Part II
Prehistoric Migrations
There is a large body of literature in biogeography that attempts to deduce prehistoric migrations of taxa solely from their present distribution patterns. Strangely, such deductive reconstructions, by long tradition, have come to be called historical plant geography, although as Stott (1981) pointed out, they are not generally based on any genuine historical evidence. Some of these reconstructions assume that through geologic time plant ranges have simply expanded and contracted around centers of origin or refuge. Where present distributions of taxa are disjunct, there has been endless contention between proponents of two polar a priori explanations: long-range dispersal versus fragmentation of former continuous ranges. Less simplistic deductions based on consideration of the biology of the taxa and the known paleoecology of the region are more plausible and interesting. In the absence of any actual evidence of past distributions, however, there is no way to confirm the time or spatial scale of the postulated migrations. A scholar dealing with a predetermined cast of characters without a fossil record may have no alternative but to speculate about their migrations. Here, the cast of characters is not predetermined; as in Part I, case histories will be chosen for which there is actual time depth in the evidence.
The transition from written history to prehistory requires a change in approach. It is no longer feasible to organize cases under habitat types. In the fossil record, environment is not a matter of observation but of interpretation. Reconstruction of paleoenvironments is not a starting point but a goal of research. The starting point here will be fossil assemblages from particular geologic time spans and particular regions. Little attention will be given to inferred paleoenvironments. This is certainly a subject of profound interest in its own right. For purposes of tracing plant migrations, however,
it is more appropriate to deal with the plant taxa directly. Using paleoenvironments inferred from fossil distributions to explain fossil distributions is logically quite circular.
The chronology will be worked backward because the more recent periods have the most complete and least problematic fossil record. While moving forward from the origin of seed plants in the Paleozoic might appear more straightforward, it would not actually give a more coherent story, simply because the record is too discontinuous.
6
Last Glacial
And Holocene
This period offers the most readable chapter in all of paleobotany. Any fossil evidence, however, involves formidable problems not shared with modern historical evidence. These problems have been carefully examined by Davis (1978) and Watts (1978). Only a few of the more intractable ones will be briefly noted here.
Identification of Taxa . The basic units of the fossil record are not ordinarily whole plants or assemblages of organs, as on herbarium specimens, but disarticulated parts. The great bulk of the seed plant record consists of pollen grains, the domain of palynology. The most abundant macrofossils are usually individual leaves or leaflets. Plant fossils of this period are generally considered to belong to living species, but their pollen and leaves have often been identified only to genus or family. A great deal of talent and effort are currently being devoted to better identification, partly by use of scanning electron microscopy.
Dating . The pioneer palynologists made an assumption that is no longer considered tenable, namely, that during Late Glacial and Holocene time, major vegetation changes were synchronous over large regions, perhaps globally, due to changing climate. Before absolute 14 C dating was available, changes in fossil floras were used to define time levels and thus to correlate dating between different sites and regions. This led to circular reinforcement of the idea of synchronous climatic control of plant migration. Forunately, development of isotopic dating has opened the way for a true chronology not derived from changes in fossil floras.
Displacement . Transport of pollen and other plant parts by wind and water may leave fossil records far outside the range of the species. Water-worn fossils can sometimes be recognized, but wind-borne pollen is not altered by its journey. Thus, if fossil pollen of a taxon is present at a site in low frequency, it can be interpreted as either an abundant species far away or a rare one nearby. For example, in eastern North America, beech, Fagus grandifolia , is recorded during the Late Glacial by macrofossils from two sites in the lower Mississippi Valley and by pollen from several sites farther east. The pollen is present in extremely low frequencies and is usually interpreted as having come from a few distant, highly localized refugia in the lower Mississippi Valley or elsewhere. However, Bennett (1985) prefers to believe that beech was very widespread but very rare all across southeastern North America. There are not enough Late Glacial pollen sites yet known in the region to resolve this question. Discrimination between distance and abundance as controls of pollen frequency is possible when many sites in different parts of a region can be compared. Uniform low frequencies of a given pollen type suggest a background pollen rain from a distant source, while locally variable frequencies suggest presence within the region. This deduction is strengthened when formerly constantly low frequencies rise abruptly in a geographic sequence, which evidently documents local arrival of an advancing species border.
Palynologists face other difficult problems in attempting to translate pollen frequencies into vegetational reconstructions because of great differences between species in the quantity of pollen produced and preserved. In its naive early days, palynology seriously underestimated the difficulty in translating fossil pollen data into geographic patterning of plant species and their environments. Dawning awareness of this difficulty has led to admirable advances in research techniques and interpretation of data. Whatever its remaining imperfections, modern palynology is infinitely better for reconstructing prehistoric plant migrations than the untestable speculations that prevail in the absence of a fossil record.
Northwestern Europe
(Bell 1969; Bertsch 1953; Beug 1982; Butzer 1971; Edwards and Hirons 1984; Frenzel 1968; Godwin 1956; Hammen et al. 1971; Huntley and Birks 1983; Iversen 1949, 1960; 1964; Kral 1972; Markgraf 1972; Pennington 1969; Prentice 1983; Ralska-Jasiewiczowa 1983; Tallantine 1972)
The reconstruction of plant migrations in northwestern Europe during this time span forms a classic chapter in paleobotany. It is the work of a large
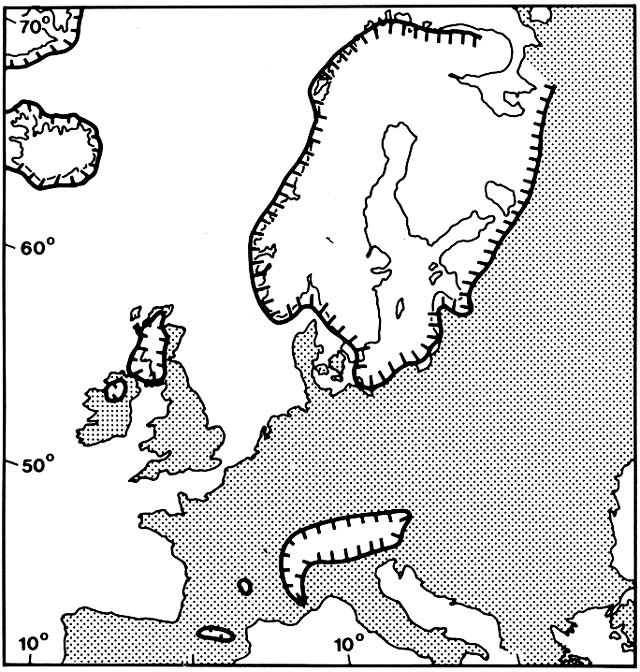
Figure 7. Areas of Northwestern Europe Covered by Glacier 12,500 Years Ago. Modern coastlines are shown
for orientation, but the sea then stood far below its present level. (Adapted from Huntley and Birks, 1983)
corps of scholars in various countries. They had a magnificent fossil record in the abundant bog and lake deposits of the region. Also, the simplicity of the forest flora of the region aided accurate identification of fossils and ecological interpretation. The same tree genera that are represented elsewhere in the northern hemisphere by multiple species are here represented by single or few species.
Late Glacial: Dryas I
The beginning of the Late Glacial, which terminated the Weichsel glaciation, is conventionally dated at about 14,000 B.P. (before the present) (Table 1). The great continental ice sheet that had formed about 60,000 B.P. began to retreat from its Full Glacial maximum. In the Late Glacial, it still covered almost all of Fennoscandia and much of the northern British Isles (fig. 7). Sea levels were still low; the North Sea and the English Channel were dry land. South of the continental ice sheet, mountain glaciers were extensive in all the ranges as far south as Spain, Italy, and Greece. Boreal forests were extensive in eastern Europe, but northwestern Europe had only scattered patches of sparse, open woodland. Barren outwash plains, flooded periodically during early summer, were extensive; they were sources of massive wind-borne loess deposits. Much of the region was dominated by grasslands where soil processes were similar to arctic tundras, with solifluction and frost heaving above permafrost.
The Dryas I vegetation, however, has no exact modern analogue, either in the arctic tundras or in the cold, dry steppes of central Eurasia. Its component species have since gone their separate ways to a variety of regions and habitats. The woodland trees, species of Picea, Larix, Pinus, Betula , and Populus , are now found in the boreal forest. Some shrub and herb species have retreated to arctic and alpine tundras, for example, Dryas octopetala, Betula nana, Salix herbacea , and Koenigia islandica . Many others are now pioneers of seashores, cliffs, stream gravels, and other naturally disturbed sites over a wide climatic range, for example, Juniperus communis, Ephedra distachya, Suaeda maritima, Triglochin maritima, Hippophae rhamnoides , and Helianthemum oelandicum . Many members of the Dryas I herb flora are now common weeds, for example, Rumex acetosella, Chenopodium album, Plantago major, P. lanceolata, Daucus carota , and Centaurea cyanus . During Dryas I, periglacial processes provided the disturbed, exposed ground needed by a heterogeneous assemblage of pioneer plants. The Dryas I vegetation supported a great mass of great herbivores, including woolly mammoths, woolly rhinos, muskoxen, reindeer, and the Paleolithic hunters that pursued them.
|
Allerød Interstadial
The brief warmer period during the Allerød Interstadial had rapid glacial melting. Sea level rose to within about 40 m below present level, but the North Sea area remained land and the Baltic region had a great freshwater lake. Many small lakes began accumulating organic sediments over the former inorganic deposits; they were colonized by aquatic plants, such as Myriophyllum alterniflorum , probably brought north by waterfowl. Dryas, Juniperus , and other pioneers advanced into deglaciated territory, but gained less territory than they lost to northward-advancing trees. Tree birches, Betula pubescens and B. pendula , spread over large, formerly treeless areas as far as Britain and Denmark. Woodlands of Populus tremula and Pinus sylvestris expanded in southern Germany and the Alps, where the tree line rose to only about
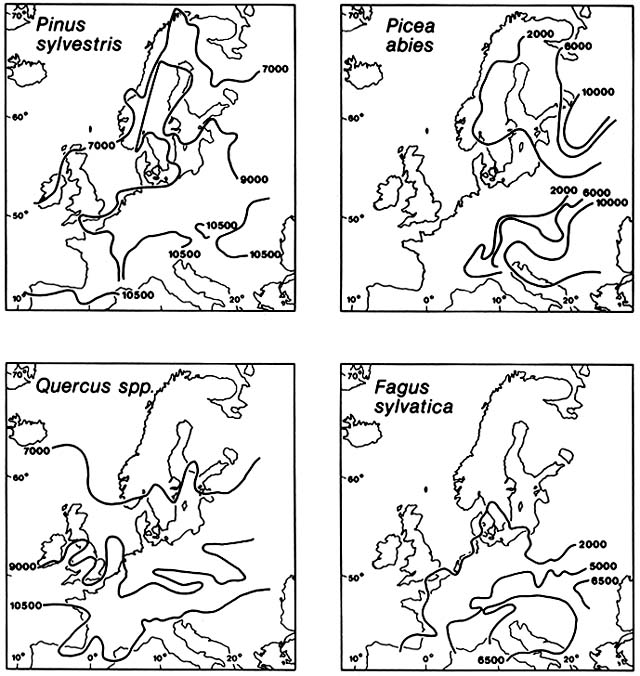
Figure 8. Postglacial Immigration of Selected Trees into Northwestern Europe. Advancing fronts are shown
at specified numbers of years before the present. Subsequent losses of territory are not shown. During the early
Postglacial, Britain was not yet an island. Each tree species migrated individually at its own rate and along a
peculiar pathway. Migration rates were commonly so fast that human inhabitants of the region must have been
well aware that the species borders were moving. (Adapted from Huntley and Birks, 1983)
500 m below its present level. In the Carpathians, Picea abies expanded its range. Woolly mammoths and rhinos became extinct during the Allerød, while muskoxen retreated to the far north. Some reindeer survived in the region and moose increased in abundance, often being the main game of the Paleolithic hunters.
In deposits of late Allerød age, charcoal horizons were associated with high frequency of pollen of fireweed, Epilobium angustifolium . Some scholars have suggested that extinction of the large herbivores and deliberate forest burning can be attributed to the Paleolithic Late Magdalenian peoples. Advance of the forests during the Allerød must have reduced the habitat of the large herbivores and made them vulnerable to overhunting. It is also possible that climatic cooling at the end of the Allerød led to death of the forests and the conflagrations recorded by charcoal horizons.
Dryas II
During the Dryas II glacial stadial, glaciers readvanced, sea level dropped, and forests were replaced by tundra and steppe over much of the region. This final Weichsel glacial stadial lasted about 1,000 years before its abrupt end.
Holocene: Preboreal
During the first subdivision of the Holocene Epoch, the Preboreal, the continental glacier melted back to separate residuals in northern Sweden and Norway. Glaciers in the Alps retreated to about their present extent. Lakes as far north as the Baltic region were rapidly colonized by Cladium mariscus and other warmth-requiring aquatics. Juniperus communis and other pioneer shrubs expanded rapidly north of the Alps, in the northern British Isles and northern Scandinavia. Juniper dominance was very brief before it was over-whelmed by advancing tree birches and poplars, such as Betula pubescens, B. pendula , and Populus tremula . From scattered Dryas II relic stands, these expanded to regional woodlands. Pinus sylvestris began moving northward from the Alps, but had not yet caught up with the birches. Picea abies began spreading slowly westward in the southeastern Alps and Carpathians, but it remained in the mountains (fig. 8), leaving the Danube and Pripet lowlands uninvaded. During the Preboreal, the tree line in the Alps rose to approximately its modern level.
Warmth-requiring deciduous hardwoods, which had probably become extinct north of the Alps during the Weichsel, began to reinvade. Of these, hazel, Corylus avellana , moved the fastest, advancing from Italy northwestward
across France to Britain, which was not yet an island. Hazel then looped eastward across the low countries to the Baltic. By 9,500 B.P. , hazel was approaching its present limits in northern Scotland and Scandinavia. In parts of northern Europe, hazel had outrun pine. During the same time span, deciduous Quercus spread from Spain and Italy across western France to southwestern England, while Alnus, Tilia , and Ulmus were still advancing through France. By the end of the Preboreal, the open steppe and tundra plants had been driven back by the advancing forests and woodlands to scattered patches. Reindeer were gone from most of the region.
Boreal
Pinus sylvestris caught up with the boreal tree birches and poplars, driving them back to scattered remnant patches, and was approaching its present northern limits in Britain and Scandinavia. At the same time, pine was losing ground over much of the region to advancing temperate hardwoods. Hazel continued to move eastward into Poland and also pushed northward into deglaciated parts of Fennoscandia. The oaks, Quercus , continued to move rapidly northward through Britain and Ireland and also up the Danube and across central Europe. Lindens, elms, and ash trees, Tilia, Ulmus , and Fraxinus spp. had moved past the Alps more slowly and were still advancing across France and Germany far south of their present limits at the end of the Boreal. Meanwhile, alders, Alnus spp., were invading poorly drained areas. An undetermined species of alder made a tentative advance into northwestern France early in the Boreal, but this migration stalled for unknown reasons. At the same time, far to the east both Alnus glutinosa and A. incana pushed north from southeastern Europe clear into Fennoscandia. Picea abies continued to expand slowly westward in the mountains of central Europe but did not move out onto the plains. Abies alba finally began moving northward into the Alps, presumably coming from a glacial refuge in the Appenines.
Atlantic
During the Atlantic period, deglaciation of Fennoscandia was completed and eustatic sea level approached the present level. Britain and Ireland became islands. Encroachment of the rising sea onto the continental shelf must have extended the mild, humid maritime climate eastward. Hornbeam, Carpinus betulus , was restricted to Italy and the Balkans at the beginning of the Atlantic. By the end of the period, hornbeam had spread northward through Poland to the Baltic, joining the mixed deciduous hardwood forest. Meanwhile, alders continued to spread into deglaciated territory, especially in swampy carrs and fens. During the Atlantic, alders spread northward through
Fennoscandia to the Arctic Ocean and westward from the Baltic Sea into the Low Countries and England, then northward to Scotland, Ireland, the Faroes, and Iceland.
Another deciduous hardwood, beech, Fagus sylvatica , invaded the region more slowly around both flanks of the Alps. Early in the Atlantic, beech advanced from Italy into southern France and from the Balkans up the Danube Valley. By late Atlantic time, the eastern invasion had rounded the Alps and was moving westward through the central European mountains. Beech advanced selectively, favoring chalk soils and leaving the mixed oak forest in mesic sites.
Meanwhile, pine, birches, and other pioneer trees continued to be squeezed into edaphically and climatically marginal sites. Of all the Holocene subdivisions, the Atlantic had the most continuous expanse of late successional hardwood forest. In certain places, however, such as the Pennine plateaux of southern Britain, extensive blanket peat bogs, with Sphagnum moss and Eriophorum sedge, began replacing former hardwood forest. As for fir and spruce, during the Atlantic, Abies alba reached its present northern limits along the Rhine and began moving into the eastern Alps, where it met and overlapped with Picea abies moving in the opposite direction. After 3,000 years in the eastern Alps, Picea finally crossed the Rhine into the western Alps during Atlantic time. At the same time, a separate migration of Picea abies , presumably from a glacial refuge in Russia, reached the Baltic from the east.
