundate 12 km. of tributaries. An additional 6.4 km. of Kellogg Creek would be affected below the dam due to changes in streamflow.
A species of special concern that occurs in Kellogg Creek is the red-legged frog (Rana aurora —fig. 2), which is well adapted for living in arid environments with intermittent or temporary aquatic habitat. However, it has
|
little tolerance for habitat disturbances or competition from exotic species. Approximately 18 km. of red-legged frog habitat, virtually the entire Kellogg Creek population, could be adversely affected if Los Vaqueros Reservoir impoundment project is built.
Thomes-Newville Reservoir
The primary effect of the proposed Thomes-Newville Reservoir will be on the North Fork Stony Creek, Glenn and Tehama Counties. Preliminary investigations by DFG indicate that the North Fork Stony Creek area supports at least 4 species of amphibians amd 14 species of reptiles. The proposed reservoir will inundate about 14 km. of perennial stream and about 40 km. of intermittent stream. In addition, about 13 km. of Thomes Creek may be affected by water diversion. Another species of special concern, the foothill yellow-legged frog (Ranaboylei ) occurs in North Fork Stony Creek and its tributaries. These frogs are adapted to rocky foothill streams. Salt Creek, on the project site, supports an excellent population of yellow-legged frogs. The majority of the yellow-legged frog population will be affected adversely if this project is completed.
Conclusions
Amphibians and reptiles represent important ecological components of riparian communities. Many species are permanent residents of the riparian zone, while others are transient or temporal visitors.
Amphibians and reptiles may be abundant in riparian systems where they can outnumber other taxa. Riparian systems provide important corridors of dispersal for many species. Disruption of these corridors can cause isolation and may lead to local extinctions.
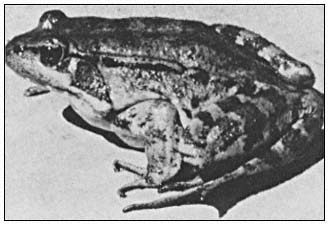
Figure 2.
Adult red-legged frog (Rana aurora ). Photo by Robert L. Livezey.
Activities which affect riparian systems adversely have their greatest effects on those amphibians and reptiles that occur in the riparian zone throughout their life. There is critical need for more quantified studies on how these activities directly affect riparian herpetofaunas; and a need for research on the relation of amphibians and reptiles to structural diversity of riparian vegetation.
Acknowledgements
We thank Kimberly A. Nicol and David P. Muth for assistance in preparing the tables. Stephen J. Nicola and Larry L. Eng reviewed an early draft.
Literature Cited
Barrett, John G., and Almo J. Cordone. 1980. The lakes of California. Inland Fish. Admin. Rep,. 80–5. 10 p. California Department of Fish and Game.
Burton, Thomas M., and Gene E. Likens. 1975. Salamander population and biomass in the Hubbard Brook experimental forest, New Hampshire. Copeia 1975:541–546.
Bury, R. Bruce. 1968. The distribution of Ascaphustruei in California. Herpetologica 24:39–46.
Bury, R. Bruce. 1979. Population ecology of freshwater turtles. In : Marion Harless and Henry Morlock (ed.). Turtles: perspectives and research. 695 p. John Wiley and Sons, Inc., New York, N.Y.
Bury, R. Bruce, Howard W. Campbell, and Norman J. Scott, Jr. 1980. Role and importance of nongame wildlife. Trans. 45th North Amer. Wildl. Nat. Res. Conf. 1980:197–207.
deVlaming, Victor L., and R. Bruce Bury. 1970. Thermal selection in tadpoles of the tailed frog, Ascaphustruei . J. Herpetol. 4: 179–189.
Fitch, Henry S. 1975. A demographic study of the ringneck snake (Diadophispunctatus ) in Kansas. Univ. Kansas Mus. Nat. Hist. Publ. 62. 53 p.
