2—
STRUCTURE, STATUS, AND TRENDS IN THE CONDITION OF CALIFORNIA RIPARIAN SYSTEMS
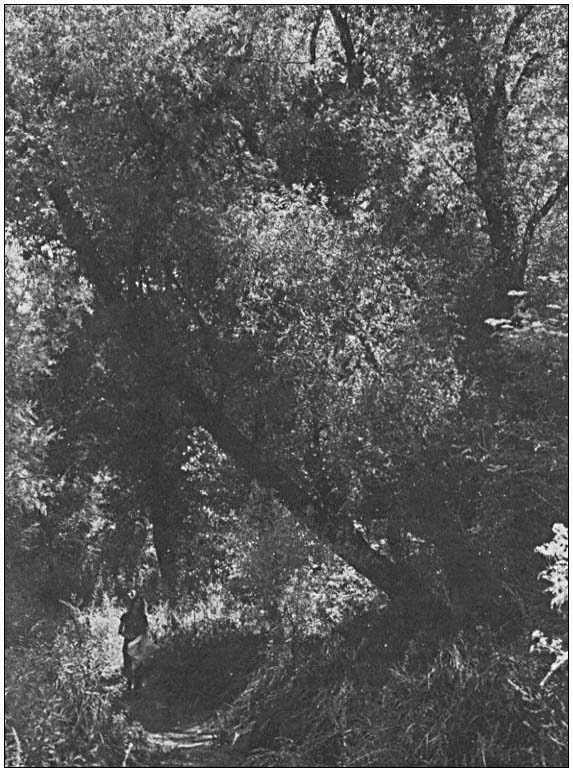
Summary of Riparian Vegetation Areal and Linear Extent Measurements from the Central Valley Riparian Mapping Project[1]
Edwin F. Katibah, Nicole E. Nedeff, and Kevin J. Dummer[2]
Abstract.—This paper summarizes the areal and linear extent measurements of riparian vegetation on the floor of the Central Valley of California, based on the maps produced by the Central Valley Riparian Mapping Project. Results are presented by riparian vegetation category for the applicable counties, and for the depositional bottomland or floor of the Central Valley as a whole.
Introduction
In 1978, the California Legislature, responding to the need for information on riparian resources in the state, appropriated $150,000 to the Department of Fish and Game (DFG) for a study of riparian resources in the Central Valley and California Desert (AB 3147, Fazio). A portion of this money was allocated for the mapping of riparian vegetation in the Central Valley.
In June, 1979, riparian mapping teams from the Geography Departments of California State University, Chico, and California State University, Fresno, completed mapping of the riparian vegetation on the floor of the Central Valley. The Department of Water Resources (DWR) made available 35mm. color slides of aerial photographs of those parts of the Central Valley (principally the irrigated and non-irrigated agricultural zones) for which it had photocoverage. Surrounding foothills and higher slopes were not included in the mapping project. The teams transferred riparian vegetation distributional data onto standard USDI Geological Survey (GS) 1:24,000 topographic quadrangle maps (quads). Riparian vegetation was mapped on fade-out blue copies or mylar overlays for each quad. A total of 465 individual map sheets, covering 388 unique quads, were compiled.
Area and Limits of Mapping Coverage
While the enabling legislation called for the study of Central Valley riparian resources up to the upper edge of the blue oak/digger pine zone (about 760 m. (2,500 ft.) elevation in the mountains surrounding the floor of the Central Valley) (Küchler 1977), only the depositional bottomlands of the Central Valley were mapped in this project. This was because the available DWR aerial photography was limited to those portions of the Central Valley where patterns of water use (principally agricultural) were being monitored.
Thus, the data on areal and linear extent reported here must not be construed either as the total amount of riparian vegetation for the entire Central Valley, which includes upland slopes as well as depositional bottomlands, or for the listed counties. As indicated in figure 1, only the depositional bottomland portions of the listed counties were mapped. As a result, summaries for Central Valley counties having only small amounts of depositional bottomlands (e.g., Nevada, Amador, Napa, Shasta) reflect only a small portion of these counties' total riparian resources.
Figure 1 indicates the actual mapping coverage by quad, within the relevant counties. Quads are identified by an index numbering system commonly used by California state agencies. A complete list of 388 individual quads, mapped for riparian vegetation, and the respective length and area measurements derived for each quad, are presented in the final report to DFG (Katibah, Nedeff, and Dummer 1980).
[1] Paper presented at the California Riparian Systems Conference. [University of California, Davis, September 17–19, 1981].
[2] Edwin F. Katibah is Associate Specialist, Remote Sensing Research Program, Department of Forestry and Resource Management, University of California, Berkeley. Nicole E. Nedeff is a Graduate Student, Department of Geography, and affiliated with the Remote Sensing Research Program, Department of Forestry and Resource Management, University of California, Berkeley. Kevin J. Dummer is Staff Research Associate, Remote Sensing Research Program, Department of Forestry and Resource Management, University of California, Berkeley.