Subboreal
During the Subboreal, there was large-scale replacement of forest by blanket bogs, heathland, bracken, and grassland. At the same time, beech finally moved out of the central European mountains and advanced at a startling rate across Poland to the Baltic Sea and across Germany and France to England. Meanwhile, the border of hornbeam remained nearly stationary in southeastern Europe, but hornbeam advanced from northern Poland westward across northern Germany. Spruce, Picea abies , crossed the Baltic into Scandinavia, but remained rare and local there during the Subboreal. Fir, Abies alba , completed its migration in the central European mountains, even surpassing its present borders in places, including the Alps, where it grew higher than the present timberline.
Subatlantic
Blanket bogs have continued to spread to the present time at the expense of woodland, especially in wet coastal and cool mountain regions. In general, the fossil record suggests fluctuating, patchy, local vegetational changes,
largely attributable to human activities, rather than progressive changes in species ranges. There are a few exceptions. After a long history of relatively slow advance, hornbeam began advancing rapidly about 2,000 B.P. Within 500 years the species advanced from western Germany across all of France to the Bay of Biscay. During the first half of the Subatlantic, spruce essentially completed its immigration into Scandinavia.
Changing Interpretation of Postglacial Migrations
In the early days of palynology, it was taken for granted that regional vegetational changes were controlled by climatic changes. Early Postglacial advances of tree species were thought to have been restrained by gradually rising temperature, allowing cold-tolerant boreal species to precede the warmth-demanding species. The northern European climate was considered to have improved to a so-called optimum in the Atlantic period, followed in the Subboreal by deterioration, as evidenced by decline in abundance or retreat of certain species. Essentially, the whole sequence was interpreted as a series of different climax vegetation types, each in equilibrium with changing climate. The conceptual model did not allow for successional or migrational lag.
There is no doubt that drastic climatic changes during the Allerød and again at the beginning of the Holocene set off great plant migrations. However, the assumption that the moving species borders were constantly in equilibrium with climatic controls was inevitably challenged. Bertsch (1953) pointed out a possible spurious correlation between cold tolerance and sequence of immigration of the dominant tree species. The most cold-tolerant trees, such as Pinus, Betula , and Alnus , are quicker to mature and more widely dispersed than the most warmth-requiring ones, such as Ulmus, Tilia, Quercus , and Fagus . Iversen (1960) reiterated this point and also noted that, because the boreal species are cold tolerant, they would have survived the Weichsel glacial at higher latitudes and thus, other things being equal, would have arrived first in Postglacial time. Furthermore, the first Postglacial immigrants were pioneer species, capable of invading open habitats. The later arriving dominants, although slower to mature and less dispersible, are longer lived and more shade tolerant; even under a constant climate, they might be expected to eventually replace the pioneers. Iversen (1964) later suggested that some vegetational changes after Atlantic time, which had been attributed to climatic deterioration, were due to gradual leaching of immature soils derived from base-rich glacial drift and also to accumulation of acid peat. In northern Europe, the possibility that forests can be successionally replaced by blanket bogs and heaths is now taken seriously by many ecologists.
It is possible that in early Holocene time, climate had changed so abruptly
from the glacial climate that there was a period of free migration before species could fill their new potential areas. During this free migration, location of the moving borders would have been largely controlled by how far seed was dispersed and how long it took for new colonies to mature and produce the next wave of seed. Many of the advancing tree species spread at almost incredible rates; their advances must have been very obvious to people inhabiting the region. Almost all of the dominant trees accomplished much of their advance at rates of over 300 m/year, averaged over centuries and millennia. Pinus, Corylus, Alnus, Ulmus, Acer, and Carpinus advanced at rates of over 1 km/year, averaged over centuries. Thus, all of these advanced at rates of many kilometers per generation. Explaining location of their borders does not require invoking climatic or other environmental controls. Rather, it demands long-range dispersal agents. North-flowing rivers and birds were probably crucial. Human dispersal probably was also, particularly in species with heavy, edible seeds, such as Pinus, Corylus, Quercus, and Fagus . Early Postglacial Europe was inhabited by so-called Mesolithic peoples. Unlike the specialized Paleolithic big game hunters of the glacial steppes and tundras, the Mesolithic cultures had a more diversified food base, including fishing, hunting deer, and gathering nuts and other vegetable foods. Archaeological caches of hazel and other nuts show they were commonly transported by the people.
Later, with the arrival in northern Europe of Neolithic pastoral and agricultural peoples, evidence of anthropogenic effects on plant distribution becomes much clearer. This began in the Subboreal period. Before 14 C dating the end of the so-called Atlantic optimum and the beginning of the Subboreal was defined by a change in abundance of pollen of certain tree species, particularly an abrupt drop in Ulmus . This so-called elm fall was accompanied by subtler and less consistent changes in abundance of other species for which rather vague climatic causes were suggested. Subsequently, 14 C dating revealed that the elm fall and associated changes in pollen of other species were not synchronous throughout the region, as had been assumed, but varied by several hundred years between different places. Moreover, it was found that the pollen record did not show unidirectional changes but rather complex local fluctuations, with forest trees alternating with birches and other pioneer trees. Along with increases in pioneer trees came resurgence of pioneer herbs that had disappeared from the fossil record in early Postglacial time, such as Rumex spp., Chenopodium spp., Plantago lanceolata, and Centaurea cyanus . Presumably, these had survived in naturally open coastal and riparian habitats as Postglacial forests took over most of the region and were preadapted to become weeds when the forests were opened by Neolithic pastoral and agricultural clearings. Subboreal pollen diagrams commonly show the first appearance of domesticated cereal crops at the same levels as resurgence of native pioneer trees and weeds.
Causes of the elm fall are still something of a puzzle. It is questionable whether Neolithic shifting cultivation in early Subboreal time was sufficiently extensive to cause a decline of the magnitude recorded. Palynologists and archaeologists, particularly in Scandinavia and Great Britain, have proposed that lopping of elm branches for fodder for cattle was largely responsible for the elm fall. Arrival of the fungus responsible for Dutch elm disease, Ceratocystis ulmi , has also been suggested. Huntley and Birks (1983) have neatly combined all three hypotheses. Spread of agriculture and the fungus may have coincided because injury to elms by Neolithic felling and lopping would have made the trees more susceptible to attack by bark beetles that transmit the fungal spores.
The puzzling acceleration during the Subboreal of the advance of beech, Fagus sylvatica , has also been attributed to human agency. Unlike its North American congener, the European beech is not usually able to invade undisturbed forest. Its sudden expansion coincides in time with the elm fall and the beginning of shifting cultivation. Later in the Subboreal, with the beginning of the Iron Age when plow farming moved onto heavier soils, beech may have expanded on abandoned upland fields on chalk and limestone.
It is widely recognized that northern Europe has had very little truly natural vegetation, in which species distributions have been under direct climatic control, since the Atlantic period. For Subboreal and later time, sorting out any signals of climatic change from the other variables affecting plant geography is a baffling problem. Some scholars, including Beug (1982) and Prentice (1983), are optimistic that this can eventually be accomplished, but it has not yet been done.
A special problem with attributing plant migrations to climatic change arises with freshwater aquatics. Some of these were much more extensive and abundant in deglaciated territory during early Holocene time than now, for example, Cladium mariscus, Trapa natans, Najas marina, N. flexilis, Ceratophyllum demersum, and C. submersum . Their retreats and local extinctions have been cited as evidence of climatic deterioration since the Atlantic optimum. It is curious that such retreats should be concentrated among aquatics, which are relatively indifferent to climatic gradients. Also, their losses of territory have been due to thinning out of the density of sites rather than to general southward displacement of the border of the gross ranges. Some of the winking out of colonies may be due to limnological changes as new glacial lakes became older.
Eastern And Midwestern North America
(Black and Bliss 1980; Davis 1965, 1978, 1981a, b, 1983; Dean et al. 1984; H. Delcourt 1979; P. Delcourt 1980; Delcourt and Delcourt 1977, 1981,
1984; Delcourt et al. 1983; Grimm 1983; Ritchie 1976; Vesper and Stuckey 1977; Watts 1983; Wright 1971, 1976)
After early naive attempts to find parallels with the European Late Glacial and Holocene climatic and vegetational sequence, North American workers have developed a story that is in many ways different and more complex.
Full Glacial
During the Wisconsinan Full Glacial, from about 25,000 to 15,000 B.P. , the continental ice sheet covered half of the North American continent, extending from the northern Rocky Mountains across what later became the Great Lakes to the Atlantic Ocean, where the southern margin on Long Island reached the latitude of Madrid and Naples (fig. 9). Instead of the great European tundra or steppe grassland, there was a very narrow and probably discontinuous treeless belt along the ice margin. Scattered mountain glaciers and alpine tundra extended far south into the Appalachian Mountains. Unglaciated North America east of the Rockies was mostly dominated by conifers now found in the boreal forest, although not in the same associations. Picea and Larix were dominant over a great area west of the Appalachians, and Abies on both flanks of those mountains. Unlike the modern boreal forest, much of the interior conifer forest, especially in the north, had no pine component. Pinus banksiana was present in parts of the Midwest and was dominant in much of the southeast, especially on a coastal plain that was much broader than now because of lower sea level. Much of Florida had dry scrub, savannas, and active dunes. A few hardier genera of deciduous hardwoods—Populus, Alnus, Betula, Carya, and Quercus —were widely associated with the dominant conifers, but the complex of broadleaf trees that dominate mature, mesic forests today were generally absent. Only recently have fossils of these from the Full Glacial been identified in the lower Mississippi Valley and eastward; presumably the species survived in especially favorable microhabitats, such as warm, south-facing gorges.
Late Glacial
During the Late Glacial, from about 15,000 to 10,000 B.P. , ice retreat began and continued without any interruption comparable to the Dryas II Advance in Europe. The deglaciated area was evidently initially colonized by pioneers from the formerly narrow tundra beyond the ice margin: many sedges, a few grasses, and several shrubs, including Salix herbacea, Vaccinium uliginosum, and Dryas integrifolia, a nitrogen fixer. In New England, it was
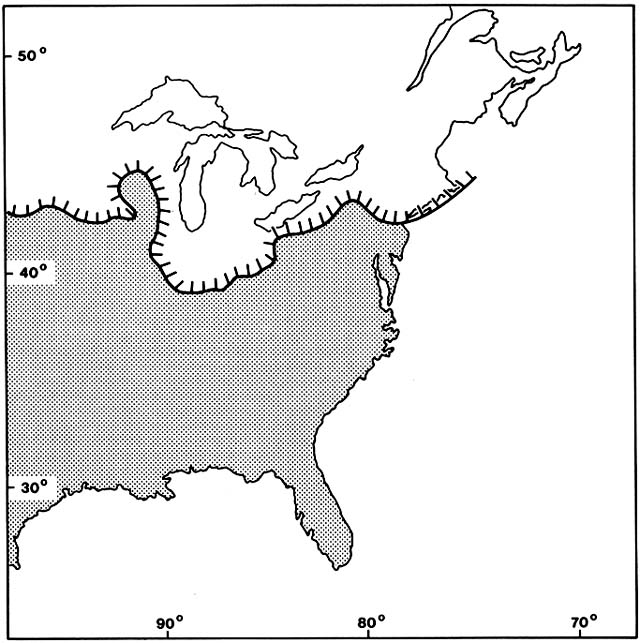
Figure 9. Areas of Eastern North America Covered by Ice During the Last Glacial. (Adapted from Davis, 1983)
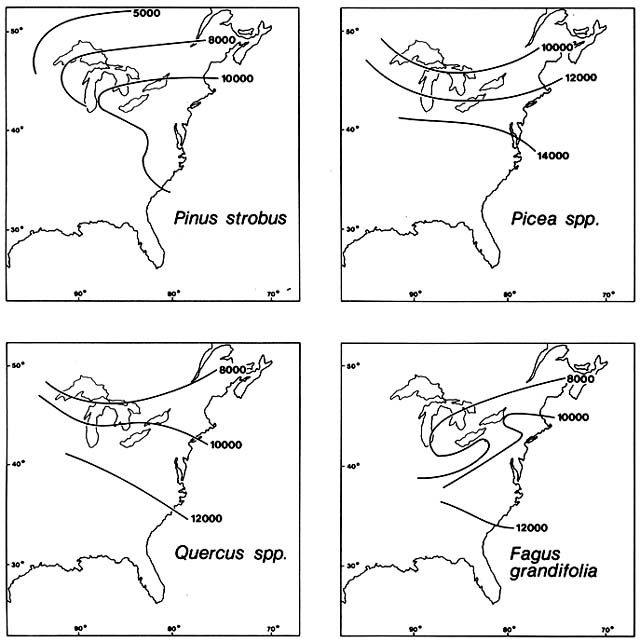
Figure 10. Postglacial Immigration of Selected Trees into Northeastern North America. Advancing fronts are
shown at specified numbers of years before the present. Subsequent losses of territory are not shown. As in Europe,
species migrated individually, not in fixed associations. (Adapted from Davis, 1983)
about 2,000 years before the deglaciated area was invaded by forest; in the Great Lakes region, the tundra phase was evidently much briefer. With the advance of the forest, Dryas and other pioneer colonists of the deglaciated territory retreated to arctic–alpine ranges, leaving isolated relics in the forest on scree and stream gravels.
The conifers that comprise the modern boreal forest migrated individually during the Late Glacial. Generally, spruce was the first to invade tundra on deglaciated territory, with Picea glauca usually ahead of P. mariana and P. rubens. Picea moved northward rapidly west of the Appalachians, reaching the Canadian prairie provinces, Lake Michigan, and Lake Erie by about 13,000 B.P. At the same time, Picea moved upward in elevation in the Appalachians, where the treeless tundra barrier to cross-mountain migration was broken about 12,500 B.P. Having crossed the mountains, Picea moved rapidly northeastward through New York and southern New England, reaching New Hampshire by 11,000 B.P. (fig. 10). Larix laricina , the tamarack, moved northward into the Great Lakes region close behind spruce; tamarack then looped northeastward around the northern flank of the Appalachians. Meanwhile, in the southeast, Pinus banksiana and Abies , probably A. balsamea , moved northward east of the Appalachians, reaching Chesapeake Bay by about 14,000 B.P. , southern New England by about 12,000 B.P. , and northern New England by about 10,000 B.P. By then, they had also branched northwestward through New York into the Great Lakes region, where Pinus banksiana populations from east and west of the Appalachians may have joined.
By the end of the Late Glacial, all these boreal conifers had fairly straight northern borders running west to east from Lake Superior to the St. Lawrence. By then, they had extensively overlapping ranges. Along with boreal hardwoods, Populus balsamifera, P. tremuloides, Betula papyrifera, and Alnus rugosa , they were in some areas forming associations like the modern boreal forest. As they advanced northward, the boreal trees began losing territory in the south.
Two nonboreal conifers, white pine, Pinus strobus , and hemlock, Tsuga canadensis , were moving northeastward and northwestward from glacial refuges in the southern Appalachian foothills or coastal plain. Before the end of the Late Glacial, both were in New England and the southern Great Lakes region.
Full Glacial refuges of many temperate deciduous hardwoods probably lay closer to the Gulf of Mexico. By 13,000 B.P. , various species of Quercus, Ulmus, Acer, Carya, Fraxinus, and Ostrya or Carpinus or both were moving rapidly northward along both sides of the Appalachians. By 10,000 B.P. , most of them were in the southern Great Lakes region and southern New England. Carya moved faster in the west, where it had reached nearly its modern limits by the end of the Late Glacial, while it had only reached the
Carolinas in the east. Fagus evidently remained east of the Appalachians and moved more slowly; by 13,000 B.P. it had reached Georgia, and by 10,000 B.P. it was in the Chesapeake Bay region. Castanea had not yet put in its appearance.
Deglaciated territory was colonized very rapidly by freshwater aquatic plants, probably due to dispersal by migratory waterfowl. Fossils of various species of Myriophyllum, Nuphar, Nymphaea, Potamogeton, Sagittaria, and Typha are known from Full Glacial time from a wide area of the southeastern United States. Fossils of most are also known from Full Glacial time from unglaciated regions in the southwestern United States. Fossils of all these taxa with 14 C dates between 15,000 and 10,000 B.P. are known from deglaciated territory between the Rockies and New England.