Murphy, Michael L., and James D. Hall. 1981. Varied effects of clear-cut logging on predators and their habitat in small streams of the Cascade Mountains, Oregon. Can. J. Fish Aquat. Sci. 38:137–145.
Noble, G.K., and P.G. Putnam. 1931. Observation on the life history of Ascaphustruei Stejneger. Copeia 1931:97–101.
Sands, Anne (ed.). 1977. Riparian forests in California: their ecology and conservation. Institute of Ecology Pub. 15. 122 p. University of California, Davis.
Spight, T.M. 1967. Population structure and biomass production by a stream salamander. Amer. Midl. Nat. 78:437–447.
Stebbins, Robert C. 1966. A field guide to western reptiles and amphibians. 279 p. Houghton Mifflin Co., Boston, Mass.
Sullivan, Brian K. 1981. Distribution and relative abundance of snakes along a transect in California. J. of Herp. 15:247–248.
Tilley, S.G. 1974. Structure and dynamics of populations of the salamander Desmognathus ochrophaeus Cope in different habitats. Ecology 55:808–817.
Some Implications of Population Growth to California's Renewable Resources[1]
Judith Kunofsky[2]
Abstract.—Efforts at better management of riparian systems will ultimately fail unless zero population growth is achieved. The increasing population in the United States, often overlooked as a causal factor, has contributed to the significant deterioration of riparian resources in this country. Advocates of riparian protection can be a vital link between proponents of population stabilization and the public and political leaders capable of effecting reversal of present population trends.
Introduction
This paper is a presentation that is not of original research. Nor am I a researcher. Rather, its perspective is that of advocate, and I hope it will be regarded as welcome diversity to other technical presentations, rather than as an aberration.
The organization I represent, Zero Population Growth (and, in particular, its California arm), is a political and educational non-profit membership organization founded in 1969 to advocate a rapid end to human population growth in this country and around the world. We have active programs in Washington, D.C; in Sacramento; and in communities around the state and the country. In addition, my position as director of the Population and Growth Policy Program of the national Sierra Club enables me to represent that organization on this issue as well.
The case I will make is that efforts at better management of riparian resources will ultimately fail unless the population stops increasing—unless we achieve zero population growth.
For those unfamiliar with the concept, zero population growth (zpg) occurs globally if the number of births each year equals the number of deaths. In a limited geographical area such as the United States or California or this room, population change is more complicated. Population change equals the number of births plus the number of people moving in (immigrants), minus the number of deaths and the number of people moving out (emigrants). If these balance, if the population remains roughly constant from year to year, we achieve a state of zero population growth, also known as population stabilization.
Most people now believe that the population of the United States has stopped growing or is well under way toward stabilization. This is not true. The country's population is increasing by two and a half million people per year, the result of roughly 3.6 million births, 2.0 million deaths, and 800,000 net immigration (immigrants minus emigrants). While the goal is much closer than it was a decade ago in terms of the birth rate, zpg is neither here nor is it assured. Despite the decrease in the number of births per woman, continued population growth is resulting from the combination of an "echo" of the post-war baby boom and immigration to this country. Growth could continue for several decades or perhaps far into the distant future. The US population could increase from today's 228 million to 260 million in 50 years and stop increasing, or it could reach 350 million or more by the year 2030, depending on the future course of fertility in and migration to the United States. Here in California, the state's population of 24 million is increasing by almost half a million people per year (more precisely, 479,000). We are far from achieving zero population growth.
Current Status of Riparian Systems
It is useful to briefly review the status of this country's riparian systems, i.e.: ". . . the vegetation and associated animal life found in close proximity to streams and other watercourses, around lakes, and adjacent to springs, seeps, and desert oases" (Warner 1979). According to Dr. Warner, riparian systems are:
[1] Paper presented at the California Riparian Systems Conference. [University of California, Davis, September 17–19, 1981].
[2] Judith Kunofsky is past National President of Zero Population Growth (1977–1980) and continues to serve on its board of directors. She also heads the Population Growth Policy Program of the Sierra Club.