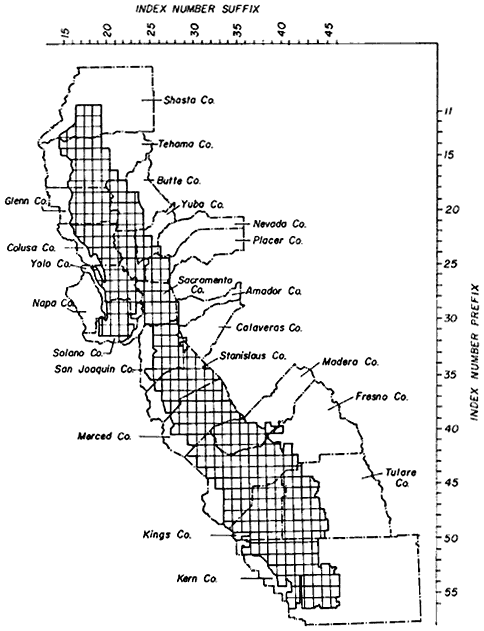
Figure l.
Locations of 1:24,000 quads in which riparian vegetation
was mapped. Quads only partially mapped are included.
Mapping Procedures
For the actual mapping of riparian vegetation, the teams used a physiognomic mapping category system, with vegetative life-form as the basic criterion: trees, shrubs, and herbaceous cover. These three basic lifeform categories were further refined by certain "modifiers", and by hybridizing the primary categories. Table 1 is a summary of the riparian mapping category codes developed by the mapping teams. The table does not include hybridized vegetation categories (i.e., R1/R2, R1/R3) developed and used by the teams. A more complete description of the riparian mapping project methodology may be found in Nelson and Nelson (1983).
|
Central Valley Riparian Vegetation
Table 2 is presented to give the reader some idea of the actual plant species found within Central Valley riparian systems. Typical riparian trees and shrubs are listed, along with an indication of their relationship to the mapping category codes used in the project.
|
Measurement Methods
Upon completion of the Central Valley Riparian Mapping Project, the DFG contracted with the Remote Sensing Research Program, Department of Forestry and Resource Management, University of California, Berkeley, to calculate the lengths and areas of each category of riparian vegetation by individual quad and by county.
Riparian vegetation on each overlay for each quad, comprising a network of polygons and linear features (with representative mapping category codes), was measured using a flat bed digititizer.[3] The digitizer has a resolution of 1,000 points per inch and 1,000,000 points per square inch. Areas and lengths of riparian vegetation were calculated in digitizing units and converted to acres and miles, as appropriate. At a map scale of 1:24,000, the digitizer conversion factors were:
lines:

areas:

Results
The riparian length and area results were tabulated by county and by quad overlay. An example of the county riparian vgetation tabulations is presented in figure 2. The complete set can be found in Katibah etal . (1980). In virtually all cases, the applicable counties did not have complete mapping coverage (see figure 1).
Table 3 gives an aggregated summary of the riparian vegetation mapping category measurements for the entire mapped portion of the Central Valley study area. Mapping categories were consolidated by combining all hybridized categories by their principal components (e.g., R1/R2 and R1/R3 would be included in the aggregated category R1 hybrid). Also included in the hybridized categories were categories where the modifiers "c", "d", and "i" were combined with a major riparian vegetation category (e.g., R3d would be included in R3 hybrid). The "miscellaneous" category refers to categories where the principal component in a hybridized category does not represent riparian vegetation (e.g., M/R3, where M designates marsh). Additionally, categories for valley oak are given under the codes R1v and R1v hybrid, even though this is a subcategory.
[3] Talos Series 6000 high resolution digitizer.
|
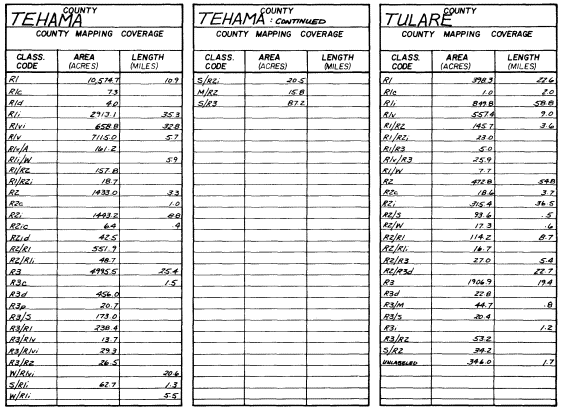
Figure 2.
Example of a county summary tabulation for
bottomland floodplain, riparian and aquatic wetlands.
Authors' Note
The results presented in this paper are based on a report submitted to the DFG (Katibah, Nedeff, and Dummer 1980). This report, containing more detailed information than is presented here, is filed with the original riparian vegetation maps (compiled by the Central Valley Riparian Mapping Project) at the California Natural Diversity Data Base, DFG, Sacramento.
Literature Cited
Central Valley Riparian Mapping Project. 1979. Interpretation and mapping systems. Report prepared by the Riparian Mapping Team, Geography Department, California State University, Chico, in cooperation with the Department of Geography, California State University, Fresno. 24 p. California Department of Fish and Game, Planning Branch. Unpublished manuscript.
Katibah, Edwin F., Nicole E. Nedeff, and Kevin J. Dummer. 1980. Areal and linear extent of riparian vegetation in the Central Valley of California. Final report to the California Department of Fish and Game, Planning Branch. Remote Sensing Research Program, Department of Forestry and Resource Management, University of California, Berkeley.
Küchler, A.W. 1977. Map of the natural vegetation of California. 1:1,000,000 + 31 p. A.W. Küchler. Department of Geography, University of Kansas, Lawrence.