Holocene Epoch
By the beginning of the Holocene, about 10,000 B.P. , spruce was losing ground in the south faster than its northward advance. The spruce belt was being compressed into a narrow band close to the retreating ice and the glacial lakes; isolated mountain outposts were left behind at progressively higher elevations. By 8,000 B.P. the retreating southern and lower mountain borders stabilized near their present positions, while the northern advance accelerated, with the rapid final collapse of the ice sheet at about 7,000 B.P. In some arctic regions, the early Holocene advance of spruce carried it well beyond the present tree line. Postglacial fluctuations of the arctic tree line, the tundra—taiga border, have been dramatic in both Alaska and Canada; in places it has moved over 100 km north and south of the present tree line. Data on these displacements are very spotty in space and time; they do not appear to have been synchronous in different regions. There is also evidence of complex Postglacial fluctuations of the alpine timberline on mountains in New England. The other boreal forest trees generally lagged behind spruce in both advancing and retreating. Like spruce, they lost ground in the south to the general advance of temperate hardwoods, but held onto some relic patches in bogs, sand barrens, and burns and along streambanks and lakeshores.
After 10,000 B.P. , the nonboreal conifers, Pinus strobus and Tsuga canadensis , continued their northwestward advance through the Great Lakes region. By 7,000 B.P. , white pine was entering eastern Minnesota; by 5,000 B.P. it had advanced beyond its present limits in Ontario. Hemlock followed more slowly, reaching eastern Wisconsin about 5,000 B.P. By then, both species grew 350 m higher in the mountains of New England than now. At about 4,800 B.P. , hemlock pollen fell abruptly to about 10% of its former abundance all over eastern North America in an area of about 10 million km2 . After the
hemlock fall, there was an increase in pollen of birch and other successional species, indicating that many hemlock trees had died, not just ceased producing cones. After about 1,000 years of low abundance, hemlock began increasing and regained its approximate former abundance by 3,000 B.P.
During early Postglacial time, the temperate hardwoods continued their advances at varying rates. In eastern Canada, present northern borders of Quercus were approached by 8,000 B.P. , of Ulmus and Acer saccharum by about 6,000 B.P. , and of Carya and Fagus by about 4,000 B.P. While advancing northeastward through New England into eastern Canada, Fagus also advanced laterally westward, finally entering eastern Wisconsin after 4,000 B.P. Chestnut, Castanea dentata , appeared in the fossil record in the southern Appalachians by 8,000 B.P. and spread northward very slowly, reaching Pennsylvania by about 5,000 B.P. and southern New England by about 2,000 B.P. Its role in what is generally regarded as the regional forest primeval was thus brief.
In the Midwest and Canadian prairie provinces, some of the territory abandoned by the boreal forest was not wrested away by hardwood forest, but was instead taken over by a mosaic of prairie, oak savanna, and open woodland. The pattern of the mosaic may have been related to topography and fire frequency. The details are obscure because wind-pollinated prairie grasses cannot be separately identified and because most other prairie herbs are insect pollinated and rarely recorded as fossils. Much of the fossil evidence consists merely of changes in the proportion of tree and herb pollen. It seems, however, that prairie and savanna expanded rapidly in the region after 10,000 B.P. By 9,000 B.P. , the prairie—forest ecotone may have occupied about the same region as it did at the time of white settlement. In Minnesota, however, there was evidently a temporary eastward advance of the prairie after 9,000 B.P. for about 100 km. This advance ended about 5,000 B.P. , followed by an increase in savanna, with Quercus macrocarpa , and woodland. It is not clear how much of this middle Holocene change in Minnesota vegetation was due to changes in gross species ranges and how much was simply a change in local abundance.
Problems of Interpretation
As in Europe, pioneer North American palynologists generally assumed that changing Postglacial vegetation was controlled by changing climate. Davis (1965) pointed out the ironic contrast between the types of explanations traditionally sought for paleodistributions and for modern species distributions. Paleoecologists tend to invoke control by unknown past climates, whereas few investigators have even attempted to establish relationships between present species distributions and known climatic gradients. Present
distributions have commonly been explained by invoking so-called historical factors, that is, residual effects of unknown past distributions. In North America as in Europe, it is now generally recognized that Postglacial plant migrations did not necessarily keep up with climatic change, but may have been temporarily shaped by successional and migrational lags.
Edaphic conditions in recently deglaciated terrain obviously would have acted as a selective brake on colonizing species. In Chapter 1, it was noted that on historically deglaciated terrain, Picea colonization was delayed for decades until edaphic conditions on the raw moraines had changed, partly due to nitrogen-fixing pioneers, including Dryas and Alnus . A relay floristics succession cannot, however, be invoked as a general explanation of the sequence of species migration in Late Glacial and later time. For example, the millennia that elapsed between deglaciation of New England and forest invasion are far too long. Also, Davis (1983) pointed out that Alnus generally followed rather than preceded Picea . She also pointed out that sequence of arrival of species varied greatly within the region, often as a function of distance from refugia rather than in a regular successional sequence. She showed that 12 dominant forest tree genera advanced across the region at rates varying from a low of 100 m/year for Castanea to 400 m/year for Pinus ; in all 12, the average advance was at least several kilometers per generation. Moreover, the rivers in this region, unlike those in northern Europe, do not generally run north; thus long-range flotation could not have been generally important. Amazingly rapid advances were accomplished by species with light wind-dispersed seeds and by species with heavy animal-dispersed seeds. Howe and Smallwood (1982) noted that Picea , with seed averaging 0.002 g in weight, migrated at rates averaging 250 m/year, while Quercus , with seed 500 to 1,500 times heavier, advanced an average of 350 m/year. The former was probably mainly wind dispersed and the latter mainly bird dispersed.
Thus, in North America as in Europe, climate may have changed so abruptly that a period of free migration ensued. Until the advancing species eventually bumped into their relocated Holocene climatic limits, location of their moving borders may have been controlled by how long it took for each new generation to start producing seed and how far the seed was dispersed.
The migrations that can most reasonably be interpreted as under climatic control are later Holocene ones after borders had been stabilized for a while and then advanced again or retreated, for example, the fluctuations in arctic and alpine timberlines and in the prairie—forest ecotone that were discussed above. It is not yet clear, however, how widespread or local these fluctuations were. Many paleoecologists believe that North America had a climate that was warmer and drier than now during some part of the Holocene. This has been named the Xerothermic, Altithermal, or Hypsithermal period. This is problematic because dates suggested for the beginning and end of the period disagree by millennia, even within the part of the continent under consideration
here. For example, it was dated by Delcourt (1980) at 12,500–5,000 B.P. on the Gulf coast and at 8,500–4,500 B.P. on the Cumberland Plateau of Tennessee, by Watts (1979) at 10,000–6,000 B.P. in Appalachia, by Davis (1983) at 9,000–500 B.P. in northeastern North America, by King (1981) at 8,300–5,000 B.P. in Illinois, by Swain and Winkler (1983) at 5,500–2,500 B.P. Wisconsin, and by Wright (1976) at 8,000–4,000 B.P. in Minnesota. Wright (1976) and other workers have concluded that the warm dry period was "time transgressive" in different regions. If so, there is no reason to assume that either the causes or effects of whatever climatic fluctuations occurred in eastern North America during the Holocene were similar in different parts of the region. Placing such presumably heterogeneous phenomena under a single label can only cause confusion.
Davis (1965) noted that some late Holocene vegetation changes that had been attributed to climatic cooling were actually anthropogenic. For example, she found that in New England, changes in tree pollen that had been considered prehistoric and used as evidence for the end of the Xerothermic interval actually dated from the time of European colonization. The changes in abundance of tree species may have resulted from agricultural clearing, old field succession, and actual conifer planting. This reinterpretation was bolstered by the discovery of pollen of European weeds, Plantago major and P. lanceolata , in the same strata.
Davis (1965) also made a plea for greater attention to possible effects of prehistoric Indian activities on vegetation. Compared to Europe, very little has been done in North America to correlate palynology and archaeology. The American story is quite different from the Eruopean one because of the absence of pastoralism and the later development of agriculture. The Early Woodland Indians of the period 3,000 to 2,000 B.P. grew a little maize and a few other domesticated plants, but had a basically hunting and gathering economy. Only after about 1,000 B.P. was maize a major crop. Because Indian shifting cultivation was limited in time and localized in space within the region, it may be possible some day to evaluate its impact on forests of the region by correlating changes in archaeologic and palynologic data. Evaluating the impact of the more ubiquitous hunting and gathering people may be impossible. Their arrival in the region evidently coincided with the climatic revolution of the Late Glacial. It is generally assumed, perhaps correctly, that Indian burning had profound vegetational effects, particularly on the prairie—woodland ecotone, but there may be no way today to discriminate between the effects of Indian- and lightning-set fires in a vegetation mosaic that no longer exists.
Davis (1981b ) suggested a natural biotic rather than an anthropogenic or climatic cause for the dramatic hemlock decline at 4,800 B.P. , namely, outbreak of a pathogen or insect parasite specific to Tsuga .
Southwestern United States
(Baker 1983; Spaulding 1983; Spaulding et al. 1983; Thompson and Mead 1982; Van Devender and Spaulding 1979; Van Devender et al. 1984; Wells 1977, 1983; Wells and Hunziker 1976; Wells and Woodcock 1985)
The region considered here extends from the central Great Basin in Utah and Nevada through the Mojave and Sonora deserts of California and Arizona to the Chihuahuan Desert of New Mexico and Texas; this region is locally known as the Southwest. Rising above the present deserts are many plateaus and mountain ranges with scrub woodlands, conifer forests, and a few alpine tundras. The rugged topography, steep environmental gradients, and complex flora make fossil pollen records hard to decipher. Pines, junipers, oaks, and other dominant genera each have multiple ecologically diverse species with indistinguishable pollen. The pollen rain is an inseparable mixture from different altitudinal zones. In some vegetation types, imported pollen may actually be deposited more abundantly than local pollen. Moreover, pollen is preserved in relatively few sites.
Fortunately, the poor pollen record is supplemented by abundant macro-fossils from caves and rock shelters, mainly deposited by so-called pack rats or wood rats of the genus Neotoma . These animals gather all sorts of plant materials to build middens around their nests. Supposedly, they forage non-selectively and only within a radius of about 100 m from their nests. Hundreds of Neotoma middens in the Southwest contain fossil plant materials that have been 14 C dated to the Wisconsinan Glacial or the Holocene Epoch. The fossils are commonly identifiable to species. The middens present some problems in sorting out deposits made at different times. As in other kinds of fossil records, there are occasional disagreements between experts as to dating.
Full Glacial
During the Full Glacial, from about 21,000 to 12,500 B.P. , the Southwest had only scattered mountain glaciers. Presumably, alpine tundras and Artemisia steppe extended well below present timberline.
In the Great Basin, pluvial lakes, some of enormous size, set a regional base level at about 1,500 m elevation. Conifer forests grew down to this level over huge areas now dominated by pinyon—juniper scrub woodland and desert shrubs. Bristlecone pine, Pinus longaeva , now a rare subalpine species, grew as much as 600 m below its present lower limits; its range was continuous over the higher, eastern part of the Great Basin, becoming discontinuous
westward and southward. Limber pine, P. flexilis , and boreal juniper, Juniperus communis , both extended down more than 1,000 m below their present lower limits and may have had continuous ranges from the Rockies to the Sierras. Also present were a few deciduous shrubs, for example, Acer glabrum and Rosa woodsii , that are still associated with mountain conifer forests. In southeastern Nevada at the southern margin of the Great Basin and slightly below its general base level, Douglas fir, Pseudotsuga menziesii , joined Pinus flexilis as a dominant. Also present there were Rocky Mountain juniper, J. scopulorum , and a cactus, Opuntia polyacantha . Nowhere in the Great Basin, however, was there Full Glacial evidence of the species that dominate the modern pinyon—juniper woodlands, Pinus monophylla, P. edulis, and J. osteosperma, nor of the modern desert shrub communities.
The Mojave Desert and the adjacent Grand Canyon region had lower base levels and fewer pluvial lakes. The forest conifers that blanketed the Great Basin were confined to highland areas. The subalpine species, including Pinus longaeva and P. flexilis , grew above 1,800 m elevation. The montane species, including Pseudotsuga menziesii, Abies concolor, and Picea spp., grew down to about 1,500 m. At lower levels, the conifers did not form continuous forests, but were interspersed with pinyon—juniper woodland and high desert shrub species, including Pinus monophylla ; Juniperus osteosperma ; shadscale, Atriplex confertifolia ; desert almond, Prunus fasciculata ; bitter brush, Purshia tridentata ; Apache plume, Fallugia paradoxa ; curl-leaf mahogany, Cercocarpus ledifolius ; and big sagebrush, Artemisia tridentata . The lower limits of these species extended well below their present limits, but some had upper elevational limits as high as today. Shadscale actually extended to higher elevations than today.
Sites in lower parts of the Mojave, between 1,500 and 500 m, were almost all dominated by junipers, usually J. osteosperma , and accompanied in places by Pinus monophylla and various shrubs still found in pinyon—juniper woodlands today but at higher elevations, including joint fir, Ephedra viridis ; blackbrush, Coleogyne ramosissima ; antelope brush, Purshia glandulosa ; little leaf mahogany; Cercocarpus intricatus ; ash, Fraxinus anomala ; and desert sage, Salvia dorrii . Mixed with these were a few species that still grow at lower levels of the Mojave, such as wingscale, Atriplex canescens, and thornbush, Lycium andersonii . Some edaphically dry sites were nearly treeless; for example, on a rocky, calcareous, south-facing slope at Point of Rocks, Nevada, a 15,000-year-old midden had fossils of only one tree species, the Joshua tree, Yucca brevifolia . The dominants were shrubs, shadscale, and snowberry, Symphoricarpos longiflorus . The most likely region in the Mojave to have had a treeless desert scrub during the Full Glacial is probably Death Valley, with a floor below sea level and in an intense rain shadow. A Full Glacial midden dated at 20,000 B.P. from 400 m elevation on the flank of the valley in a zone now having only extremely sparse scrub of creosote bush, Larrea divaricata , and bursage, Franseria dumosa , showed Juniperus osteosperma and Yucca
brevifolia present but not abundant. Thus, junipers and Joshua trees grew more than 1,000 m below their present lower limits. The dominant species at the site was evidently the shrubby Yucca whipplei , accompanied by shadscale and Opuntia basilaris . It seems likely that the lower parts of Death Valley were completely treeless desert. Nowhere in the Mojave, however, is there Full Glacial fossil evidence of the creosote bush—bursage or other modern desert scrub communities. If creosote bush, now the most common dominant over huge areas of the Mojave, was present there during the Full Glacial, it must have been extremely rare.
The known Sonoran Desert fossil record for the Full Glacial is very sparse. Neotoma middens have been found at a few localities in the lower Colorado River basin of California and Arizona at elevations between 250 and 1,000 m. These generally show dominance by Juniperus osteosperma and Pinus monophylla , far below their present lower limits. Common associates were all species that are still common members of pinyon—juniper woodlands now restricted to higher elevations and moister climates: Ephedra viridus, E. nevadensis, Yucca brevifolia, Y. whipplei, Nolina bigelovii, Atriplex confertifolia, Acacia greggii, Cercocarpus intricatus, Arctostaphylos pungens, Rhus tribolata, Artemisia tridentata , and Haplopappus cuneatus and scrub oaks, including Quercus chrysolepis, Q. dunnii, Q. turbinella . As in the Mojave, the northern Sonoran Full Glacial vegetation evidently lacked the common dominants of the modern desert scrub. Whether they were present anywhere in the huge Sonoran Desert south of the U.S.—Mexican border is not known.
In New Mexico and Texas, the region now occupied by Chihuahuan Desert scrub at elevations below 1,500 m had a Full Glacial vegetation of pygmy conifer woodland. Midden deposits consistently show dominance by junipers, usually J. scorpulorum , and by pinyons, Pinus edulis and P. remota . The first two species grew at lower elevations and farther south than now; P. remota grew much farther west than now. Associated with the pinyons and junipers at lower elevations were scrub oak, other shrubs, and succulents, such as Agave, Yucca , and Opuntia spp. At higher elevations in the mountains of New Mexico and Texas, pinyons and junipers were associated with montane and even subalpine conifers. For example, middens dated 13,000 to 15,000 B.P. at elevations between 1,600 and 1,700 m in the Sacramento and San Andres mountains of southwestern New Mexico showed the presence of Pseudotsuga menziesii in a pinyon—juniper woodland. A San Andres midden also yielded a unique Late Glacial record of Pinus ponderosa . In the nearby Guadalupe Mountains of western Texas, middens dated at 13,000 B.P. at elevations of about 2,000 m showed that Pinus edulis and Juniperus scopulorum grew together with not only Pseudotsuga but also with subalpine spruces, Picea engelmannii and P. pungens , and a white pine, Pinus strobiformis .
Thus, during the Full Glacial the Southwest from timberline to base level was evidently dominated by conifers that are now restricted to disjunct highlands. These formed forests at high elevations and woodlands below.
There is no record of extensive ponderosa pine parkland, sagebrush steppe, or treeless desert scrub. Some species now present in those communities are known from Full Glacial fossils as subordinate members of conifer woodlands and also in local microhabitats. Other species that are today among the most widespread and abundant in deserts of the Southwest are completely missing from the Full Glacial record, including columnar cacti, Cereus spp.; ocotillos, Fouquieria spp.; bursage, Franseria dumosa ; and paloverdes, Cercidium and Parkinsonia spp.