". . . perhaps the most important of all ecosystems to fish and wildlife," determining the health of associated aquatic environments, enhancing erosion control, and improving water quality (ibid .). They constitute important agricultural lands and sources of timber. Riparian vegetation is the source of nutrients for streams, of diverse recreational opportunities, of clean water, fuel, and shade.
However, America has in the last 150 years destroyed between 70% and 90% of her indigenous riparian resources and badly damaged much of the rest (US Council on Environmental Quality 1978). Examples of that destruction:
1) In 1977 the USDI Bureau of Land Management concluded that 83% of the riparian systems under its control were ". . . in unsatisfactory condition and in need of improved management, largely because of the destruction caused by excessive livestock grazing, road construction, and other damaging human activities" (Almand and Krohn 1979).
2) "On the 2.3 million acres of riparian lands and wetlands within the National Forest System, livestock grazing . . . timber harvest and associated road construction and silvicultural practices, recreation, public highway construction, and mining . . . all . . . continue to exert destructive pressures requiring prompt and concerted corrective measures (USDA Forest Service 1979).
3) In California: ". . . riparian systems are now so decimated as to be in jeopardy as a productive ecological resource" (Warner 1979).
What Is the Source of the Problem?
Our riparian resources are in such terrible shape because of human impact. This impact is increasing because of continued conversion of riparian lands to other uses—agriculture, forestry, livestock grazing, water control, highways, recreation, and housing. Directly or indirectly: ". . . all the dominant impacts are human ones" (ibid .).
ZPG maintains that the role of sheer humannumbers cannot be ignored, and an end to population growth, the achievement of zpg, is an integral part of any serious, long-term plan for rehabilitating and sustaining riparian systems. To the extent that researchers, planners, regulators, and concerned Americans ignore this issue, they are dooming their work to ultimate failure.
This appraisal of the effect of population growth is applicable to any ecosystem or natural feature. This is part of the difficulty of the population concept; the impact of the size and growth of the human population is relevant to all resources, exclusive to none, and therefore, unfortunately, too often overlooked or considered such a general problem as not to be worth mentioning in any specific context.
Protection of Riparian Systems
Returning to the discussion of riparian systems, since the source of the problems is human impact, we clearly need to change human activity. What does this mean?
First, we need specific management protections for riparian systems. However, even the best management will eventually be overwhelmed by continued population growth. When the pressure of human demands is great enough, any scheme of resource management or protection will go out the window. This is not a law of biology, but one of politics. When short-term human needs conflict with the long-term maintenance of the carrying capacity of an ecosystem, unfortunately we, as a society, as a world, seem to always sacrifice the long-term goal for the short-term need.
Resource management alone, however well-done, is simply not enough to protect riparian systems. These specific protections must be coupled with a reduction in demand for those services provided by riparian systems. This reduction requires a change in patterns of consumption (e.g., conservation and adoption of more environmentally sound technologies). It also necessitates a change in the quantity of consumption, which means, for example, fewer people directly enjoying the benefits of recreation along rivers, or fewer people relying on the products of riparian environments.
A decade ago, the Science and Technology Advisory Council of the California Assembly held a forum at the University of California, Davis, to analyze the nature and consequences of population change in the state. At that conference, demographers Kingsley Davis and Frederick G. Styles observed:
If any state in the United States epitomizes the dilemmas of advanced technology, it is California; and if any one of its problems embodies the dilemmas, it is rapid population growth . . . The productivity of its economy makes it a prime target for interstate and international migration, causing it to be . . . the most populous state in the union . . . Although it has been subject to temporary lulls in growth . . . its population increase has been remarkably persistent . . . The resulting environmental damage is greater than that found almost anywhere else in the United States. . . .
If advanced societies are to solve their environmental problems, not to mention other questions concerned with the quality of life, they cannot in-
dulge in continued rapid population growth. No sensible planning, no satisfactory management of land use, no long-run solution to urban problems is possible in the State of California if it continues to add half a million to its population each year (Davis and Styles 1971).