Nelson, C.W., and J.R. Nelson. 1983. The Central Valley riparian mapping project. In : R.E. Warner and K.M. Hendrix (ed.). California Riparian Systems. [University of California, Davis, September 17–19, 1981]. University of California Press, Berkeley.
Roberts, W.G., J.G. Howe, and J. Major. 1977. A survey of riparian forest flora and fauna in California. p. 3–19. In : A. Sands (ed.). Riparian forests of California: their ecology and conservation. Institute of Ecology Pub. No. 15. 121 p. University of California, Davis.
An Historical Overview of the Sacramento River[1]
Lauren B. Scott and Sandra K. Marquiss[2]
Abstract.—This paper summarizes an analysis of two aspects of the history of the Sacramento River: the fluvial process; and man's development of the floodplain over the last 130 years. The analysis was made to trace the origins of problems—seepage, loss of riparian vegetation, and limited public access—occurring in the riparian zone, and to establish a perspective from which to study these problems. Significant historical aspects of these problems must be considered in a comprehensive study of the river.
Introduction
This paper presents an historical overview of the Sacramento River, shown in figure 1, to trace the origins of some of the problems occurring in its riparian zone, and to provide a perspective from which these problems can best be studied. The overview focuses on: 1) the fluvial process of the river itself, and 2) the principal activities of man over the last 130 years which have affected the river.
The Sacramento River has played a significant role in the history of the Central Valley and the State. The first humans occupying northern California chose to live along the banks of the Sacramento River as did later settlers who populated the floodplain, reclaiming the river's lands and diverting its waters. Today the river continues to provide a means of sustenance to the people of the Valley and the State.
Except for the lower reaches of the Mississippi River and certain reaches of the Columbia and Ohio Rivers, the floodwaters of the Sacramento River are greater than those of any other river in the United States. The river system, when combined with the runoff from the north and coastal areas, accounts for 70% of the State's total water production (US Army Corps of Engineers 1978). The water is used for irrigation, power, and municipal and industrial needs. The river itself is a navigation route, an increasingly important recreation resource, particularly near heavily urbanized areas, and a haven for numerous species of fish and wildlife which depend on its waters and the riparian system along its banks to survive.
Long before man came in contact with the river, natural processes that develop and shape rivers were creating the Sacramento River man would have to live with when he entered the Valley. The problems that we struggle with today have their roots in the early, pre-settlement development of the river.
Over the last 130 years, dams, dikes, levees, drainage works, bypasses and bank protection systems have been built to control the river and to protect people living in the floodplain. These facilities, often built years apart, to varying standards, and for different purposes, have greatly altered the river system. Built to solve a variety of problems, they created other problems. Among these problems are seepage of river water into adjacent agricultural lands, loss of riparian vegetation to urban and agricultural encroachment, and restriction of public access to the river. The many uses of the river have also resulted in diverse and sometimes conflicting views on how and by whom these problems should be resolved and how future development of the river should or should not proceed.
The overview presented here identifies significant historical aspects of these problems showing how the changes inherent in the fluvial process itself, together with the changes caused by man, have resulted in the river as we know it today.
Fluvial Morphology
Fluvial morphology can be defined as the science of the forms created by the action of flowing water (Lane 1955). The relationships among the many factors operating in this process are complicated and not completely understood. Two concepts from fluvial morphology—equilibrium and evolutionary development of rivers—have been chosen to show the importance of the fluvial process in understanding some of the problems of the Sacramento River.
[1] Paper presented at the California Riparian Systems Conference. [University of California, Davis, September 17–19, 1981].
[2] Lauren B. Scott is a Civil Engineer, and Sandra K. Marquiss is a Technical Writer-Editor, USDI Bureau of Reclamation, Sacramento, Calif.
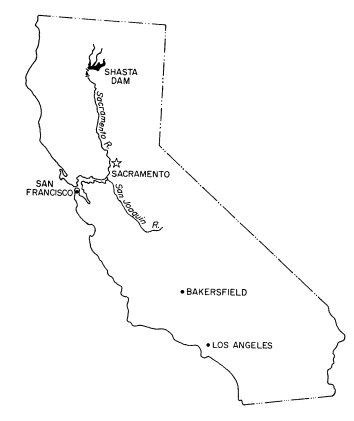
Figure l.
Location map.
Equilibrium
Alluvial rivers are among the most dynamic of all geomorphic forms. All changes in the river, however, whether occurring over geologic eons, or within a human lifetime, are governed by the principle of equilibrium; that is, although the river continually changes, only those changes leading to equilibrium, or stability, persist (Maddock 1976).
The principle of equilibrium is based on the assumption that all variables influencing the form of a river are interrelated in such a way as to represent a predictable system (Leopold and Maddock 1953). Since morphology and form of a river are primarily determined by the nature and quantity of sediment and water moving through the channel, the river's configuration is the result of a relationship among four variables: quantity of sediments, size of sediments, water discharge, and channel slope. The relationship among these variables is expressed as

A result of the dynamic properties of the fluvial process operating on a portion of the Sacramento River is illustrated in figure 2, which shows the configuration of the Sacramento River in 1874 and again in 1974. Some of the changes are notable: for example, the creation of a slough.
Evolutionary Development of Rivers
The variables of the fluvial process working to create a river valley have been characterized by some geomorphologists as an evolutionary process, a progression from youth to old age. There is no sharp division between the ages and no general agreement as to when one age ends and another begins (Johnson 1932).