Late Glacial
Fragmentary evidence suggests that plant migrations signaling the end of the Full Glacial in the Southwest began between 12,500 and 10,000 B.P. , a time span arbitrarily called the Late Glacial.
In the eastern Great Basin, sequences of midden deposits at different elevations in the Confusion Range show upward displacement of the lower border of the subalpine Pinus longaeva . At 12,000 B.P. it still extended down to the lowest sites at about 1,700 m elevation; by 10,300 B.P. it was gone even from the highest sites at 2,160 m. Its upward retreat was closely followed by advance of Pseudotsuga menziesii and Juniperus scopulorum . During the Full Glacial, their nearest approach to the Confusion Range had been at Rainbow Canyon, 160 km to the south. By 12,000 B.P. Pseudotsuga had arrived at the lowest sites in the Confusion Range, but Juniperus scopulorum was not yet present there. By 10,300 B.P. , these two species dominated the range up to the summit.
In the present Mojave Desert, major plant migrations evidently began about the same time. At Rampart Cave, at 500 m elevation in the Grand Canyon, where there had been pinyon—juniper woodland around 12,000 B.P. , there were only desert shrubs by 10,000 B.P. At Point of Rocks, at 900 m elevation in Nevada, the site mentioned above as having had Joshua trees in the Late Glacial had become much more similar to the present by 11,700 B.P. By then it had only desert shrubs, including desert almond, Prunus fasiculata ; snakeweed, Gutierrezia microcephala ; and Opuntia spp. being dominant. In Death Valley at 400 m, where a Full Glacial midden had shown juniper and Joshua trees present, a 10,000-year-old midden showed only bursage, Franseria dumosa . Higher up on the flanks of Death Valley at 800 m, where Utah juniper had been dominant in the Full Glacial, only shrubs were present by 11,000 B.P. These included Yucca whipplei ; antelope brush, Purshia glandulosa ; rubberbrush, Chrysothamnus teretifolius ; and Opuntia basilaris . The Mojave was evidently first invaded in Late Glacial by a scrub oak, Quercus turbinella , which had been present in the Sonoran Desert during Full Glacial. The first Mojave macrofossils of this species, dated 12,000 B.P. , were found 60 km west of Searles Lake, California. In the same region, a
rise of oak pollen before 11,000 B.P. may mark the advance of this now common Mojave oak.
In the northern Sonoran Desert, middens indicate that Pinus monophylla began its northward and upward shift between 13,000 and 10,000 B.P. , leaving behind an impoverished juniper woodland. The first midden record of a desert community without woodland species is from the lower Colorado River valley near Yuma, Arizona. Dated at 10,500 B.P. , the middens contained creosote bush, Larrea divarieata , and bursage, Franseria dumosa , which still grow in the area.
In the present northern Chihuahuan Desert of New Mexico and Texas, middens dated between 10,500 and 10,000 B.P. at elevations of 1600 to 1700 m in the Sacramento Mountains showed change from the Full Glacial vegetation. Pinyon and juniper persisted but Pseudotsuga menziesii was gone, replaced by desert shrubs, including zorillo, Choisya dumosa , and honey mesquite, Prosopis glandulosa . In the nearby Guadalupe Mountains at 2,000 m elevation, where there had been a complex Full Glacial mixture of pinyon, juniper, and forest conifers, all the conifers except juniper were gone by 11,500 B.P. , replaced by grassland. Pollen profiles from the Llano Estacado, adjacent to the Chihuahuan desert on the northeast, show a change between 14,000 and 10,000 B.P. from pine and spruce dominance to an association transitional to the present grassland and desert scrub.
Holocene
After the beginning of the Holocene, at about 10,000 B.P. , lower elevations in the Great Basin were abandoned by the subalpine conifers, such as Pinus longaeva, P. flexilis , and Juniperus communis , and were temporarily occupied by spreading montane conifer species, especially Pseudotsuga menziesii, Abies concolor , and Pinus ponderosa . The available midden record is so spotty in time and space that the migrations can be bracketed only loosely and at a few places. For example, in the Snake Range in eastern Nevada at elevations between 2,000 and 2,700 m, these three montane conifers were absent at 9,200 B.P. but present by 4,600 B.P. Pseudotsuga and Abies are known to have been present during glacial time 200 and 280 km south of the Snake Range, respectively. Where ponderosa pine came from is a great mystery. Its only known glacial record in the Southwest is from New Mexico. The first available Holocene record is also from New Mexico, 700 km east of the Snake Range, at Chaco Canyon, from about 5,500 B.P.
Wells (1983) noted that there is no evidence these montane conifers, unlike the subalpine species, ever had continuous ranges across the Great Basin (fig. 11) and that their migration rates during early Holocene time were orders of magnitude too fast to have been accomplished by short-range wind dispersal of their heavy seeds. He noted that common birds of the
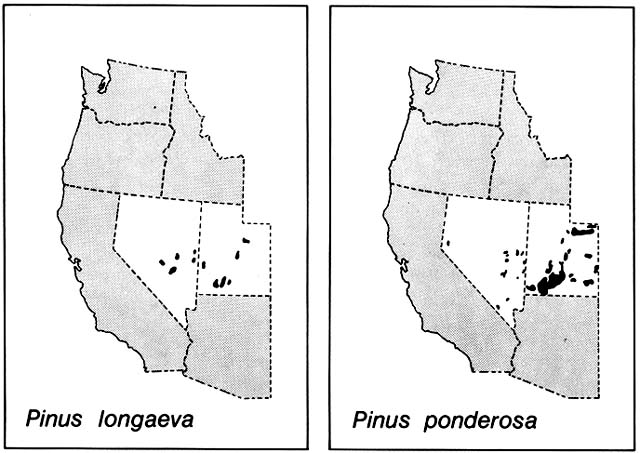
Figure 11. Partial Present Ranges of Pinus longaeva and P. ponderosa in the Great Basin. Bristlecone grows up to
timberline and Ponderosa at slightly lower elevations, but both are confined to high mountains that rise like forested
islands above a sea of desert scrub. These strikingly similar modern patterns of the two species conceal radically
different migrational histories. During the Wisconsin Glacial period, bristlecone blanketed lower elevations in the region
down to the great pluvial lakes, its present disjunctions are the result of upward retreat when the postglacial arid climate
developed. During the glacial period, ponderosa was evidently absent from the entire region and it did not immigrate until
the lowlands were too dry for it. Its disjunctions probably result from long-range bird dispersal between mountain
forests. (Adapted from Critchfield and Little, 1966)
region, especially jays, nutcrackers, and crossbills, are excellent long-range dispersal agents, not only of conifers but also of oaks and montane shrubs. Thus, the present disjunct, islandlike montane ranges of many plant species in the Great Basin probably are not the result of fragmentation of continuous early Holocene ranges but rather were achieved by long-range colonization. This colonization was not completed until after pinyon and juniper, also dispersed long distances by birds, had occupied intermediate elevations and after lowlands of the Great Basin had been taken over by desert scrub dominated by Artemisia and Atriplex .
By 10,000 B.P. in the Mojave Desert, the disjunct patches of subalpine conifers had begun their upward withdrawal, leading to extinction on most mountain ranges. They were being replaced at elevations of up to 2,400 m by Juniperus scopulorum and other montane conifers. The montane conifers, which had extended down to about 1,500 m elevation, were in turn being replaced by J. osteosperma, Pinus monophylla , and other members of the modern pinyon-juniper woodlands, which had previously occupied the lower levels of the region. By about 9,500 B.P. , these woodlands were being invaded by a scrub oak, Quercus dunnii , which was present in the northern Sonoran Desert during glacial time. By 7,500 B.P. the lower levels of the Mojave Desert were being taken over by desert scrub, including Larrea divaricata , similar to modern associations.
Early Holocene records in the Sonoran Desert are sparse. At some places, juniper woodland was withdrawing from levels below 1,000 m and being replaced by Larrea -dominated desert scrub by 7,500 B.P.
In the present Chihuahuan Desert of Texas, sparse evidence suggests gradual decrease in woodland species and increase in desert grassland species until about 4,000 B.P. , after which Larrea divaricata, Fouquieria splendens, Mammillaria spp., and other desert scrub species arrived.
Problems of Interpretation
Macrofossils preserved in pack rat middens have provided much more accurate information on the Southwest's prehistoric plant geography than was available from the region's sparse and almost indecipherable pollen record. The midden record, however, has its own interpretational problems. Each individual fossil assemblage gives highly accurate and complete information on plant species present, but only for a very tiny area and a short time span, leaving huge gaps to be filled by interpolation. This is problematic because location and preservation of middens were not necessarily random in relation to vegetation patterns. Some bias must be expected simply because preservation of ancient middens depended on shelter by rocks from rain and fire. The possibility that unrecorded plant species were present in the region
is taken more seriously by some experts than others. For example, Spaulding et al. (1983) suggest that many species that are still missing in the midden record were probably present since pre-Wisconsinan times on both sides of the Continental Divide. By contrast, Wells (1983) tends to take the absence from the record (at least of species that are presently common) at face value; he prefers to postulate that they immigrated from the south after Wisconsinan times.
Another limitation of the midden record is that the rats deposited material irregularly and episodically. Unlike pollen deposits, which under ideal conditions may accumulate continuously in neat strata through long time spans, midden deposits are commonly interrupted and disturbed, especially by rodent tunneling. This problem is well recognized by all investigators, but opinions differ as to the best methods of dissecting a midden and dating its components.
Thus, there is room for conflicting interpretations of the midden macrofossil record, as shown by the case of Larrea , the creosote bush, which dominates huge areas of all three North American deserts. It is agreed that the North American Larrea population, sometimes called L. tridentata , was ultimately derived from a species native to temperate South America and that its intercontinental dispersal was probably due to migratory birds. The North American population has differentiated into separate races, with the Chihuahuan Desert race being diploid, like the South American progenitor. The other two races are polyploid, 4N in the Sonoran Desert and 6N in the Mojave. The simplest hypothesis would be that the 4N and 6N races evolved in sequence as the species expanded westward from a founding colony. However, Larrea does not appear in the available macrofossil record of the northern Chihuahuan Desert until about 3,300 B.P. , whereas it was definitely present in the Sonoran Desert of southwestern Arizona by about 10,500 B.P. and in the Mojave Desert of California by about 7,500 B.P. There are reports of even earlier Larrea fossils from Sonoran and Mojave middens, but the dates are questionable. Unfortunately, the Neotoma fossil record has not been investigated south of the Mexican border.
Using a different approach, Vasek (1980) estimated the age of the largest known Larrea clone in the California Mojave Desert as nearly 12,000 years. This clone has a radius of nearly 8 m around a central bare area from which the old dead wood has rotted away. The age was extrapolated from modern growth rates. Vasek suggested that growth rates during the early Holocene may have been greater and that the clone's age might be reasonably estimated as about 9,500 years.
On the basis of current evidence, Wells and Hunziker (1976) proposed that dispersal of Larrea into North America occurred in the Late Glacial, with rapid northward and westward migration accompanied by differentiation of the polyploid races. Based on the same evidence, others (Spaulding
et al. 1983; Van Devender et al. 1984) proposed that Larrea arrived and expanded westward across the Continental Divide during an earlier Pleistocene interglacial period.
Whatever uncertainties remain, the Neotoma macrofossil record has provided solid evidence of major, rapid plant migrations in the Southwest since Late Glacial time. Some of these migrations could not have been even roughly reconstructed by postulating displacement en masse of modern vegetation.
In the Southwest, as in other regions discussed previously, Late Glacial and early Holocene plant migrations were generally so rapid that it seems gratuitous to postulate gradually changing climatic controls. The problem is explaining how species borders could shift so fast, not what was restraining their movement. The evident lack of climatic brakes on early Holocene plant migrations does not preclude possible later Holocene climatically controlled vegetation changes. The classic Altithermal model postulated that during middle Holocene, climate in the Great Basin was warmer and drier than now; there is some supporting evidence of this (Byrne et al. 1979). In the Southwest, however, as in other regions discussed previously, whatever late Holocene climatic fluctuations occurred were evidently not synchronous or equivalent in different parts of the region (Baker 1983), and their effects on plant distributions are unclear.
Some late Holocene vegetational changes have been attributed to human impact. For example, on the Colorado Plateau in northwestern New Mexico, from about 5,500 to 1,250 B.P. , Neotoma middens show an apparently stable altitudinal gradient from desert scrub at lower elevations up through pinyon and juniper to ponderosa pine. After 1,250 B.P. , middens no longer contained pinyon or ponderosa pine and contained little juniper, although all three are present in the region now. Archaeologists have postulated that the Anasazi people, who occupied Chaco Canyon and other parts of the region from before 1,000 to 800 B.P. , used approximately 100,000 ponderosa pines to construct their multistoried pueblos and other buildings and also used huge quantities of pinyon and juniper for fuel.
Northern South America
(Bradbury et al. 1981; Flenley 1979; Hammen 1974; Leyden 1985; Salgado-Labouriau 1982)
In the Colombian Andes, pollen profiles dated between 30,000 and 14,000 B.P. suggest that during the Full Glacial, borders of vegetation zones were about 1,000 m below their present levels. Thus, species belonging to the alpine paramo, the subalpine heathlike scrub, and the montane forest must
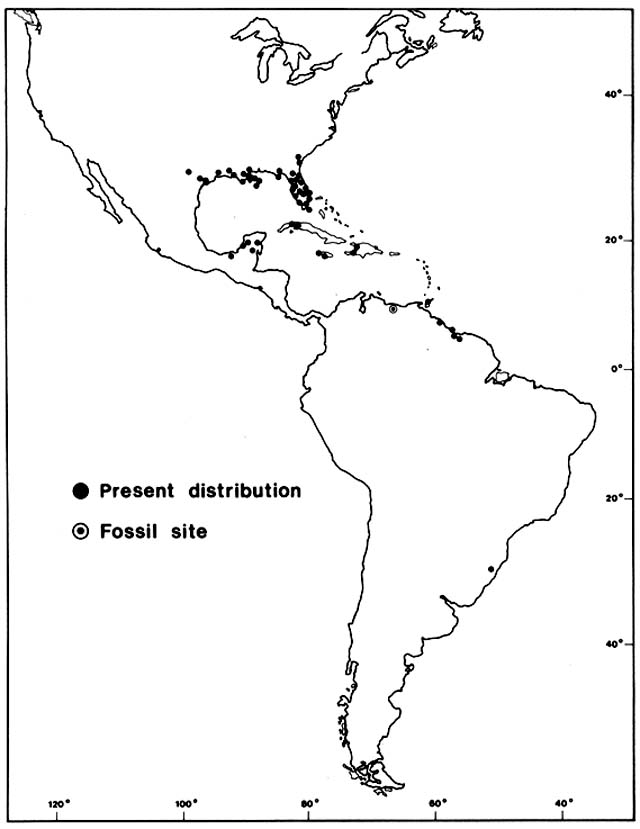
Figure 12. Present Distribution of Amaranthus australis . Without historical evidence, it would be debatable
whether such a disjunct distribution was ancient or recent and whether it was due to natural or human dispersal.
In this case the pattern is probably old and natural. Fossil evidence from Lago de Valencia in Venezuela suggests
that the population of this species now growing around that lake was established about 8,300 years ago. In
southeastern United States seeds of this species are eaten in large quantities by migratory waterfowl about to take
off for the tropics, and most of the West Indian and Latin American colonies grow along major flyways. All the
close relatives of this species are North American natives. It is doubtful, however, that A. australis could have
survived there during glacial periods. Perhaps birds reintroduced it to its homeland from tropical colonies.
(Adapted from Sauer, 1972)
have had ranges that were slightly broader than now but not continuous between major mountain ranges. In other words, ranges of highland species, including Podocarpus, Alnus, and Quercus, may have always been disjunct in the region. In the adjacent lowlands, savanna grassland and open woodland, with Byrsonima crassifolia and Curatella americana as dominants, were probably more continuous than at present. There is no evidence that lowland tropical forests, now extensive in the region, were present during the Full Glacial. Between 14,000 and 10,000 B.P. , pollen profiles suggest a rapid upward displacement of vegetation borders to approximately modern elevations, although individual cores within the region are not closely synchronous.
In Venezuela, a 40-m-deep lake, Lago de Valencia, lies at an elevation of about 400 m. Present natural vegetation of the surrounding region is a mosaic of savanna, tropical deciduous forest, and wet evergreen forest, depending on topography. Late Glacial profiles dated between 13,000 and 10,500 B.P. show that instead of a lake the basin at that time contained saline marshes dominated by Cyperaceae and Poaceae with some Asteraceae , including Ambrosia , and Amaranthaceae , especially Alternanthera . The pollen also suggests that during the Late Glacial upland vegetation surrounding the marshes was predominantly savanna. Rare tree pollen of Bursera and Acalypha indicates that there was some open deciduous woodland in the watershed but no forest. This does not support a hypothesis advanced by several biogeographers who attempted to deduce prehistoric migrations of taxa from present distributions. They proposed that the Lago de Valencia region was a Pleistocene refugium for various tropical forest plants and animals. Pollen profiles dated 10,500 to 9,800 B.P. from Lago de Valencia show that the Pleistocene saline marshes and savannas were replaced in the early Holocene by a shallow, brackish lake with widely fluctuating margins. This lake supported both aquatic and littoral halophytes, including Ruppia , chenopods or amaranths, and Ambrosia . Low but consistent input of tree pollen, mainly Bursera, Acalypha, and Moraceae, indicates an open woodland in the watershed but not near the lake.