Zero population growth will be reached eventually. I have no doubt of this, nor do I think it is a matter of opinion. In the long run, the population of the earth will be no greater than its carrying capacity, that being a function of the level and style of consumption of humans, the quality of natural systems at the time, and the population size.
We still have a long way to go in convincing some people of this. Last year I was being interviewed by a member of the press for a story on California's population growth. "Tell me," he said, "does California have a carrying capacity?" "Of course," I responded, "but that is not a matter of my opinion, that is a biological fact." I pointed out to him that people might disagree about what California's carrying capacity for humans actually was, but I had no doubt at all that there certainly was one! I suspect he remained skeptical.
It is my opinion that the earth is probably well beyond its carrying capacity now. One device for generating a sense of the earth's carrying capacity is to list the countries one thinks could support populations twice their current numbers for the next 1,000 years. Assume, for the purpose of this exercise, that those population levels are reached within 100 years. If your sense of the world is anything like mine, your list will be very short. If it is, you believe the world is close to or beyond its carrying capacity.
Are any of these statements new? Not really. A decade ago, these same observations could have been made about the effect of population growth on riparian systems. Scientifically, little remains to be done except to document the degradation of natural resources that has occurred in the past ten years. This relative lack of "new" scientific research is another reason it is sometimes difficult to focus attention on population growth.
California might try to manage its riparian resources despite increasing population in a number of ways. Here are some theoretical examples. We could encourage a smaller fraction of the population to utilize the systems. Each person could, on the average, visit them less often. Californians could decide to visit other ecosystems (or use timber from other ecosystems). Or we could expand the number of riparian systems open for human recreation—or for timber production. However, this merely postpones the inevitable day of reckoning with growth. Already more than one million people are turned away annually from over-booked state campgrounds, whether riparian or not.
No, this will not be enough. A concerted effort over time to limit California's numbers must be part of all of our efforts to protect riparian systems.
This is not to assert that any particular resource "runs out" or becomes totally degraded overnight. To the contrary, change is most often gradual, as the choices and our future options become ever more limited.
Of course, achievement of zero population growth alone will not solve our problems. It will not by itself protect any ecosystem, preserve the quality of human life or the wellbeing of the economy. It is not a substitute for wise management and stewardship of resources, but it is an essential complement to and component of such management.
Analyzing Population's Effect on Riparian Systems
Before I tell you what has been done and what needs to be done, let me point out some dilemmas in analyzing the problem and developing solutions.
First, the local population in and around a riparian system is not self-regulating with respect to the carrying capacity of that system, because resources to sustain the population are imported from outside the system. For example, the number of people living near a riverbank is not related to the carrying capacity of that riverbank, because the population can bring in outside resources to satisfy its needs.
Second, the demands on a riparian system are not limited to the demands of the people living near it because resources produced by riparian systems are exported from the system. For example, agriculture responds to demands for goods from outside that system where the goods originate. According to the National Agricultural Lands Study, the harvest of one-third of the cropland in the United States is exported from the country (US Department of Agriculture and Council on Environmental Quality 1981).
Another example of this phenomenon is the National Forest Management Act, which calls for the Executive Branch to establish national targets for timber, wildlife, recreation, and wilderness. These are then apportioned to particular national forests and finally to particular ecosystems or parcels of land. This is not the kind of approach likely to match resource demands with the carrying capacities of natural systems.
Third, population growth comes from two sources, fertility and immigration. Fertility is only indirectly influenced by the public will, through social pressure and some government actions. Immigration is influenced directly only
at certain geographic levels, specifically, by national governments, and only very indirectly at lower levels such as states or communities. Regions wanting to slow or end their population increases have few strong legal foundations on which to do so.
Fourth, those most motivated to be aware of the problems of population growth in its biological diminsions are not the ones best situated to affect policy. It is not surprising that the political leader in California most sensitive to the problems of population growth is Huey Johnson, Secretary of the Resources Agency for the State—someone who has no jurisdiction whatsoever over those factors influencing fertility and migration.