By the time a river is old, as is the Sacramento River, the features of its valley are well developed and distinct. In the case of the Sacramento, floodplains are wide with low relief, and the river follows a broad, meandering course. Its channel is graded; that is, its energy and slope are just sufficient to carry away the material delivered to it from the uplands. Natural levees occur along its banks, with low-lying poorly drained swamp areas or flood basins on either side (Simmons and Senturk 1977). Two of these features—natural levees and meandering—are keys to not only understanding problems along the river but formulating their solutions.
Natural levees are the result of repeated overflows of sediment-laden river water onto adjacent lands, and occur where the valley slope is lowest and the duration of overbank flow is highest. The coarse, sandy material deposited close to the channel gradually builds up forming broad slopes which fall gently away from the river. Because they are comprised of coarse sediment, these levees are extremely porous and transmit water readily.
Natural levees along the Sacramento River occur discontinuously from Red Bluff downstream, and are most extensively developed in the river's middle reach from Ord Ferry to Sacramento. This is the same reach where the most extensive seepage problems occur. Here the river has adjusted to the lower slope of the Valley floor by annually overflowing its banks and emptying its water into the adjacent lateral flood basins. Near the city of Sacramento, levee heights range from 3.0–4.6 m. (10–15 ft.) above the adjacent low basin lands. Levee widths range from 3.2–4.8 km. (2–3 mi.).
Another important feature of the Sacramento River is its meandering. Meandering results from the constant and sometimes rapid changes in the form of a river and is the configuration taken by most alluvial rivers.
Meandering is thought to be caused by the direction of currents in the channel. These currents, in essence, cause a constant process of erosion of the riverbank and deposition of this

Figure 2.
Sacramento River, 1874 and 1974 (from Brice 1977).
material at a point farther downstream. The material deposited downstream accumulates as a point bar which builds out from the bank, constricting the channel and subsequently forcing the channel to move in a lateral direction. In such a way meander loops are thought to form and "move," or migrate down the valley (Simmons and Senturk 1977). The loops move unequally however, and may occasionally be cut off and abandoned as the river changes its course.
When acutoff occurs, the part of the river bypassed forms an oxbow lake, which gradually begins to fill in with sediment. The lower end of the oxbow, receiving the relatively impermeable finer silts and clays, eventually forms what is, in effect, a clay plug between the old meander loop and the main channel. Because of its impermeability this plug is essentially a semipermanent geologic control which can affect river geometry. Natural levees deposited from overflow and point bar, oxbow, and alluvial deposits laid down in meandering create complex soil structures along the river. It is through these soils that groundwater flows between the mountains and the river. Some deposits are very permeable and transmit water readily; others are impermeable, but influence the possible directions, horizontal and vertical, that water can travel.
A major factor in the fluvial process is the interrelation of all reaches of an alluvial river. Although specific changes in a river may originally be local, the effects of the changes can extend to all parts of the river (Burkham 1981). This characteristic is important when assessing the impact of man's activities on a river basin. Since the effects of a development on the river cannot be isolated to the reach in which it occurs, the net result of any change can be a greater departure, along the whole river, from equilibrium than that which was originally present.
Development of the Sacramento River by Man
Beginning with the discovery of gold at Coloma on the American River in 1848, man became another variable in the fluvial process. Principal activities of man affecting the river were urban settlement and agricultural development on the floodplain, and hydraulic mining of the surrounding foothills. The combined effect of these activities on the regime of the river was profound and far-reaching; the problemns of seepage, loss of riparian vegetation, and restricted public fishing access may be cited as consequences. The effects of hydraulic mining were so immediate and so drastic as to influence the course of all other development on the floodplain.
Hydraulic Mining
Hydraulic mining began in 1852 with the discovery that water under pressure could easily and economically remove the layers of lava and sediment covering the gold deposited in the ancient stream channels of the Sierra Nevada. Eventually, giant machines operated from considerable distances could tear apart a bank several hundred feet high in a very short time.
The machines that removed gold from the hills deposited millions of tons of silt and gravel in the nearby streams. Erosion that would have occurred naturally over hundreds of years occurred literally overnight. In one analysis of the amount of sediment in the rivers resulting from hydraulic mining, it was concluded that the 35 years of hydraulic mining tripled for about 100 years the average annual amount of sediment passed from the Sacramento Basin into San Francisco Bay under natural condititons. Over 1,000,000 acre-feet of debris has been deposited throughout the valley or passed into the Bay.[3]
The effect on the valley below was enormous. As river channels filled with more and more debris, the rivers rose. It is estimated that in some reaches the elevations of the Sacramento, Feather, Yuba, Bear, and American Rivers, the rivers most affected by sediment deposition, rose as much as 6 m. (20 ft.) (State of California 1978). With higher streambeds, capacities to carry water were greatly diminished. During a series of floods in 1861–62, 1875, and 1878, the debris washed out of the mountains and into the streams. The rivers overflowed their banks, inundating farms and homes with muddy polluted water.
By 1880, fertile land lost to hydraulic mining totaled more than 17,400 ha. (43,000 ac.).[4] The State Engineer, speaking to the State Legislature in 1880, described the effect of debris on farmland adjacent to the Yuba and Bear Rivers:
. . . the bottom lands were submerged . . . with sand and clay sediment, to such depths that in places orchards, gardens fields, and dwellings were buried from sight . . . and the course of the devastating flood was marked out by broad commons of slimes and sands.[3]
Antagonism between farmers and miners grew, culminating in a series of suits filed against the mining companies, in which the farmers at first sought damages, and eventually sought the complete abolition of hydraulic mining. In 1884, the State Supreme Court in the case of Woodruff v. North Bloomfield etal . prohibited the discharge of any mining debris into the streams. This decision, known as the Sawyer Decision, ended hydraulic mining.