Between 9,800 and 8,300 B.P. , the lake filled, overflowed, and freshened, with a change in aquatics and a sharp decline of littoral halophytes. At the same time, there was a sharp rise in tree pollen, especially Bursera, Spondias, Trema, Brosimum, and other Moraceae, indicating advance toward the lake of early successional tree species, presumably on thin, undeveloped soils. At about 8,300 B.P. , there was a sudden rise in a pollen type that may document arrival of Amaranthus australis, a species now abundant in marshes along the lake shore (fig. 12). This tall herb is native to the Everglades in Florida and to other marshes of the subtropical and tropical Gulf of Mexico region. It also has a widely disjunct distribution in marshes of the West Indies and South America, presumably due to long-range dispersal by migratory waterfowl,
as discussed above in Chapter 1. Pollen profiles from Lago de Valencia indicate that since 8,300 B.P. , the region around the lake has had a mosaic of savanna, woodland, and forest with moderate fluctuations in abundance of different taxa, but basically much like the modern vegetation.
Pollen cores on the coasts of Guyana and Surinam document drowning of some savannas during early Holocene time by eustatic rise of sea level. Grasses were replaced by mangroves, first Avicennia , followed by a long period of dominance by Rhizorphora , indicating deeper water. Later, Avicennia reappeared, perhaps due to sediment deposition after sea level rise tapered off.
Reconstruction of Late Glacial and Holocene plant migrations in northern South American is still in an embryonic state. Available fossil pollen records, especially those with adequate dating, are few and far between in a region with formidable vegetational diversity. Little is known about the pollen signatures of present vegetation types of the region. Translating fossil pollen spectra into vegetation types will be especially hard for tropical forests with predominantly insect pollination. Also, detecting prehistoric human influences on plant distributions may be extremely difficult. Most late Holocene pollen diagrams from northern South America show some indications of forest clearance, and some have maize pollen in their upper levels, usually since about 3,000 B.P. However, archaeological evidence indicates that agriculture had begun in the region long before that, presumably on such a local scale that the effect on the pollen rain was very subtle. Most tropical crops are poorly recorded in fossil pollen records, and the pollen of many milpa weeds and second-growth trees is indistinguishable from that of natural riparian pioneer vegetation.
Japan
(Tsukada 1982a,b,c, 1983a,b,c, 1985)
During the last Full Glacial, from about 25,000 to 15,000 B.P. , lowering of sea level made the Sea of Japan a lake, and the present Japanese islands formed an interconnected arc with land bridges to mainland China via Korea and to Siberia via Sakhalin. Arctic tundra extended southward to northern Hokkaido, and there were mountain glaciers and patches of alpine tundra down to central Honshu. The tundra graded through park woodland with pines and tree birches into boreal conifer forest, which covered most of northern Honshu and the highland areas to the southwest. Temperate conifers covered most of southwestern Japan and graded into a mixed forest of temperate conifers and deciduous hardwoods in southern coastal areas. In these forests, the species associations and dominance were completely different
than in the modern mixed conifer—hardwood forests. Perhaps members of the modern broadleaf evergreen laurel—oak forest survived in the extreme south, but fossil evidence for this has not yet been found.
After about 15,000 B.P. , in Late Glacial and early Holocene time, the vegetation changed rapidly. The borders of physiognomic formations moved roughly 10° northward in latitude at a given elevation and 1,400 m upward in elevation at a given latitude. By 10,000 B.P. , rising sea level had separated the islands and brought moist, marine climate to the west and east coasts.
Interpretation of the pollen record is difficult because most important tree genera are represented in Japan by multiple species. Tsukada (1982b , 1983a , 1985) has been able to discriminate between some congeners and, with the help of macrofossil evidence, has proposed a tentative reconstruction of tree migrations, only a few of which will be sketched here as examples.
The deciduous beech is represented in Japan by two species, Fagus crenata and F. japonica . The two are difficult to distinguish palynologically and have widely overlapping modern ranges; they will be treated here as a unit. Fagus evidently survived the Full Glacial in sparsely scattered populations along the coasts of Honshu south of 37°N latitude. About 12,000 B.P. , Fagus began invading inland mixed conifer—hardwood forests and expanded rapidly northward. By 9,000 B.P. , Fagus was moving into far northern Honshu, by 8,000 B.P. into southern Hokkaido, and by 7,000 B.P. was approaching its present northern limit. Meanwhile, in the south, Fagus had retreated from the lowlands of Kyushu and Shikoku during Late Glacial time and from the lowlands of southern Honshu in early Holocene time, when the deciduous hardwoods were replaced by evergreen broadleaf hardwoods.
Cryptomeria japonica , closely related to Sequoia and Taxodium , had Late Glacial refugia on both coasts of Honshu centering around 35°N latitude and on the south coast of Shikoku in lowland areas now partly covered by the sea. About 12,000 B.P. , Cryptomeria began migrating inland and upward. It also began expanding in both directions along the Sea of Japan coast of Honshu. Moving southwestward, it reached the southwest tip of Honshu by 7,000 B.P. ; moving northward, it reached its present natural limit on the west coast by about 4,000 B.P. Its northward expansion on the Pacific side of Honshu was slower, not being completed until about 1,000 B.P.
Tsukada (1983c ) has suggested that human activities played an intriguing and important role in the ancient vegetational history of Japan. When large game animals, including mammoth, bison, horse, giant elk, and boar, became extinct there in the Late Glacial, the Paleolithic people turned increasingly to plant foods. At that time, the advancing deciduous broadleaf forests supplied an abundance of beechnuts, acorns, chestnuts, walnuts, and hazelnuts. Human transport of these seeds may have accelerated forest migration. In the early Holocene, archaeological sites continued to be concentrated in deciduous forest regions with abundant nut supplies. There is evidence of a
primitive buckwheat agriculture as early as 6,600 B.P. in southwestern Honshu. In the Late Jomon period, about 4,000 to 3,000 B.P. , slash and burn agriculture spread in southwestern Japan, first for buckwheat and soon after for rice cultivation. As forest clearance intensified and spread into northeastern Japan, it was followed by spread of weed plants. Prominent among these was Japanese red pine, Pinus densiflora , which spread explosively in old fields starting about 2,000 B.P. in southwestern Japan and approached its present limit in northern Honshu about 700 B.P.
Cryptomeria was much exploited by prehistoric humans, the decay-resistant wood being easily split by simple, primitive tools. Since before 2,000 B.P. , Cryptomeria boards and posts were much used to dike flooded rice fields. Drastic reduction in Cryptomeria pollen followed its middle Holocene maximum. After about 1,000 B.P. , Cryptomeria again increased markedly, presumably due to protection and reforestation. Deliberate prehistoric planting is believed to be responsible for the arrival of Cryptomeria on Kyushu about 2,000 B.P. and on Tsushima Island, between Kyushu and Korea, about 1,500 B.P. Planting of Cryptomeria on Hokkaido began much later.
East Africa
(Butzer 1971; Flenley 1979; HSamilton 1974, 1982; Livingstone 1967, 1971, 1975)
Sharp climatic gradients on the towering East African volcanoes cause a whole spectrum of vegetation types, from alpine tundra through heath and montane forest to lowland forest, to be telescoped into a short horizontal distance. The region has no deposits of local macrofossils like the Neotoma middens of southwestern North America and therefore must rely on palynology for fossil evidence. As in other mountain regions, palynology faces problems in sorting out local from transported pollen, but careful work has revealed some major vegetation changes since glacial times.
During the Full Glacial, snowlines were about 1,000 to 1,200 m lower than today, producing glaciers on Mount Kenya, Ruwenzori, Kilimanjaro, and Elgon.
On Ruwenzori, valley glaciers flowed down below the snowline to about 3,000 m elevation until about 1,500 B.P. The moraines that they abandoned formed Lake Mahoma, which is now surrounded by montane forest. Fossil pollen and grass phytoliths from the lake indicate that until about 13,000 B.P. it was above timberline and close to the border between alpine tundra and heath; present nearby were some of the famous Afro-alpine endemic plants, including the giant Lobelia and Dendrosenecio . Soon after 13,000 B.P. ,
rising timberline evidently passed the lake with arrival of various montane forest species. However, the presently important montane forest conifer, Podocarpus , did not arrive until about 3,000 B.P. About 1,000 B.P. , there was a strong increase in grass and weedy dicot pollen that may have signaled agricultural clearing in distant lower elevation areas.
On Mount Kenya, borders between vegetation zones were about 1,000 m below their present levels from about 26,000 to 14,000 B.P. A pollen profile from a site at 3,100 m elevation, now surrounded by heath shrub, shows that it was surrounded by alpine tundra grassland until about 10,500 B.P. when the rising grassland—heath border passed that level. At the same time, heath vegetation was replaced by forest at a lower site, at 2,400 m elevation. The first tree species to arrive, Hagenia abyssinica , appeared about 10,500 B.P. and was soon followed by some other montane forest trees, but Podocarpus did not arrive until about 5,000 B.P. Hagenia remains dominant today at the heath—forest border, about 500 m above the site.
In the Cherangani Hills, near Mount Elgon, fossil pollen from a 2,900 m elevation site indicates an alpine grassland with Dendrosenecio from 28,000 to 14,000 B.P. , when heath vegetation took over. After about 10,000 B.P. , Olea and other dry montane forest trees arrived; Podocarpus arrived about 9,000 B.P. The present vegetation near the site is mainly savanna, which may have been formed by clearing of the dry forest.
The pollen profiles mentioned above are all from highlands and had alpine tundra or heath vegetation during the Full Glacial. There are no Full Glacial sites that recorded presence of forest, so locations of Pleistocene refugia of montane trees are unknown. They evidently did not descend low enough to spread out widely or have continuous migration routes between the mountain ranges. The few pollen records from lower elevations indicate that the great East African plains had Full Glacial vegetation much like the present. A pollen record from west of Lake Malawi at 1,400 m elevation indicates the presence since 20,000 B.P. of open grassy woodland similar to the present. A large number of species persisted from Late Glacial through Holocene time with virtually no change in the roster until signs of Iron Age agriculture appeared. Another pollen profile from Lake Victoria at 1,100 m elevation indicates a regional vegetation of grassy savanna until about 13,500 B.P. , when Olea, Trema , and other dry forest trees arrived. Much later, a decrease in forest and an increase in grass may date from agricultural clearing.
Thus, there was a fairly coherent pattern of abrupt upward displacement of borders between alpine and subalpine vegetation, starting about 14,500 B.P. , followed by more prolonged migrations of Podocarpus and other montane trees. Livingstone (1975) noted that Holocene migration rates and sequences of arrival in the East African highlands were clearly not limited by changing climate, but may have been controlled by distance from refugia and gradual soil development, especially on moraines.
7
Pleistocene Before
Last Glacial
The fossil record becomes more and more fragmented with increasing time depth. Also, time before the Last Glacial is beyond the reach of 14 C dating. Eventually it may be possible to establish a solid global chronology based on other kinds of dating, including seafloor magnetic reversals, such as the Jaramillo and Olduvai events. The terrestrial fossil record, however, has not yet been tied to marine chronology, and stratigraphic correlations between local series remain questionable. Furthermore, identification of fragmentary fossils with living taxa, particularly at the species level, becomes increasingly tentative with time depth. Nevertheless, the fossil record does give some insight into Pleistocene plant migrations; although the view is dim, it is real and not imagined.
Northwestern Europe
(Butzer 1971, 1974; Frenzel 1968; Pennington 1969; Szafer 1966; Turner 1975; West 1970)
The vegetation history of successive cold and warm phases (Table 2) was in some respects cyclic and repetitious. In Britain, for example, the forest invasions at the beginning of each warm phase or interglacial for which there is a good record followed a sequence roughly similar to that of the Holocene: pioneer herbs, shrubs, Betula , and Pinus preceded the mixed hardwood forest of Quercus, Ulmus, Fraxinus, Corylus , and the later arriving Carpinus and
sometimes other tree genera. At the close of each warm phase, the mixed hardwoods were replaced by pines, birches, and readvancing heaths and bogs before the spread of steppe and tundra in the next cold phase.
Within this gross cycle, species migrations showed many striking differences between successive phases, partly due to regional extinctions during cold phases. For example, Sequoia and Tsuga returned to the region for the last time during the Cromerian, Carya and Pterocarya for the last time in the Holstein Interglacial. The last record of Rhododendron ponticum in Ireland, before its modern reintroduction (discussed in Chapter 3), was also in the Holstein. By then, plant extinctions were nearly over and the flora was nearly identical to the Holocene flora. Animal extinctions continued longer. Forest elephants, hippopotami, rhinoceroses, water buffalo, and monkeys were still present in northwestern Europe in the Eem Interglacial.
The sequence of immigration of members of the mixed hardwood forest was not constant in different warm phases. For example, of three common associates in Britain, Corylus arrived first in the Pastonian Interglacial, Quercus in the Hoxnian, and Ulmus in the Ipswichian. On the continent, the mixed hardwood forest species rapidly replaced conifers during the Cromerian and Eem interglacials, but during the intervening Holstein Interglacial, the hardwoods remained a minor and patchy component of a persistent, monotonous conifer forest dominated by Picea and Abies . Also, the ultimate borders of some species varied greatly between successive warm periods. For example, macrofossils show that during the Holstein, the Eem, or both, Fagus sylvatica, Tilia platyphylla, Vitis sylvestris , and Buxus sempervirens had borders far north of their Holocene ones in both the British Isles and on the continent. Macrofossils of Hedera helix and Ilex aquifolium , however, showed that their Holstein and Eem borders remained south of their Holocene ones.
Szafer (1966), Frenzel (1968), and other workers have long recognized that plant associations throughout the Pleistocene were kaleidoscopic, not constant blocks of species. This was true not only during migrations following shifts in climate, but also of so-called climax vegetation that had equilibriated during the middle of warm and cold phases. This was probably partly due to climatic differences between successive cycles, but it was probably largely due to vagaries of migration. Reinvasions of forest species during successive warm phases had to come from distant southern refugia. Butzer (1985) noted that full glacial climates in the Mediterranean region were so harsh that refuges for temperate forest species were not islands of familiar vegetation but strange associations, probably stunted and sparse in growth, that were sporadically distributed in areas of broken topography on sunny slopes with shelter from the wind and favorable moisture conditions. The sparse fossil record in the Mediterranean obscures the locations of refugia, but it is likely that they varied greatly between successive cold phases and between species. Each readvance of a species during a warm phase may have followed
|
lowed a unique pathway. Rates of advance must have varied between species depending on time to maturity, radius of seed dispersal, and competitive and successional interactions with other species.
A less obvious point made by Frenzel (1968), Turner (1975), and other workers is that retreat of tree species late in the interglacial phases may not have been due solely to climatic deterioration. It is exceedingly difficult to disentangle soil acidification, podsolization, and expansion of healthland due to climatic change from soil changes mediated by vegetation. West (1970) suggested that hardwoods persisted longer in Britain during the Ipswichian than in other interglacials because the failure of Picea and Abies to immigrate in the Ipswichian may have reduced podsolization then.
Advances and retreats of cold-tolerant species were probably also individual rather than latitudinal shifts of blocks of species. Pollen evidence from glacial phase loess deposits is sparse, but what is present does not suggest a flora like the Holocene arctic-alpine tundras of Europe. Glacial floras of the region were perhaps more like those of the present high, cold, arid steppes of interior basins of Eurasia. Bell (1969) noted that glacial fossils, both in Britain and on the continent, included halophytes, such as Glaux maritima and Suaeda maritima, along with steppe species and did not include the whole steppe flora. Retreats of cold-tolerant species during interglacials were probably much less catastrophic than those of warmth-requiring species during glacials. Steppe and tundra plants were driven back not by warmth but by competing vegetation. They may have mostly retained a wide presence in northern Europe during interglacials on steep rocky slopes, along seacoasts, lakeshores, and streams, without remaining in the fossil record.
Extinct Homo erectus and forms transitional to H. sapiens were present in Europe since at least Elster time. During the Elster and Saale glacials, these Paleolithic people evidently subsisted largely by hunting big herbivores of open grasslands. During the interglacials, they evidently adapted to open woodland habitats. It is generally assumed that Paleolithic peoples lacked the population size and technology to have much effect on the flora and fauna. They may, however, have aided in the amazingly rapid migrations of hazel, beech, and other nut-bearing trees. Also, there is evidence from a Paleolithic site in Suffolk, Great Britain, of forest burning during the Holstein Interglacial. An associated pollen profile shows a sudden decline in tree pollen and a rise in pioneer weedy plants. Some plants now considered anthropophilic weeds, such as Rumex acetosella, Polygonum aviculare, and Stellaria media, were recorded as fossils in northern Europe by Cromerian time, that is before archaeological evidence of the arrival of Homo . They evidently were native pioneers preadapted to join in the migrations of early humans as ruderal camp followers.