Fifth, the effects of population are gradual and cumulative, just as the degradation of riparian systems is gradual. The expression "population bomb" is an inappropriate metaphor. In a particular crisis, population growth is never seen as the proximate cause and therefore is often ignored completely.
A fine discussion of this problem—population being overlooked as a causal factor—was contained in a recent editorial in "Science" by demographer Kingsley Davis (Davis 1981).
Seldom are public policies constrained only by scientific and engineering limitations; they are also limited, consciously and unconsciously, by social norms. Solutions to the energy problem, for example, usually take one of two forms: to conserve energy, or to increase or at least maintain the total supply . . . A third approach—stopping or reversing population growth—is seldom treated as a part of energy policy . . .
(S)topping or reversing population growth could play a major role in solving the energy problem. When we take into account the environmental problems that heroic efforts to increase the total energy supply will entail, or the human problems that reducing average per capita consumption throughout the world will bring, we conclude that population control is not only a desirable but also a necessary part of any effective energy policy. To "solve" the energy problem otherwise is like fixing a leaky roof by putting more containers on the floor . . .
(W)e still construe energy policy as producing or saving energy for however many people there are, not as producing fewer people so as to give each one as much energy as he or she needs . . .
Yet it is people who use energy. With fewer people, less energy is needed. This may seem obvious, but so far we have tragically postponed acting on it. . . .
My sixth, and final observation, is that the decisions which cause population growth, namely fertility and migration decisions, whether of individuals or governments, are virtually never seen as environmental decisions at all.
Fertility zxdecisions are matters of individual choice. Public policy aspects are the protection of those rights, ensuring (theoretically) that every child is a wanted child, and protecting the health of women and children.
Immigration decisions are made because the individual believes, in most cases correctly, that his or her life will be bettered by the move. Immigration policies are seen as labor policies, components of international relations, fulfillment of commitments to family reunification, and service to refugees.
Still further, migration within the country is seen as a response to economic growth or economic stagnation. Many cities in the Northeast or Midwest see their current population stability or decrease not as a welcome sign of balance but rather as a symptom of serious economic problems.
Response to These Dilemmas
One response to the problems posed by population growth, and a common one, is to try to "buy time". Journalist Hall Gilliam addressed the problem of "Finding Space for All the People".[4] He considered tradeoffs among population growth, urban open space (within cities and as farmland at the fringes), and density, and concluded that those concerned about environmental protection must endorse higher densities of housing. He then broadened his observations with a conclusion paralleling some of my own. To make it more relevant to this discussion, read "riparian system protection" where he uses "open space protection."
. . . (G)reater urban density is no guarantee that regional open-space lands will remain open, even with legal protection. Open-space laws can be changed, and if population pressure becomes intolerable, they will be.
That brings us to the unanswered question: How can unlimited population growth be accommodated in a limited area?
Probably the best we can do now is to buy time to find an answer. Meanwhile, we can house more people in existing urban areas, keep the farmlands intact
[4] Earthwatch column, San Francisco Sunday Examiner/Chronicle, August 30, 1981.
to feed the cities, and pray for insight.
I do not believe that "buying time" is all we can do. The evidence of the last decade is that much can be done to slow population growth, as indicated by these gains:
1) the establishment of Federal and state programs to fund family planning services;
2) the conclusion of the 1972 Commission on Population and the American Future that the nation should welcome and plan for an end to population growth (Commission on Population and the American Future 1972);
3) the 1973 Supreme Court decisions legalizing abortion throughout the country;
4) the rapid and continuing proliferation of movements to control growth in individual communities (whose motives range from the very best to the very worst);
5) the decrease in the number of children born to American couples and the decrease in the number of children they desire;
6) the turnaround in public attitudes towards population as evidenced by a 1977 Gallup poll that found 87% of those polled would rather that the US population not increase any more;
7) the increase in attention given to immigration as a source of half of our country's population growth.