The most adverse effects of hydraulic mining were on the rivers. Debris had choked and clogged some channels and completely obliterated others. With their equilibria destroyed, the rivers readjusted by overtopping their banks, depositing large quantities of debris in the valleys and carrying the remainder to San Francisco Bay.
Transport of the debris from hydraulic mining downstream took many years. Fluctuations in the low water levels, or streambed elevations, for the mouth of the Yuba River at Marysville and for the Sacramento River at Sacramento, shown in figure 3, indicate the length of time, depth of deposition of mining debris in riverbeds, and rate of erosion back to original stream elevations. The changes illustrated for the Yuba and Sacramento Rivers would occur in a similar manner in other streams in the Sacramento River system which experienced hydraulic mining.
Although the bed of the Sacramento River returned to its original elevation, the plan view of the river was permanently altered. Before the river established a new pattern of stability, dams and levees were built to control floods.
Development of the Floodplain
One of the most important impacts of hydraulic mining on the Valley came when mining was stopped. By the late 1800's development of the Valley's resources had come to an impasse. The interests of farming and mining appeared incompatible; one could continue only at the expense of the other. The Sawyer Decision in 1884, which ended the hydraulic mining era, signaled the beginning of the agricultural era, and also determined the course of future development of the Sacramento River. With the growth of agriculture and commerce as the Valley's principal economic activities, the Sacramento River system would be extensively developed for irrigation supplies and for flood control.
Urban settlement of the floodplain began with the Gold Rush and was concentrated around the city of Sacramento. The city's population of about 150 in 1848 exploded to 12,000 by 1852 (Sacramento Magazine 1976). With its favorable location at the juncture of the American and Sacramento Rivers, the city was an important port and supply center, linking the coast and the city of San Francisco with the goldfields. Upstream, the city of Marysville, also at the juncture of two rivers—the Feather and the Yuba—was another population center in the Valley.
In the decade following the Gold Rush many settlers turned to farming, and within a few years agriculture had become the principal use of land in the Valley. Agricultural development began on the natural levees, called rimlands
[3] Jones, G.H. January, 1967. Alteration of the regimen of the Sacramento River and tributary streams attributable to engineering activities during the past 116 years. Prepared for the Historical Records of Sacramento Sector, American Society of Civil Engineers.
[4] Hagwood, J.J. 1970. From North Bloomfield to North Fork: attempts to comply with the Sawyer Decision. Unpublished draft thesis for completion of Master of Arts degree, California State University, Sacramento.
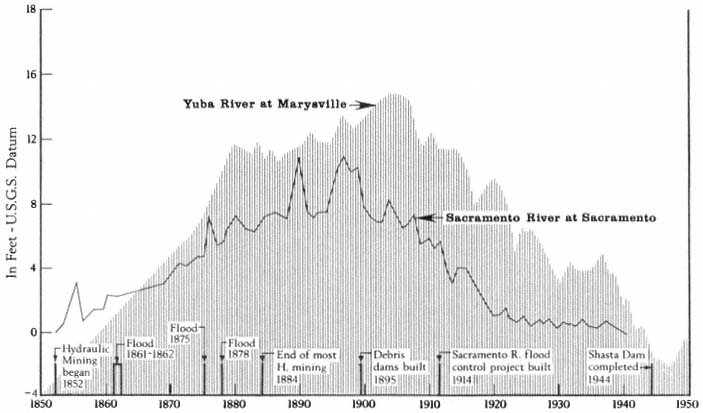
Figure 3.
Changes in bed elevation for portions of the Yuba and Sacramento Rivers, 1850–1950 (from G.H. Jones4 ).
because of their higher locations, which supported dense stands of riparian forests. It is estimated that in 1842, nearly 324,000 ha. (800,000 ac.) along the Sacramento River were forested, sometimes extending 8 km. (5 mi.) from the river.[5]
After the rimlands, the overflow, or tule. lands, comprising approximately 200,000 ha. (500,000 ac.) were developed (State of California 1976). Impetus for large-scale reclamation of the tule lands came in 1850 when the passage of the Arkansas Act transferred ownership of all such swamp and overflow lands from the Federal Government to the State on the condition that the lands be drained. The State in turn made these lands available for private ownership on the same condition of reclamation. This conditional transfer of ownership is significant because it determined that much of the land along the river would be used for agriculture and that ownership would be private, which has resulted in the problem of restricted public access to the river.
Levee Construction
As tule lands were reclaimed, the number of towns and farms increased, as did the need for flood protection. During very large storms the volume of water delivered to the river could be from four to eight times greater than the capacity of the channel, depending on the section of the river. The Sacramento River, particularly in its middle reaches over the flat valley floor, could not contain the volumes of water resulting from winter storms and spring runoff, and overflowed its banks almost annually. In the lower portion of the river the severity of these floods was amplified during the hydraulic mining period. For much of the year the floodplain was an inland sea, as vast quantities of water moved slowly down the valley through the flood basins to reenter the river in its lower reaches.[4]
At first, levees were constructd piecemeal by individuals or small groups with little or no consideration given to the effects on other areas along the river or the natural tendency of the river to meander. Natural drainage boundaries were ignored, with the result that some natural drains were closed off and marshes created in places which had previously been welldrained. Levee wars began as landowners on one side of the river raised their levees to force the floodwaters onto the opposite side of the river.