Southern California
(Axelrod 1966, 1978, 1983a )
Macrofossils of the Soboba flora are believed to date from about 1 million years ago, contemporaneous with the Nebraskan Glacial. They are from a low elevation site on the western flank of the San Jacinto Mountains, where the present vegetation is sage scrub, transitional between chaparral and semidesert scrub. Most of the Soboba fossil flora is represented by species that survive in the region but that now grow fully 1,000 m higher in montane mixed conifer and chaparral: Abies concolor, Libocedrus decurrens, Pinus lambertiana, P. ponderosa, P. coulteri, Pseudotsuga macrocarpa, Populus tremuloides, several species of Ceanothus, Rhamnus crocea, Prunus ilicifolia, Rhus ovata, Garrya flavescens, and Cercocarpus betuloides . Other species are extinct in the region, including Acer brachypterum, which survives in summer rain regions of Arizona, and Magnolia grandiflora, which survives in nature only in the southeastern United States but has been reintroduced to California in cultivation and thrives if given summer watering.
Fossil cones believed to be roughly contemporaneous with the Soboba flora were found near Ventura; they had evidently floated downstream to coastal lagoons and were later buried in deeper water offshore by a turbidity current. They include several closed cone pines, Pinus radiata, P. attenuata, and P. muricata/remorata ; digger pine, P. sabiniana ; and Douglas fir, Pseudotsuga menziesii . All of these survive within 200 km of the site as highly disjunct relicts, but most are now abundant only considerably farther north or in the interior.
This very limited glimpse of early Pleistocene cold phase flora suggests that some montane conifer and chaparral species had more continuous lowland ranges than they do now and that many of the modern lowland species were absent. Considering, however, that it is 1 million years old, the early Pleistocene flora of southern California is startlingly modern, particularly in comparison to mammals of the same age, which mostly belonged to genera that are now extinct.
Northern Andes
(Hammen 1979)
A lake basin at 2,600 m elevation on the Sabana de Bogotá in Colombia has yielded a continuous pollen record for the whole Pleistocene. The lake now lies in an open edaphic savanna within the general cloudforest belt about
800 m below the present alpine tree line. Fossil pollen shows repeated oscillation between floras of forest and treeless alpine paramos, which would have caused repeated isolation and coalescence of paramo islands.
More significant migrations are suggested by an increase in the roster of species over the whole Pleistocene. Of cloudforest trees, Podocarpus was already present at the beginning of the Pleistocene, Ilex and Weinmannia arrived early in the Pleistocene, Alnus along with the shrubby Gunnera in the middle, and Quercus and Drimys late. In the alpine flora, Polygonum and Plantago were present at the beginning, Miconia and ericads arrived early, and Gentiana and Ranunculus late in the Pleistocene. Some of the cloudforest species, including Podocarpus, Weinmannia, Gunnera, and Drimys, presumably arrived from the south. Alnus, Quercus, and most of the alpine paramo species are believed to have immigrated from the north.
Much tectonic uplift of the Andean Cordillera was probably accomplished before the Pleistocene, providing a highland pathway from the south. Although the Panama landbridge was also formed before the Pleistocene, it never offered a highland bridge. Highland immigrants from the north presumably arrived by long-range bird dispersal.
8
Neogene (Miocene
And Pliocene)
The Neogene Period spans over 20 million years, more than ten times the span of the whole Quaternary (Table 3). The fossil record reveals only glimpses separated by great gaps in space and time. However, there are some rich macrofossil deposits with floras that are almost all identifiable with living genera; many members appear to be closely related or identical to living species. In paleobotany, Tertiary and older fossils are usually given discrete species names in separate time levels, partly because their fragmentary nature prevents complete comparisons. Since the fossil binomials are meaningless to nonpaleobotanists, however, a common practice (which will be followed here) is to give the name of the closest living counterpart in parentheses, for example, Pinus (strobus) .
Most of the fossil floras are from freshwater sediments and are thus biased in favor of local lakeshore or stream bank plants. Also there is bias in favor of species producing large, tough leaves or other organs capable of leaving a good impression in sediment. On the basis of sediment bedding and texture, Axelrod (1958a, 1980) has in some cases been able to discriminate between fossils from local riparian vegetation and material transported from elsewhere by floods and mudflows.
Dating of Neogene floras is currently in a state of flux. Recent advances in dating marine sediments have led to drastic revision of the Neogene time scale and to a shift of the Miocene–Pliocene border to a much later date. The terrestrial and marine chronologies are not yet well integrated. Time correlations between fossil floras of different sites are often controversial except in the few cases where radiometric dates have been established.
By the beginning of the Neogene, the global map of continents and
oceans was beginning to resemble its modern pattern, but some fossil floras deposited in Neogene time have since been significantly displaced in latitude or elevation. Also, major changes in ocean temperatures, rain shadows behind mountain barriers, and coastlines make reconstruction of paleoclimates a formidable problem, one which will not be considered here.
North-Central Europe
(Axelrod 1983b ; Butzer 1971; Ferguson 1971; Frenzel 1968; Hammen et al. 1971; Mädler 1939; Szafer 1966)
North-central Europe had a wonderfully rich Neogene forest flora of both conifers and broadleaf hardwoods. Listing of genera (Table 4) understates the richness because many genera were represented by multiple species. Nearly all these genera are considered members of the Arcto-Tertiary Geoflora, which was shared among all the northern continents. Where its members originated and their directions of migration are not known. Very few of them appear to be capable of ocean crossings. A few probably had been shared between Europe and eastern North America before formation of the North Atlantic Ocean in the Paleogene. Exchanges between Europe and Asia may have occurred repeatedly during both Paleogene and Neogene.
Various floras from Poland, Germany, and the Netherlands, from which Table 4 is compiled, suggest that this rich forest flora persisted into the early Pliocene, when it was decimated during the Villafranchian, a time with predominantly heath vegetation. Reforestation occurred during the succeeding warmer Tegelen period, but few tree species returned, although the high alpine barrier to migration did not yet exist. In the Netherlands, about 80% of the species present in the rich early Pliocene Reuverian flora but only about 20% of the species in the impoverished late Pliocene Tegelen flora are now extinct in the region. The late Pliocene Ludhamian flora of Britain was dominated by genera still common there, for example, Pinus, Alnus, Betula, and Quercus ; it had only a few genera that have since become extinct in the region, for example, Tsuga and Pterocarya . Thus, the so-called modernization of the European flora by regional extinction, although not completed until the Pleistocene, was well underway during the Pliocene. Most of the extinct species or close relatives survive elsewhere; for example, North America has living counterparts of the fossil European Pinus (strobus) and Populus (balsamifera) and eastern Asia has living counterparts of fossil Acer (palmatum) and Quercus (serrata) .
Freshwater aquatic species may have suffered less Pliocene and later extinctions in north-central Europe than forest trees did. Szafer (1946) reported
|
early Pliocene fossils in Poland of 23 species of freshwater aquatic and marsh plants. One water lily, a species of Euryale, is now extinct in Europe and survives only in eastern Asia. All the other species belong to genera that survive in the region, for example, Ceratophyllum, Najas, Sagittaria, Trapa, Carex, and Polygonum ; Szafer assigned 16 of these fossils to living northern European species.
Western North America
(Axelrod 1958a,b, 1973, 1980, 1983b, 1985a, Axelrod and Raven 1985; Cross and Taggart 1982; Leopold 1969; Raven and Axelrod 1974; Thorne 1978; Wolfe 1972; Wolfe and Tanai 1980)
The rich macrofossil record of western North America has long been studied by an outstanding corps of paleobotanists. Parts of the region have fossil beds preserved in ash falls and lake basins created by volcanic and
|
tectonic activity. These may record not only stable vegetation controlled by climate and topography but also temporary successional series. The region is so vast and diverse and the fossil record so discontinuous that only a tentative and sketchy migrational story has yet emerged.
In the Miocene time, the woody flora of the region included a great majority of the genera that are important there today and many others that no longer survive there (Table 5). For broad generalization, it is useful to separate Arcto-Tertiary and Madro-Tertiary floras, although the fossil record indicates that, then as now, species ranged individually and formed kaleidoscopic rather than constant associations. Many species, however, evidently had broader and more continuous ranges then because the present great mountain ranges did not yet exist.
|
Arcto-Tertiary Flora
All but a few of the gymnosperms and deciduous angiosperms in Table 5 are classified in the Arcto-Tertiary group. These genera had spread from uncertain origins all around the northern hemisphere during Paleogene time. Few if any of them appear to have been capable of ocean crossings. Some presumably had crossed between Europe and North America before the North Atlantic Ocean barrier developed in the Paleogene. Contacts across Beringia may have continued intermittently during the Miocene. Especially close relationships between eastern Asian and western North American members of the Arcto-Tertiary forest floras have long been recognized. Recent chemotaxonomic study has shown that Miocene fossils from Oregon of several Arcto-Tertiary genera have organic compounds more similar to living eastern Asian than to eastern North American congeners (Niklas and Giannasi 1978). Different authorities, including Batchelor (1979) and Frakes (1979), have irreconcilably different concepts of eustatic sea level changes during this time.
During the early Miocene, from about 25 to 15 million years ago, a rich assortment of Arcto-Tertiary conifers and deciduous hardwoods ranged from Alaska, and perhaps a Bering land bridge, down to northern California and inland to Idaho and northern Nevada. They did not comprise a homogeneous vegetation. Lowlands were presumably dominated by broadleaved evergreen and deciduous hardwoods, many of which are no longer present in the region. Also present were some conifers, such as Glyptostrobus and Metasequoia , that are no longer present there. Higher elevations were dominated by confier genera, some of which are still present there, such as Abies, Picea, Pinus, Pseudotsuga , and Sequoia. Abies, Picea , and Pinus forests were more extensive inland in Montana and Wyoming, although the major uplift of the modern Rocky Mountains had not yet begun. A few deciduous Arcto-Tertiary genera, including Metasequoia, Carya, Pterocarya, Sassafras , and Ulmus , that have since become extinct in the region were still present locally in early Miocene time.
At the same time, in central California and west central Nevada, the Arcto-Tertiary and Madro-Tertiary genera mingled in a mosaic, with the Arcto-Tertiary flora presumably occupying the cooler north slopes and higher elevations. Pure conifer forests may have been confined to isolated high volcanic peaks. In Miocene southern California, the Arcto-Tertiary flora was evidently represented only by riparian deciduous hardwoods, such as Acer, Alnus, Fraxinus, Platanus, Populus , and Salix , all of which have close modern relatives in the region, and by one extinct species, Ulmus (americana) .
Neogene migrations of the Arcto-Tertiary flora consisted mainly of retreat to disjunct relict areas and local extinctions, which Axelrod (1985a ) has
attributed mainly to decreasing summer rainfall due to ocean cooling reinforced in places by rising topography and rainshadows. Retreats were most drastic in Alaska, where few hardwood trees were left by late Miocene time except riparian Alnus and Salix . By late Miocene time, parts of Alaska had glaciers down to sea level, and above 65°N, taiga, tundra, and heath had taken over. Although probably open, the Bering land bridge was evidently no longer a pathway for intercontinental tree migration. Beginning about 14 million years ago forests in the Pacific Northwest, from Washington and Idaho to central California and central Nevada, became impoverished as many species retreated to maritime coastal regions, rising interior mountains, and riparian sites. Of the Arcto-Tertiary genera that have become extinct in the Pacific Northwest since the early Miocene, none of the conifers and only a few of the deciduous hardwoods are known to have survived after the Pliocene. However, the fossil record is so fragmentary that relicts may have persisted without being recorded.
Madro-Tertiary Flora
The woody flora at the start of Neogene time also included many genera that evidently originated and remained in North America and Mexico, being unable to penetrate high enough latitudes to use Tertiary land connections to Eurasia around either the North Atlantic or North Pacific. This Madro-Tertiary group includes nearly all the evergreen angiosperm genera listed in Table 5, which are still important members of the modern sclerophyll woodlands and chaparral in the region. The group also includes the deciduous angiosperms that survive in interior arid areas of the region (Table 5); these are members of modern thorn scrub and riparian desert vegetation. This flora also includes a few miscellaneous angiosperm genera no longer locally present but surviving in Mexico. Some of these genera are strictly confined to tropical lowlands today, such as Cedrela , a tall, compound-leaved deciduous tree of Mesoamerica.
During early Miocene time, some Madro-Tertiary species ranged much farther north than their surviving relatives. For example, in the Pacific Northwest's present Cascade Mountain region, the Arcto-Tertiary flora commonly had an admixture of Madro-Tertiary evergreen broadleaf trees and chaparral species, including Quercus (chrysolepis), Lithocarpus (densiflora), Arbutus (arizonica), Persea (borbonia), Heteromeles (arbutifolia), Ceanothus (cuneatus), Cerocarpus (betuloides) , and the broadleaf deciduous Cedrela (odorata) . During the Pliocene in central California, some Madro—Tertiary sclerophyll species still grew north of the ranges of their present relatives, Quercus (engelmannii, tomentella), Rhus (laurina, ovata), Ceanothus (spinosus) , and Lyonothamnus (floribundus) .
|
During early Miocene time, Madro-Tertiary sclerophyll woodland and chaparral species also ranged farther east than their surviving relatives. For example, in west-central Nevada, areas now dominated by desert shrubs and sagebrush had early Miocene floras including all the species noted in the preceding paragraph except Cedrela and Quercus (tomentella) . These fossil floras also included several other sclerophyll tree and shrub genera now confined to the west side of the Sierra Nevada.
In late Miocene time, the general level of the incipient Sierra Nevada was probably still 2,000 m lower than today. The 7-million-year-old Mount Reba flora, from a site now near timberline at 2,650 m, was dominated by Madro-Tertiary sclerophyll woodland species whose modern equivalents are present at about 600 m elevation in the adjacent foothills. Evidently, development of the intense Great Basin rainshadow and spread of desert flora postdate the Miocene. There is no Pliocene fossil record from Nevada.
Most of what is now southern California lay beneath the sea through the Neogene. The few Miocene floras known from the region all evidently grew at low or medium elevations and lack the montane conifers now present in the region. Along with the few deciduous riparian trees noted above, Miocene floras included a variety of chaparral, sclerophyll woodland, and desert shrub genera, mostly with close relatives surviving in the region (Table 6). Considering how much time and tectonic change has ensued, the Miocene floras were amazingly modern; by comparison the contemporary fossil fauna
included many strange, extinct genera. Also, Miocene floras found west of the San Andreas fault actually grew in much lower latitudes. For example, the Mint Canyon flora may have been displaced 200 km northward from its original location. This might be expected to explain the presence of xerophytic fossil plants north of the present limits of Mexican relatives (Table 6), but the same xerophytic species were present in Miocene floras found east of the fault and not displaced. Two species, Lyonothamnus (floribundus) and Prunus (lyonii) that were present on the southern California mainland during the Miocene have surviving relatives only on the offshore islands. When the retreat of the xerophytic and island species began is not known. Early, middle, and late Miocene floras from the region are very similar, and there is no Pliocene record from the region.
Middle America
(Graham 1973, 1976S, 1982)
Pollen records from Mexico and Central America suggest southeastward migration during the Miocene along the Cordilleran axis of many Arcto-Tertiary tree genera: Abies, Picea, Pinus, Alnus, Fagus, Juglans, Liquidambar, Populus , and Ulmus . All of these appeared in Vera Cruz, on the Mexican Gulf coast, during the Miocene. They seem to have formed a lowland forest of mixed conifers and broadleaf deciduous trees adjacent to coastal mangroves with no sign of intervening tropical lowland vegetation. Little work has been done, however, on the pollen signatures of modern tropical lowland forests. It is conceivable that insect-pollinated trees could be present in a region and leave little trace in the pollen record. In Vera Cruz at present, the mangroves grow next to extensive rainforest; the Arcto-Tertiary genera are still present in southern Mexico but grow high in the mountains and in different associations.
The Cordilleran system was being uplifted during the Miocene, and by late Miocene time the Panama land bridge had probably formed. Fossil pollen indicates that Alnus and Juglans had reached Panama during the Miocene and entered northern South America during the Pliocene.
9
The Deep Past
The Neogene and later periods discussed above cover less than one-tenth of the time that seed plants have existed. Their fossil record, which has great gaps in space and time, began in the Devonian Period (Table 7), more than 300 million years before the Neogene. Unlike the Neogene, for which nearly all fossil plants can be identified to living genera, if not species, the of characters becomes increasingly strange with greater age. More often than not, it is highly speculative whether these strange creatures were evolutionary dead ends or primitive ancestors of much changed modern descendants. Moreover, paleontologists not only have to deal with strange creatures, they usually have only bits of creatures that nobody has seen whole. Separate taxonomies have to be constructed for pollen, leaves, stems, seeds, and other organs. Articulating them is a great accomplishment. For example, the existence of a great extinct order, the bizarre seed ferns or pteridosperms, was not revealed until it was finally concluded that fossil seeds, long named Lagenostoma, and fernlike foliage, named Lyginopteris, belonged to the same plant.