In early 1981, a Governmental task force prepared recommendations for the (Carter) Administration on how to respond to the Global 2000 Report (US Council on Environmental Quality and Department of State 1980). The task force's findings included this recommendation (US Council on Environmental Quality and Department of State 1981):
Population growth in richer countries, though much slower (than in less developed countries), is of concern because consumption of resources per capita . . . is very much higher . . . The United States should develop a national population policy.
In fact, in 1974 then-Governor Reagan stated:
Our country . . . has a special obligation to work toward the stabilization of our own population so as to credibly lead other parts of the world toward population stabilization.
Recommendations
What is needed now is a way to articulate goals linking policies on fertility and immigration with the overall need to end population growth. We need a national population policy.
H.R. 907, introduced into the House of Representatives by Richard Ottinger of New York, declares a policy of comprehensive and coordinated planning for demographic change and establishes the goal of eventual stabilization of the population of the United States by voluntary means. The bill has been co-sponsored by more than two dozen representatives.[5]
Researchers, scientists, and managers of government programs to protect riparian systems do not have direct or indirect control of programs influencing the numbers of people in the country. They can, however, serve as a vital link between those who best understand why we need population stabilization, and those political leaders who can articulate such policies and bring zpg about. I offer as a model the policy statement issued by the Society of American Foresters:[6]
If human populations continue to increase substantially, insatiable demands on forestland resources will occur. The United States has the capacity to provide leadership in this global population challenge—as it has done in the conservation movement. Our legislative measures . . . have established a world standard. Yet these measures treat only the symptoms of uncontrolled population growth. This primary conservation issue has yet to be seriously addressed by the nation . . .
The best science and technology we can devise will not extricate us from the absolute limitations of the carrying capacity of our environment.
Therefore the Society of American Foresters supports a national policy of population stabilization and establishment of an office to coordinate its implementation. While recognizing that
[5] More recently, in October 1981, Senator Mark O. Hatfield of Oregon introduced S.B. 1771, the Global Resource, Environment, and Population Act of 1981. That bill, co-sponsored by Senators Alan Cranston (California), Charles Mathias (Maryland), Slade Gorton (Washington), and Spark Matsunaga (Hawaii), is a stronger version of H.R. 907.
[6] Excerpted from "Statement of the Society of American Foresters" submitted to the Subcommittee on Census and Population, Committee on Post Office and Civil Service, US House of Representatives, May 12, 1981, re: HR 907, to establish a national population policy and Office of Population Policy.
the technical aspects of such a policy are peripheral to the expertise of professional land managers, we also recognize that the long-term effectiveness of our management and conservation efforts depends on the resolution of this major domestic and global challenge.
In this context I wish to offer several recommendations. As scientists, managers, administrators, planners, and concerned public, you are in an advantageous position to define population problems and provide guidance in their solution.
First, professional organizations to which you belong should pass resolutions supporting population stabilization for the United States, endorsing the Ottinger and Hatfield bills or, if the latter is prohibited by your organization's tax status, calling for hearings on the two bills.
Second, you must continue to teach each new generation of Americans about the need to end population growth, because what is obvious to one generation may be forgotten by the next. Population education is not sex education, just as learning how to build, control, and put out a campfire is not the same as learning about the (positive and negative) effects of fire on forest ecosystems.
Third, you must expand research on the carrying capacity of particular ecosystems and on the concept of carrying capacity in general. It is important that you continually stress that "proper management" cannot possibly be enough; stabilization of the demands on a system must accompany management.
Fourth, use the language of goals. You need to say "when we reach zpg . . ." or "the ultimate population of this area will . . ." You must stress the tradeoffs between numbers of people and their consumption or lifestyles.
Fifth, in your professional lives, make recommendations for population stabilization. Raise this theme of the need for zpg in government service, on committees to which you belong, in reports you write to or for government agencies, and in testimony you prepare. Every time there is developed a set of recommendations for action, make sure action on population is among them. Remember that acknowledgment of the need for rapid achievement of zpg will come because those who think about resources point it out.