[5] Michny, F. 1980. Causes for the loss of riparian forest along the Sacramento River. Unpublished report. USDI Fish and Wildlife Service.
The configuration of the river changed rapidly and radically. Some levees eventually measured 7.6 m. (25 ft.) high and 61 m. (200 ft.) wide at the base. As levees were built higher, the water levels rose higher, and water that had previously overflowed into natural flood basins was now confined to a channel between the levees. As a result, during floods, the surface of the river water was often well above the level of the surrounding land.
Levee construction was accelerated when debris from hydraulic mining raised the riverbeds, decreasing the capacities of the river channels, including the Sacramento, which even under natural conditions could not contain its floodwaters. By the 1870's the beds of the Yuba and the Feather Rivers, tributaries to the Sacramento River, were higher than the town of Marysville, whose citizens responded by building better and even higher levees to protect the city. The city of Sacramento, inundated by a series of floods during the 1850's and 1860's, had by 1870 literally been raised by as much as 3.8 m. (12 ft.) to prevent future flooding of the city (Sacramento Magazine 1976).
Ultimately, efforts by individuals and small reclamation districts to prevent extensive flooding were ineffective. As the century closed, the complications from mining debris, a series of floods, and the inefficient and, in some cases, even detrimental levee system amply demonstrated the need for a Valley-wide flood control system.
Flood Control
The first centralized flood control plan was the Sacramento River Flood Control Project, formulated by the California Debris Commission. This project in essence rearranged the landscape to allow the river to revert to its natural regime during floods. The changes in regime enabled floodwaters overflowing into the adjacent flood basins to be conveyed slowly down the valley and returned back into the river in its lower reaches.
Authorized in 1914, the project was essentially in place in 1944 and is now about 90% complete. From the Sacramento River, water flows east into Butte Basin, thence to Sutter Bypass where it flows across the river into Yolo Basin, then through Yolo Bypass back into the river.
Foundation and composition of the man-made levees are part of the seepage problem. Man-made levees were built on top of the natural levees which were extremely porous. For economic reasons, the levees were often constructed from soils adjacent to or within the channel. These coarse, silty soils, which were deposited on the natural levees, were also extremely porous.
Levee construction and reclamation of levee lands destroyed large amounts of riparian vegetation and began the conversion of riparian lands to croplands which continues today. Levee systems often evolve into bank protection systems. This results in further loss of vegetation because the use of riprap and rock revetment to control erosion requires stripping the land of vegetation.
The Central Valley and State Water Projects
The Central Valley Project, begun in the 1940's, and the State Water Project, begun in 1960, were also constructed as part of the Valley-wide flood control system. Their purpose was in part to alleviate the imbalance in water supply between the northern and southern parts of the State. Both projects store and transfer water from the north to the central and southern parts of the State for irrigation and domestic use. The Sacramento River and its tributaries are the principal conveyors of this water to collection points in the Delta, where it is distributed south by a series of canals and holding reservoirs.
Key features of the Central Valley Project are Shasta Dam and Reservoir, which are operated for flood control and which modify the flow of the entire river downstream from the dam. Overall, this development has resulted in substantially higher summer flows, and intensified problems of erosion and sediment deposition. Because of the high flows, the streambanks never dry out and are more susceptible to erosion. Substantial amounts of sediment formerly deposited in flood basins are now deposited in the downstream overflow and bypass areas adjacent to the river and in the navigation and flood control channels.
Conclusions
This historical overview of the river points up two conditions as the basis for seepage, loss of riparian vegetation, and limited public access along the river. These conditions are: a) the location, composition, and foundation of flood control levees, resulting both from fluvial morphology and the activities of man; and b) the nature of land ownership and control along the river, laid out by the Arkansas Act.
Seepage problems were an inevitable consequence of agricultural development of levee lands and construction of man-made levees on top of the natural ones. Permeable complex soils deposited along the river are a major part of the problem. And although the impermeable deposits do not transmit water, they do influence the direction the seepage water can flow.
Construction of levees began and then accelerated the conversion of riparian lands to croplands. The riparian vegetation which remains today is essentially limited to thin strands along the river.
Land ownership patterns are directly related to both the loss and preservation of riparian vegetation, and directly affect the most popular recreational use of the river—fishing. Public access for recreation in general and fishing in particular is very limited and in some reaches almost nonexistent, a problem which will become more acute in the future.
A final value of an historical overview is the awareness of a continuing theme in the river's development—that of perspective. The levee building of the late 1800's was the result of piecemeal solutions to what was a Valley-wide problem of flooding. The broad and unified planning approach ultimately required to solve the flooding problem, is required again today to study the problems existing in the riparian zone. The fragmentary nature of land use and ownership patterns along the river has resulted in the creation of several publics, each competing to control some aspect of the river. Agreement on a common perspective of the river is difficult to achieve.
An overview suggests that an agreement on such a perspective could begin with the recognition that the river at any given time is the result of a continuing process among interrelated variables, of which the activities of man is but one. Planning from this point of view would seek to reinforce the natural tendency of the river toward equilibrium, and hence offer more satisfactory solutions to problems existing in the riparian zone.