Also, research on early seed plants has necessarily been partitioned among specialists in different taxonomic groups whose first task was to work out evolutionary relationships of particular lineages. Only gradually have plant geographic syntheses emerged.
For this time span, another change in approach to migrations is necessary. There is no hope of tracing geographic changes on the species level. Even for higher taxa, information on geographic distributions is generally rudimentary. All that can be attempted here is to seek evidence of really grand migrations of higher taxa. This will be done chronologically, starting with the origins of the first seed plants. Some notice will be taken of continental movements, but for all of the dazzling light that plate tectonics has shed on
|
Table continued on next page
|
geomorphology, it is still of limited use in reconstructing plant migrations. Contact between tectonic plates is irrelevant if the continental margins were submerged. A shallow sea is as much a barrier for land plants incapable of long-range dispersal as is a deep ocean.
Paleozoic And Mesozoic Gymnosperms
(Arnold 1969; Axelrod 1974; Bambach et al. 1980; Barnard 1973; Beck 1976; Brenner 1976; Chaloner and Lacey 1973; Chaloner and Meyen 1973; Douglas and Williams 1982; Florin 1963; Hallam 1977; Hughes 1976; Mamay 1976; Miller 1977; Penny 1969; Phillips et al. 1985; Plumstead 1973; Rabinowitz et al. 1983; Retallack and Dilcher 1981a ; Roche 1974; Stewart 1983; Taylor 1981)
Origin of Gymnosperms
About 3 billion years passed between the appearance in the fossil record of the first plants with chlorophyll, the cyanobacteria or blue-green algae, and the appearance of the first vascular land plants, the Silurian pteridophytes.
These non-seed-bearing pteridophytes diversified during the Devonian into several major lineages, commonly interpreted as having surviving members in the whiskferns, horsetails, clubmosses, and ferns. All these evolved complex vascular diploid bodies, which in some cases were large trees. Once established, a diploid pteridophyte is well fitted for survival on land. Also, the microscopic haploid spores, which pteridophytes produce in huge quantities, are well adapted to long-range wind dispersal. After the spores land on the ground and germinate, however, the ensuing haploid stages, including a swimming sperm, are not much better fitted for land life than their algal ancestors.
Evolution by the gymnosperms of pollen and seeds eliminated the weak links in the pteridophyte life cycle for sexual reproduction on land. The evolutionary steps were complex, but the net result was simply sheltering and nurturing the vulnerable stages within the body tissues of the mature vascular diploid. Gymnosperm cones are organs of the diploid plant and are supplied with water and food by its vascular tissues. The only stage of the sexual reproductive cycle that has to exist out in the world on its own is the haploid pollen. The target of a pollen grain, unlike a fern spore, is not the ground but an opening in the integument surrounding the young seed on a female cone. There it can deliver the sperm to the egg without external water.
Origin of the gymnosperms came remarkably soon after the origin of their pteridophyte ancestors. An intermediate group, the progymnosperms, appeared in the Devonian in what is now North America and Europe. The geography of the Paleozoic world is still very speculative, but reconstructions for the Devonian and early Carboniferous commonly show North America, Greenland, and Europe united as a single continent, called Euramerica, which was located in low latitudes and separated by oceans from proto-Asia and Gondwanaland. The oceans had evidently not barred pteridophyte migrations; from the Silurian to the Carboniferous, pteridophyte floras had fairly uniform global distributions. The seeds of early gymnosperms, however, like those of most modern ones, were evidently poorly equipped for long-range wind or water dispersal. Although gymnosperm pollen grains are capable of long-range wind dispersal, this serves only to maintain gene flow between already established disjunct populations of a species, not to found a new population.
So far as is known at present, the first gymnosperms were confined to Euramerica. The first named seed plant species, Archaeosperma arnoldii , appeared there in Late Devonian time. Its seeds were borne in pairs on open, cuplike structures arranged more loosely than in the tightly condensed cones of later gymnosperms, and there was a larger opening in the integument for entry of the pollen than in later gymnosperms.
Carboniferous
The Carboniferous is named for the remains of the great swamp forests laid down as coal. In both Europe and North America, the abundant fossil record shows that these swamps were dominated by pteridophyte trees, with gymnosperms as subordinate associates. The nature of the upland flora, if any, is not known.
The gymnosperms of the Early Carboniferous (or Mississippian) already represented several families within the extinct order of Pteridospermales or seed ferns. Two other gymnosperm orders, presumably also derived from the progymnosperms, namely the Cordaitales and Voltziales, became widespread in both midcontinental North America and western Europe in the late Carboniferous (Pennsylvanian). Both were structurally closer to modern conifers than to ferns.
Some paleogeographic reconstructions show Pangea beginning to be assembled in the Late Carboniferous, with proto-Asia and Gondwanaland approaching or contacting Euramerica. This would account for reports of Pennsylvanian age gymnosperms of all three orders in parts of Asia and Gondwanaland, although dating and identity of these fossils is not very clear. Even if Pangea was assembled, there were great barriers to seed plant migration during the Pennsylvanian because of extensive marine transgressions and, in Gondwanaland, extensive glaciation.
Permian
During Permian time, Pangea was complete, with broad contacts near the equator between Euramerica and western Gondwanaland. A single world ocean extended from pole to pole and spanned 300° of longitude at the equator. The interior of the continent must have had great deserts, particularly behind upthrust mountains; shallow inland seas created other barriers to seed plant migration. In spite of contacts between continental plates, the Permian had regional floristic differences.
The three Carboniferous gymnosperm orders, Pteridospermales, Cordaitales, and Voltziales, survived in the Permian in Euramerica and were joined by probable primitive representatives of two living gymnosperm orders, Cycadales and Ginkgoales. During the Permian, all five lineages appeared in Asia and Gondwanaland, where they were mainly represented by different genera than in Euramerica. The Cordaitales became extinct late in the Permian.
The most famous Gondwana gymnosperm genus is Glossopteris , which
can be classified either as a member of the Pteridospermales or in an order of its own. Glossopteris was a tree with trunks as large as 40 cm in diameter and with narrow, tongue-shaped leaves. It dominated coastal swamps that accumulated to form much of the commercial coal of the southern hemisphere, uplands being occupied by other gymnosperms. In the Permian, Glossopteris spread over huge areas of present South America, Africa, India, Antarctica, and Australia that had been glaciated during the Carboniferous. The paleolatitudes within which Glossopteris grew ranged from about 40°S to almost the Permian south pole (fig. 13). It is not known whether the trees were evergreen or deciduous, but in any case, it is hard to account for their survival in nearly six months without sun.
Triassic and Jurassic
The Mesozoic began with Pangea fully assembled and with low sea levels. During Triassic time, the Tethys Sea formed between the single northern continent, Laurasia, and Gondwanaland, but it remained narrow in places; there may have been intermittent contacts between the northern and southern continents during much of the Mesozoic. By the Middle Jurassic, sea level had risen and shallow continental seas separated North America—Greenland from Europe and South America from Africa, at least intermittently. Also during the Jurassic, India-Madagascar may have already broken away from Africa.
Glossopteris survived through the Triassic but died out early in the Jurassic, ending one pteridosperm lineage. Two other now extinct orders possibly derived from the pteridosperms, namely the Caytoniales and the Bennettitales, arose during the Triassic. The Caytoniales had a fleshy tissue partly enclosing the seed that suggests animal dispersal. One genus of Caytoniales, Lepidopteris , had a remarkable range across Laurasia and Gondwanaland in paleolatitudes from 60°N to 60°S. The Bennettitales, which superficially resemble cycads, had spectacular reproductive structures that suggest adaptation for animal pollination.
The two Paleozoic gymnosperm orders that survive today, Cycadales and Ginkgoales, spread widely through both Laurasia and Gondwanaland in the Mesozoic, increasing greatly in abundance and diversity. Some Jurassic Ginkgo leaves are indistinguishable from those of the living G. biloba, and some of the cycads were already similar to living genera.
Two other living orders of gymnosperms, Coniferales and Taxales, arose in the Mesozoic not long before the Voltziales, their probable progenitors, became extinct. Five important modern conifer families had evidently already diverged by the Early Jurassic: Pinaceae in Laurasia, Podocarpaceae in Gondwanaland, and Araucariaceae, Cupressaceae, and Taxodiaceae on both
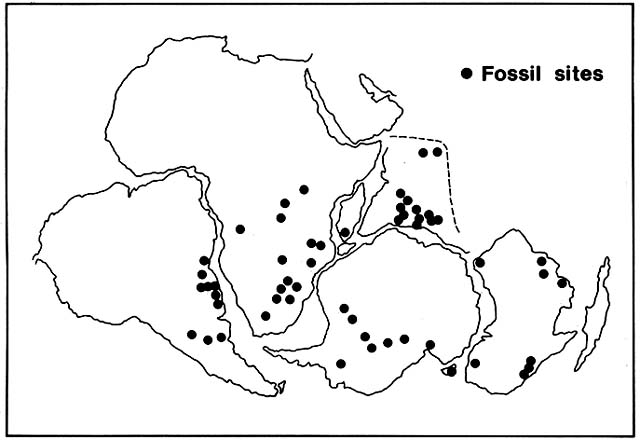
Figure 13. Late Paleozoic Range of Glossopteris , a Dominant Seedfern on the Supercontinent of Gondwanaland.
Gondwanaland broke up into several continents, outlined by dashed lines. Fossils of Glossopteris were then
displaced to widely separated parts of the globe. Since the genus evidently was not adapted for transoceanic
dispersal, its fossil distribution appears inexplicable on an earth with fixed continents. This was noted as evidence
for the hypothesis of continental drift long before the modern theory of seafloor spreading was developed.
continents. During the Jurassic, the Taxales, precursors of the living yew, entered the fossil record in Laurasia.
Cretaceous
The Tethys Sea continued to be a partial barrier between the northern and southern continents during Cretaceous time, but there were intermittent contacts between Europe and Africa and between North and South America. During the Early Cretaceous, the incipient Indian and South Atlantic oceans began to dissect Gondwanaland, but separation was incomplete except for isolation of India–Madagascar during most of the Cretaceous. By the Santonian, Africa was an island, but South America may still have been linked via Antarctica with Australia and New Zealand. By the Maestrichtian, the South Atlantic had widened to about 800 km between Brazil and Africa, but there had been little seafloor spreading in the North Atlantic. North America, Greenland, and Europe were still in contact north of 50°N latitude. At higher latitudes, Alaska and Asia had been in contact since the Coniacian. Laurasia, however, was divided during much of the Cretaceous by marine transgressions. Although the extensive Jurassic epicontinental seas had receded at the start of the Cretaceous, the regression was temporary. Seaways extending from the Tethys Sea to the Arctic Ocean bisected both North America and Eurasia from mid-Cretaceous until they withdrew in the Maestrichtian.
Two Triassic gymnosperm orders; the Caytoniales and Bennettitales, remained widespread in the Early Cretaceous but were extinct by the end of the Cretaceous.
Cycadales and Ginkgoales continued to be widespread throughout the Cretaceous, ranging to amazingly high latitudes in both northern and southern continents. The Taxales remained confined to Laurasia.
Among the Coniferales, the Pinaceae were represented by fossils confined to Laurasia; extant genera represented included Pinus and possibly Cedrus and Larix . The Podocarpaceae continued to be widespread in parts of former Gondwanaland, excluding Africa; fossils are commonly identified with living genera, especially Dacrydium and Podocarpus . The Araucariaceae, Cupressaceae, and Taxodiaceae continued their Jurassic pattern of wide ranges in both northern and southern continents. Jefferson (1982) described a puzzling fossil forest of conifers resembling Araucaria that lived during the Early Cretaceous in Antarctica at a paleolatitude of about 70°S. The trees were buried by volcaniclastic deposits and remained standing and rooted. The trees must have had problems of shading under the low sun angle, but they were closely spaced and had an understory of pteridophyte vegetation. Also, their well-defined growth rings show that annual growth was at a rate that would be very high for modern conifers, even at low latitudes.
Among the Taxodiaceae, fossils tentatively assigned to Sequoiadendron , which survives only in California, were deposited in eastern North America and Greenland in Early Cretaceous time (Florin 1963). By Late Cretaceous, this taxon ranged to Europe and western North America. Metasequoia , which survives only in China, had a coherent Cretaceous range from eastern Asia through Alaska to western North America. Sequoia , now confined to western North America, evidently originated there, perhaps by hybridization between Sequoiadendron and Metasequoia . Various other genera of Taxodiaceae had close Cretaceous precursors. Cryptomeria, Cunninghamia, Glyptostrobus , and Sciadopitys , which survive only in eastern Asia, had Cretaceous precursors in widely scattered sites in Eurasia, North America, and Greenland. Arthrotaxis , which survives only in Tasmania, had Cretaceous precursors in New Zealand and South America.
Cretaceous Angiosperms
(Axelrod and Raven 1978; Batten 1981; Beck 1976; Brenner 1976; Dilcher and Crane 1984, 1985; Doyle 1973, 1977; Doyle and Hickey 1976; Hickey 1977; Hughes 1976; Muller 1970, 1981; Retallack and Dilcher 1981a,b ; Srivastava 1981; Tralau 1973; Tschudy and Scott 1969; Walker and Doyle 1975; Walker et al. 1983)
The origin of the angiosperms has long been one of the most baffling problems in paleobotany. Various fossil gymnosperm orders and all living ones except the conifers have been suggested as possible progenitors, but missing links in the fossil record make all the alternatives highly speculative. Until recently it was generally supposed that the angiosperms originated someplace where there was no fossil record and underwent major phylogenetic diversification, including initiation of many living families, before entering the fossil record. Major theories of angiosperm phylogeny have been built almost entirely by deductive comparisons between living groups, with any relevant fossil evidence considered to be simply missing.
Recently, paleobotanists have launched a new attack on the problem of Cretaceous angiosperm evolution, and hopes are currently high that the fossil record is much more complete than had been realized. As noted by Wolfe (1973), the amount of missing record may have been greatly exaggerated by a practice by pioneer paleobotanists of seeking resemblances between Cretaceous leaf fossils and living genera and assigning the fossil to whatever modern family offered the closest resemblance. This practice has worked well for Neogene and Pleistocene woody species that have undergone very little subsequent evolution or extinction. In these relatively young fossil floras,
identifications based on leaves, other macrofossils, and pollen have all fit together nicely. Identifications of Cretaceous leaves with modern taxa, however, have not generally been supported by other fossils, and resemblances may be merely superficial.
Instead of leaping to identify primitive angiosperm fossils with familiar names, efforts are now being made to work forward from the beginning of the record in early Cretaceous, to build an independent taxonomy of initially strange pollen and macrofossils, which are not expected to have already become stabilized in their present forms. Pollen is being given special attention because it provides a more abundant and complete sampling of the flora from a catchment area larger than that of the macrofossils. By comparative study of enough floras worked up in this way, it may eventually become possible to trace angiosperm evolution and migration through the Cretaceous and to follow some lineages to extinction and others to recognizable modern descendants. Only glimpses of such a story are now possible.
Neocomian
The earliest generally accepted fossil evidence of an angiosperm comes from beds deposited over 100 million years ago in Siberia; the fossil consists of small, pinnately veined, simple leaves, presumably from a dicot. How far this plant had proceeded in transition from gymnosperm to angiosperm reproductive structures is unknown.
Barremian
A sparse macrofossil record of apparent monocot and dicot leaves and of dicot fruits and a more abundant pollen record suggesting both monocots and dicots are reported from both sides of the Tethys Sea in North America, Europe, Siberia, South America, and Africa. The angiosperm fossils were deposited in sediments indicating that they inhabited shores of lagoons, estuaries, and other sites disturbed by coastal erosion and deposition. Retallack and Dilcher (1981b ) suggested that the angiosperms originated as coastal pioneers capable of rapid migration by wind and water dispersal. The ocean may have served more as a highway than as a barrier to migration, as is true of later seashore angiosperms, which typically have seeds or fruits dispersed by ocean currents. Because of the pervasive symbioses between angiosperms and animal pollinators and seed dispersers, it has been widely assumed that the very origin of angiospermy involved coevolution with animals, for example, that the ovary served initially to protect the young ovules from chewing insects visiting the flowers. If the angiosperms really arose as coastal pioneers,
perhaps the ovary served initially for sea dispersal and coevolution with animals was secondary.