Sixth, take a public stand endorsing an end to population growth for the country and for the state. The latter is important because we experience the impacts of growth primarily at state and local levels. The former is important because immigration policy, critical in controling growth, is a national concern. Population policy therefore must be established at the national level.
And last, request that the State conduct an analysis of California's long-term carrying capacity, as has been suggested by Secretary of Resources Huey Johnson. You should also examine what the optimum population for California might be. The Resources Agency's report, "Investing for Prosperity" (California Resources Agency 1981), is an excellent model, having specific goals for enhancing California's resources by the year 2000. If only it had had a section on population goals for the state!
Overall, we must all use our commitment the protection of natural systems to be public leaders in making the link between our concerns and the need for population stabilization.
Conclusion
The problem of controlling California's growth, or that of any state, is a difficult because the state does not directly control migration across its borders. We in California much more than our share of foreign immigration to the country and our currently healthy economy attracts migrants from other states. To some extent we still need to take Hal Gilliam's advice to accommodate growth as best we can, thereby buying time to find a real solution.
In welcoming participants to the previously-mentioned conference on California's growth held in 1971, Bob Moretti, Speaker of the Assembly, stated that the Assembly was interested in developing:
. . . some aproaches to legislation which will influence state population growth and distribution. Even though the personal decisions of individuals will always govern where they live . . . I do not agree that we are powerless to influence them through legislation. . . . (F)ederal and state legislation in this field . . . has contributed significantly to changed attitudes and population distribution.
We can no longer accept the proposition that all growth is good. In fact the quality of our life and our economic well-being may in the future depend to a great extent on more effective management of growth. What we need to seek is a balance between our resources of air, water, and open space and our population growth.
These are tough problems, and we are not so naive as to believe that there are any simple answers. I believe, however, that unless we deal with them, our efforts to control smog, clean up our waters, and open spaces [and here one might add 'protect and enhance the
state's riparian systems'] will become mere stopgaps.
Mr. Moretti's comments are as true today as they were ten years ago when he said them.
Literature Cited
Almand, J.D., and W.B. Krohn. 1979. Position paper: the position of the Bureau of Land Management on the protection and management of riparian ecosystems. p. 359–361. In : R.R. Johnson and J.F. McCormack (tech. coord.). Strategies for protection and management of floodplain wetlands and other riparian ecosystems. [Callaway Gardens, Georgia, December 11–13, 1978]. 410 p. USDA Forest Service GTR-WO-12, Washington, D.C.
California Resources Agency. 1981. Investing for prosperity: enhancing California's resources to meet human and economic needs. California Resources Agency, Sacramento.
Commission on Population and the American Future. 1972. Population and the American future. Report of the Commission on Population Growth and the American Future. US Government Printing Office.
Davis, Kingsley. 1981. It is people who use energy. Science 211(4481):439.
Davis, K., and F.G. Styles (ed.). 1971. California's twenty million: research contributions to population policy. Population Monograph Series, No. 10. 349 p. Institute of International Studies, University of California, Berkeley.
US Council on Environmental Quality. 1978. The Ninth Annual Report of the Council on Environmental Quality. 599 p. US Government Printing Office, Washington, D.C.
US Council on Environmental Quality and the Department of State. 1980. The global 2000 report to the President: entering the Twenty-first Century. US Government Printing Office.
US Council on Environmental Quality and the Department of State. 1981. Global future: time to act. Report to the President on global resources, environment and population. US Government Printing Office.
US Department of Agriculture and Council on Environmental Quality. 1981. National agricultural lands study. US Government Printing Office.
USDA Forest Service. 1979. Report of a USDA Forest Service riparian study task force on riparian policies and practices in the National Forest system. 15 p. Unpublished manuscript.
Warner, R.E. 1979. California riparian study program. 177 p. California Department of Fish and Game, Planning Branch, Sacramento, Calif.