Literature Cited
Brice, James. 1977. Lateral migration of the middle Sacramento River, California. USDI Geological Survey, Water Resources Investigations 77–43. Menlo Park, Calif.
Burkham, D.E. 1981. Uncertainties resulting from changes in river form. J. Hydraulics Division, Proceedings of American Society of Civil Engineers 107(HY5):593–610
California Department of Water Resources. 1955. Report to the Water Project Authority on seepage conditions in the Sacramento Valley.
California Resources Agency. 1978. Sacramento River Environmental Atlas. Prepared by the Upper Sacramento River Task Force for the California Resources Agency. Sacramento, Calif.
Johnson, Douglas. 1932. Streams and their significance. p. 78–96. In : S.A. Schumm (ed.). Benchmark papers in geology: river morphology. Dowden, Hutchinson, and Ross, Inc., Pennsylvania.
Lane, E.W. 1955. The importance of fluvial morphology in hydraulic engineering. p. 180–201. In : S.A. Schumm (ed.). Benchmark papers in geology: river morphology. Dowden, Hutchinson, and Ross, Inc., Pennsylvania.
Leopold, L.B., and T. Maddock, Jr. 1955. The hydraulic geometry of stream channels and some physiographic implications. USDI Geological Survey Professional Paper 252.
Maddock, T., Jr. 1976. A primer on floodplain dynamics and water conservation. J. Soil and Water Conservation 31(2):44–47.
Sacramento Magazine. 1976. Special Bicentennial issue. p. 21–25. July–August, 1976.
Simmons, D.B., and Fuat Senturk. 1977. Sediment transport technology. Water Resources Publication, Fort Collins, Colorado.
U.S. Army Corps of Engineers. 1978. Reconnaissance report on Sacramento River and tributaries bank protection and erosion control investigation, California. U.S. Army Corps of Engineers, Sacramento District, Sacramento, Calif.
Regeneration of Riparian Forests of the Central Valley[1]
Jan Strahan[2]
Abstract.—Riparian forests of the Sacramento River have an overstory and a regeneration pattern corresponding to the successional stage and fluvial landform associated with the forest stands. Cottonwood/willow forests form initially on gravelbars. With development of the floodplain and maturation of the forest, other species enter. Floodplain forest regeneration is primarily box elder, black walnut, and valley oak with few sycamore and ash. Riverside floodplain forests differ from oxbow lake forests in species diversity, density, and reproduction. Land use and water development projects alter fluvial landforms and fluvial events to create changes in forest composition and regeneration.
Introduction
Riparian systems provide an excellent opportunity to study the effects of landform and fluvial processes on vegetation distribution and forest regeneration. Erosion, deposition, and lateral channel migration regulate both the distribution and development of vegetation in the riparian zone. With continual changes in landforms as a result of seasonal and catastrophic fluvial events, vegetation dynamics remain in a state of "perpetual succession" (Campbell and Green 1968).
The generalized patterns of vegetation zonation resulting from fluvial processes have been described and illustrated by Conard etal . (1977) for the Sacramento Valley region. The Sacramento River Atlas (Upper Sacramento River Task Force 1978) illustrates the pattern of zonation as well as the successional stages found in riparian forests. McGill (1975, 1979) has also correlated the existing riparian vegetation with fluvial landforms. Gaines (1974) has noted that the more extensive remaining riparian forests occur on islands, along bends in the river, and adjacent to oxbow lakes and other areas subject to flooding. As such, the remaining forests are a result of the most dynamic interplay between the fluvial system and riparian vegetation.
Objectives
The primary objective of this study was to develop regeneration data for the dominant tree species in the riparian forests of the Sacramento River. The information compiled can be used to assess present conditions and future trends of the forests. Work by Conard etal . (1977) and Michny etal . (1975) illustrates the variety of plant community structure and composition encountered in the riparian zone. Recognizing this, information was gathered at two levels to gain a more comprehensive picture of the structure and composition than previously developed. A detailed quantitative study was undertaken at three sites near Princeton, Glenn County, where disturbance to the fluvial system and vegetation is relatively minimal. At these sites, regeneration was examined relative to landform in different successional stages: a young forest (less than 30 years) associated with a gravelbar; an established forest (less than 70 years) located on the floodplain along the current river channel; and a mature forest (greater than 85 years) adjacent to an oxbow lake. To understand the larger patterns occurring along the length of the river, a broad survey of the river as a fluvial system was undertaken. This survey relates the effects of land use and water resource development projects to regeneration potential and stand development. The survey included a review of the geomorphic and ecological literature as well as air and ground reconnaissance.
Methods
Floodplain vegetation was sampled using the point-centered quarter method of Cottam and Curtis (1956). Transects were located perpendicular to the water course at 50-m. intervals.
[1] Paper presented at the California Riparian Systems Conference. [University of California, Davis, September 17–19, 1981].
[2] Jan Strahan is a Graduate Student, Wildland Resource Science, Department of Forestry and Resource Management, University of California, Berkeley.