Barremian pollen attributed to angiosperms include a probable monocot form, Liliacidites , and a probable dicot form, Clavatipollenites . Both resemble gymnosperm pollen in being monosulcate, that is, having a single germination furrow, but differ from gymnosperm pollen in having intricately sculptured instead of smooth coats. This sculpturing may have adapted the pollen grain to adhere to the body of an insect or to the stigma of a flower. The latter would mean that the plants had crossed the crucial line defining angiospermy, that is, the ovules were no longer exposed to direct pollination but had to be reached by growth of a long pollen tube penetrating the ovary from a stigma. Among living angiosperms similar primitive monosulcate pollen is produced by many monocots and by one of the six subclasses of dicots, namely, the Magnoliidae, which include Magnoliaceae, Lauraceae, Nymphaeaceae, and related families.
Aptian
Monosulcate angiosperm pollen continued to be widespread in both the northern and southern hemispheres. In Gondwanaland, it was joined by the first tricolpate pollen, that is, grains with three germination furrows, almost certain proof of angiospermy. In the moist pollen chamber of a gymnosperm ovule, a single germination locus suffices, but on the exposed stigma of an angiosperm flower, multiple pores on different sides of the grain are highly advantageous. Aptian tricolpate pollen has been found in Israel in sediments deposited at low latitudes on the Gondwanaland coast and also in sediments deposited along embayments in the rift valley system lying between what is now equatorial Africa and South America. Tricolpate pollen and forms with similar names derived from it by elaboration and modification are characteristic of all the six subclasses of dicots except the Magnoliidae.
Albian
During the Albian, tricolpate and derivative pollen types diversified vigorously, not only in Gondwanaland but also north of the Tethys Sea in both western and eastern North America and in Europe. Later in the Albian, angiosperms evidently spread to higher latitudes than ever before in both Laurasia and Gondwanaland; this expansion is indicated by both monosulcate and tricolpate pollen and by macrofossils. In North America and Europe, dicot leaves became much more diverse; in addition to forms that suggest Magnoliidae, leaves appeared that may represent other dicot subclasses, such
as Hamamelidae, exemplified by Platanus . Actual fossilized flowers are known from the Albian of North America and Europe; they indicate divergent adaptations for insect and wind pollination. Some are bisexual, with large, showy petals and many ovules, closely resembling Magnolia . Some are bisexual and have small, delicate petals, probable nectaries, and few ovules, closely resembling Pyrus , the apple. Some are unisexual, tiny, inconspicuous, and grouped in inflorescences strikingly similar to Platanus .
Generally angiosperms were still subordinate to gymnosperms and ferns except in coastal and riparian sites. In North America, angiosperms penetrated from the Gulf Coast to Alberta along the shores of Albian epicontinental seas and also first appeared in Alaska along shifting seashores. In river deposits, there was some segregation of fossils in different kinds of alluvium, ferns and gymnosperms being found in fine sediments and angiosperms being relatively abundant in coarser sediments deposited by high-energy streams. The leaves attributed to Platanus were commonly buried in sand.
Cenomanian
Patterns changed only slowly. Until late Cenomanian time, angiosperm pollen generally remained rare compared to gymnosperm pollen, especially in Gondwanaland. Retallack and Dilcher (1981b ) concluded that angiosperms were still mainly confined to coastal and riparian habitats, growing as mangroves along tidal distributaries, in swamps along lagoon margins, as scrub on natural levees, and as floating aquatics. Some of the richest Cenomanian fossil beds are in the Dakota Formation of Kansas, laid down in a variety of coastal and deltaic environments. Angiosperm leaf fossils there and elsewhere in North America and Europe show increasing diversity. Many have been questionably identified with living families.
Tricolpate dicot pollen continued to be widespread and appeared for the first time in high arctic latitudes. A derivative type, Normapolles , first appeared in the Cenomanian in eastern North America and Europe. It has been interpreted as signaling a reversion to wind pollination, perhaps by Fagaceae. Wind pollination implies large stands of a species, not just scattered seashore or streambank pioneers.
Turonian
Eastern North America and Europe were still in contact at high latitudes in Turonian time. They retained pollen floras that were broadly similar but already had distinct genera. In both regions Normapolles diversified rapidly. There, for the first time, angiosperm pollen was abundant relative to gymnosperm
pollen, suggesting extensive hardwood forests. The ancient, primitive Clavatipollenites had become rare except in Australia. Gondwanaland remained conifer dominated.
Coniacean—Campanian
Normapolles pollen, presumably from hardwood forests, continued to dominate eastern North America, Greenland, and Europe, which were still in contact during Coniacean—Campanian time. Western North America and eastern Asia had evidently come into contact; they shared a newly evolved pollen assemblage called Aquilapollenites . Also derived from the tricolpate type, Aquilapollenites was presumably dicot pollen, but it has no living counterpart. Some Aquilapollenites also appeared in Gondwanaland during this period. Another new derivative of the tricolpate dicot type, Proteacidites, appeared in Australia in the Coniacean; this may mark the appearance of the Proteaceae, another family in the Rosidae. The Proteaceae are a classic example of a group whose modern distribution suggests a Gondwanaland origin. In the Coniacean, it would have still been possible for the Proteaceae to migrate over land between Australia and Africa. By the Santonian, Africa was isolated.
The Gondwanaland migrations of the southern beech, Nothofagus, are especially intriguing (Axelrod and Raven 1982; Schuster 1976). A member of the Fagaceae and almost certainly originally from the northern hemisphere, Nothofagus appeared in the fossil record in South America, Australia, and New Zealand in Senonian time. Its progenitor had evidently migrated from North to South America during one of the intermittent Cretaceous contacts and had continued through Gondwanaland before the link between South America and Australia via Antarctica was broken. It is unlikely that Nothofagus could have made this migration except over a continuous land path. The seed dispersal radius of living Nothofagus is very short, and the trees are dependent on symbiotic mycorrhizal fungi that are not dispersed with the seed.
Maestrichtian
The sharp distinction between the angiosperm floras of eastern North America—Europe and western North America—eastern Asia lasted into the early Maestrichtian. The discreteness of these provinces was destroyed in the late Maestrichtian, when the epicontinental seas that had bisected the continents withdrew. The pollen record then shows general mixing of Normapolles and Aquilapollenites taxa.
From the Maestrichtian on, paleobotanists are less cautious about assigning
angiosperm fossils to living families and even genera. Muller (1981) recognized 31 extant families as present in the Maestrichtian, including those noted above as present in earlier periods.
It is customary to characterize Cretaceous angiosperm evolution as explosive, with some justification. Hughes (1976) estimated that at the start of the Cretaceous, the world had about 3,000 species of vascular plants, roughly half of them ferns and half gymnosperms. He estimated that by the Maestrichtian, there were about 2,500 surviving ferns and gymnosperms and about 20,000 species of angiosperms. The explosion had scarcely begun then; the world now has about 285,000 angiosperm species in about 300 families.
The diversification of the angiosperms is commonly credited to their specialized symbioses with animal pollinators and seed dispersers. Escape from wind pollination and wind dispersal allowed origin and survival of species growing in special scattered habitats and forming complex associations rather than dominating extensive, simple stands. What little evidence there is on Cretaceous angiosperm reproduction, however, suggests that angiosperms were then mostly generalists, with pollen dispersed by wind and unspecialized insects and seeds dispersed largely by wind and water. By the Maestrichtian, some coadaptation for pollination and dispersal by specific animal groups was evidently finally beginning, but major diversification of the most important modern pollinators and dispersers, namely butterflies, moths, bees, birds, and bats, was still far in the future.
Cretaceous—Tertiary Transition
(Hickey 1984; Pillmore et al. 1984; Smit and Kaars 1984; Tschudy and Tschudy 1986; Wolfe and Upchurch in press)
Controversy is currently very active over a hypothesis that the Cretaceous ended with a bang: a huge asteroid impact caused clouds of debris to darken the earth and shut down photosynthesis, resulting in mass mortality and extinctions of many marine and terrestrial species. There is no doubt that there were major changes in flora and fauna at the Cretaceous—Tertiary transition. The argument is over the causes and whether the changes were nearly instantaneous or spread out over perhaps 10,000 to 100,000 years or more.
So far, only a little evidence on both sides of the controversy has been adduced from the fossil record of seed plants.
Evidence from various sites in the Rocky Mountain region suggests that several fossil pollen taxa, including most kinds of Aquilapollenites, disappeared abruptly at the end of the Cretaceous. Disappearance of the angiosperm
pollen was followed by a temporary increase of fern spores from less than 30% to nearly 90% of the microfossil record. According to Wolfe and Upchurch (in press), the subsequent return to dominance of angiosperms followed a pattern of normal ecological succession but at a slower rate; this implies migration from survivors outside the region.
Axelrod (personal communication 1985) suggested that such changes might have been due to regional volcanic ashfalls rather than a global phenomenon. Hickey (1984) described floristic changes at the Cretaceous—Tertiary transition as geographically variable and gradual. Aquilapollenites taxa reached their peak diversity at the end of the Maestrichtian, but their decline extended through the Paleocene until extinction in the Eocene, a span of about 10 million years. In many other regions of the world, Hickey (1984) described floristic changes at the end of the Cretaceous as slight to moderate, with Cretaceous plant species persisting after dinosaur extinctions.
The argument is not over.
Paleogene (Paleocene, Eocene, And Oligocene
(Axelrod 1984; Axelrod and Raven 1978, 1985; Daghlian 1981; Dilcher 1973; Germeraad et al. 1968; Hickey 1984; Hos 1975; Kemp and Harris 1975; Lakhanpal 1970; Leopold 1969; Muller 1981; Penny 1969; Raven and Axelrod 1974; Stebbins 1981; Tanai 1972; Tiffney 1985; Tschudy 1984; Wolfe 1972)
Whatever extinctions took place at the Cretaceous—Tertiary transition, a variety of seed plant lineages came through and radiated rapidly in the Paleogene, when a great many living genera of conifers and angiosperms first appear in the fossil record.
The global configuration of continental contacts and separations changed drastically during the Paleogene. The North Atlantic continued to drive an oceanic wedge northward, and by the Oligocene, North America—Greenland and Europe were separated. Asia and Alaska, however, remained in contact, sea levels remained low, and there may have been no other major oceanic barriers to migration in Laurasia. In contrast, the breakup of Gondwanaland became complete during the Paleogene. Africa was an island through the whole period, except for possible brief contact with Eurasia. India separated from Madagascar early in the Paleocene and moved northward to collide with Asia in the Eocene. By late Eocene or early Oligocene, South America, Antarctica, and Australia had separated and Australia was moving into lower latitudes.
For coastal angiosperms, the oceans probably continued to be pathways
of migration rather than barriers. For example, Nypa, a mangrove swamp palm, which had first entered the fossil record in the East Indies in Late Cretaceous time, evidently migrated westward by the Tethys Sea route and across the widening Atlantic Ocean. In the Paleocene and Eocene, Nypa pollen and its sea-borne fruits were present in Southeast Asia, Australia, Europe, West Africa, and on the Atlantic coasts of both North and South America. Nypa is not known to have survived outside the tropical Indo-Pacific Ocean region after the Oligocene. Eocene pollen of Nypa and other mangroves, including Avicennia, Rhizophora, and Sonneratia, and of the beach tree, Barringtonia, has been found in southwestern Australia, which was situated south of 50°S at that time; this is a much higher latitude than is reached by any modern mangroves. Rhizophora was also present in the Paleogene of Borneo and the Caribbean. Likewise, there are widely scattered Eocene fossils attributed to various modern genera of pantropical beach plants, such as Caesalpinia in Nigeria and South America.
Transoceanic dispersal appears unlikely for the forest conifers and broadleaf hardwoods often assigned to the Arcto-Tertiary geoflora, whose ranges around the northern hemisphere were discussed previously. Some of these broad ranges, for example, of Sequoiadendron, Magnolia, and Platanus, may date from the Cretaceous, but many of the genera are not known from before the Paleogene. Perhaps some of them crossed between eastern North America and Europe in the early Paleogene before the Atlantic barrier developed, while others crossed by the longer available Bering land bridge. The relative importance of these two routes is still highly speculative. There is no evidence that the Arcto-Tertiary geoflora originated in a limited region and time span and spread as a unit. Presumably it was assembled gradually by convergence of taxa migrating over differing pathways from a variety of geographic origins. It may eventually be possible to sort out their migrational histories, but this awaits more and better dated fossil records.
There is an intriguing possibility that the northward drift of the Indian subcontinent ferried some seed plant lineages from Gondwanaland to Asia. Some Indian Paleocene and Eocene fossil floras are known from coastal and freshwater sediments associated with Deccan Traps lava flows. Some of these fossils are attributed to Podocarpus, a genus that survives in India today. Living Indian podocarps, however, belong to sections of the genus unknown in Africa or Madagascar and believed to have immigrated to southern Asia via the East Indies from Australia in much later Neogene time. Also, several of the Paleogene Indian fossils were attributed to genera of sea-dispersed coastal plants for which no ferry would have been needed, such as Nypa, Cocos, Barringtonia, Sonneratia, and Terminalia . Some of the Deccan fossils, however, belong to taxa not adapted for ocean dispersal, including Musaceae, which have a wide disjunction between Africa, Madagascar, and Southeast Asia.
Deep sea drilling has brought up Paleogene fossils from now sunken Indian Ocean regions: Broken Ridge, then perhaps part of Australia, and Ninetyeast Ridge, then perhaps a chain of volcanic islands. The floras included Araucaria, Casuarina, Proteaceae, and other taxa then present in Australia. Early Eocene floras in Australia show that Araucaria, Podocarpus, Casuarina, Nothofagus, and many genera of Proteaceae grew as far south as 60°S paleolatitude. Axelrod (1984) discussed the problem of explaining the presence of evergreen trees in polar regions. In the Oligocene, Eucalyptus and Leptospermum appeared in the Australian fossil record. It is uncertain whether their progenitors were present but undetected in the old Gondwana flora or whether they immigrated from the tropical Indo-Pacific islands.
10
Discussion Of
Prehistoric
Migrations
The clarity with which plant migrations can be resolved from the fossil record decreases drastically with increasing time into the past.
In the Late Glacial and Holocene fossil record, plants are generally assignable to living genera, if not species. There are many gaps and uncertainties, but the record is good enough to establish the presence or absence of dominant plant taxa over substantial areas and time spans. In so doing, the record reveals that in postglacial time, plant species carried out amazingly rapid and highly individualistic migrations. This fact conflicts with conceptual models that visualize vegetational associations or even whole floras as migrating ponderously as units. Also, this evidence does not support the assumption that locations of Pleistocene refugia can be deduced from present plant distributions. What have sometimes been regarded as primeval forests, both temperate and tropical, were assembled particularly late in the Holocene, and their present patterns bear no resemblance to glacial ones.
The earlier Pleistocene and Neogene records still provide evidence of past distributions of the ancestors, or close relatives of the ancestors, of living species. But the lacunae are too great and the dating too imprecise to follow migrations continuously as they proceeded. It is only possible to note geographic changes within very loose temporal and spatial brackets. The Neogene record does show that various species then had gross ranges much different from those of their present descendants, partly because present mountain ranges did not exist.
Farther into the past, it becomes increasingly uncertain whether fossils represent ancestors of much changed modern taxa or lineages that became extinct. Also, the gaps in the record become enormous, and it is only possible to discern vague outlines of rise, spread, and fall of major lineages. It is intriguing to try to relate spread of plant taxa to changes in the geography of continents as postulated by plate tectonic models. In some cases, it appears that overland pathways may have been available at the right time to allow spread of taxa that are not known to be capable of long-range transoceanic dispersal, including most gymnosperms and many angiosperms, such as Proteaceae, Nothofagus , and many other hardwood tree genera. In some other cases, seaways appear to have been available at the right time for spread of mangroves and other coastal and riparian pioneers likely to have been capable of long-range water dispersal. There is, however, too little paleobotanical evidence on dispersal mechanisms to make such interpretations more than working hypotheses.
It would obviously be a mistake to try to classify seed plants in general into those that migrate by either land or water pathways. Various taxa that are not primarily coastal or riparian and that lack adaptations for sea dispersal have certainly accomplished transoceanic migrations. This has been deduced from their ancient presence on the Galapagos, Hawaiian, and other oceanic islands. It is also demonstrated by their late appearance in the fossil record, too late for intercontinental land routes. Three of the most cosmopolitan angiosperm families, grasses, composites, and legumes, entered the fossil record during the Paleogene on various continents that were not then in contact.
Raven and Axelrod (1974) noted that the presently pantropical legume genus Acacia had crossed water barriers from South America to the West Indies by the Oligocene. Acacia has not been found in Oligocene floras of Australia, although it was formerly believed that it had been present there before the breakup of Gondwanaland. Acacia probably immigrated after Australia was completely isolated and had drifted into lower latitudes. In spite of its relatively recent arrival, Acacia has radiated into about 600 endemic Australian species, which play a major role in the continent's vegetation. Raven and Axelrod (1974) also cited the kapok family, Bombacaceae, as another pantropical family that spread by transoceanic dispersal between former Gondwanaland continents. On the species level, a member of the Bombacaceae, the kapok tree, Ceiba pentandra , was cited by Baker (1965b ) as a probable example of a noncoastal species that crossed the Atlantic in relatively recent geologic time by flotation of pods from South America to west Africa.