Points were centered at 10-m. intervals. At each point-center a 1-m. circular plot was used to tally the number of tree seedlings by species and a 10-m2 circular plot was used for saplings. Seedlings were defined as having become established this season, and saplings were classed as other size-classes less than 10-cm. diameter-at-breast-height (DBH). Saplings were further classified into seven size-classes: (1) less than 0.3-cm.; (2) 0.3- to 1-cm.; (3) 1- to 1.5-cm.; (4) 1.6- to 2.4-cm.; (5) 2.5- to 5-cm.; (6) 5- to 7.5-cm.; and (7) 7.5- to 10-cm. Vegetative reproduction was not distinguished from seed reproduction as part of this tabulation, but was recorded wherever observed. Composition and cover of the shrubs and groundcover were also recorded. On gravelbars, seedling establishment was sampled through the use of 1-m2 plots. Five-m. by 20-m. belt transects were used in the young forests on gravelbars and for levee sampling.
Physiography
Fluvial processes result in a number of characteristic landforms. Floods contribute to overbank deposition and aid in the building of floodplains. Lateral channel migration results in progressively building point bars which account for much of the existing natural topography of the Sacramento River riparian zone (Leopold 1973; Brice 1977). A cross-section through the riparian zone may have the following landforms: cut bank, point bar, natural levee, floodplain, oxbow lakes, meander scars, and islands. Variable surface features occur on these landforms, depending on the type of aggradation and frequency of flooding. The microtopography of the floodplain, consisting of ridges and swales, was formed by flows of old channels and is periodically altered by flood channel flows (Nanson and Beach 1977). These slight variations in elevation lead to considerable differences in soils and drainage conditions which provide the opportunity for tree species with different flood tolerances to occupy different elevations of the floodplains (Hosner and Minckler 1960). Vegetation, once established, also plays an active role in the depositional environment by acting as a sediment collector. Erosional bowls frequently form around trees and shrubs in the active channel.
Distinct landform changes occur in the downvalley progression of the Sacramento River. Brice (1977) describes the following features which change in the reach between Chico Landing and Colusa. As with most rivers, there is a downward progression in gravel size as one moves downvalley. The Sacramento River is classified as a gravel-bed stream from Red Bluff to Glenn. Below Glenn, it is a sand-bed stream. (Note: This shift was noted by Bryan in 1923, prior to the construction of Shasta Dam.)
Natural levees are composed of coarser materials deposited as floods flowed over the top of channel banks. Beginning at Hamilton City, the levees form a strip 4.8 to 8 km. (3 to 5 mi.) wide between Hamilton City and Colusa. Levees are discontinuous for several miles south of Stony Creek and continuous from near Butte City southward.
Brice (1977) also reviews the changes in the river which have occurred since white settlement, using the "natural" river of 1870 as a baseline. According to Brice, channel sinuosity has decreased while channel width has increased. Morphologic changes have been attributed to both clearing of riparian vegetation and the effect of levees in reducing overflow areas. These changes have caused the main river channel to be scoured deeper and wider and water velocities to increase. Meander loops from Butte City to Colusa are confined by artificial levees and tend to be distorted and unstable. Flow regulation by Shasta Dam has resulted in an increase in mean monthly flows at Red Bluff for June, July, and August from 6,190 ft3 /sec. (1889 to 1944) to 10,520 ft3 /sec. (1945 to 1970). Maximum observed flood peaks at Red Bluff before regulation attained about 250,000 ft3 /sec. with subsequent peaks of 140,000 ft3 /sec.
The California State Department of Water Resources (McGill 1979) identified 29,352 ha. of riparian zone from Butte Creek to Keswick Dam in 1977. This includes 3,828 ha. high terrace riparian vegetation (rarely flooded), 3,395 ha. low terrace (frequently flooded), 2,096 ha. gravelbars, 162 ha. oxbow lakes, and 3,942 ha. water surface, for a total of 13,423 ha. undeveloped lands. Agricultural lands comprise 14,852 ha. of the zone and 1,097 ha. are in other developed uses. Of particular significance in this study is the reduction of high terrace lands by 15% in the five years between 1972 and 1977, mostly through agricultural conversion. Erosional losses from bank undercutting are not concurrently offset by building processes.
These variations in physiography have major ecological significance in the riparian zone. Lindsey etal . (1961) attributed the different plant communities to the differences in soil-water relationships resulting from physiographic variation. The amount of floodplain activity and influence of the river on landforms results in different degrees of community stability. Wilson (1970) found stabilized forest communities developed along the Missouri River floodplain after the river had been stabilized by a series of dams and reservoirs. Campbell and Green (1968) link "perpetual succession" to rivers which actively meander over their floodplains. They found the frequent shifting of landforms and channels resulted in early successional stages occupying the majority of the floodplain. Everitt (1968) and Fonda (1974) attributed spatial distribution of the riparian plant communities primarily to the meandering pattern of the river.
Along the Sacramento River, physiographic variation was sampled through the use of transects perpendicular to the river. The three main
landform categories sampled were: gravelbar, floodplain adjacent to the riverside, and floodplain adjacent to oxbow lakes. These three categories are representative of a sequence of landform and soil development which led to progressively older forests with distance away from the channel.
Forest Establishment and Composition
Establishment and distribution of species in riparian forests is controlled by the interaction between fluvial events and ecological requirements of the species.
Fluvial Processes
The water regime of the river influences distribution through both seasonal fluctuations and catastrophic occurrences (Sigafoos 1964; Bell and Johnson 1974). Both the low-flow regime and high flows or floods causing inundation influence distribution. The low-flow regime, which provides freshly exposed surfaces, is the most important factor for successful seedling establishment and is critical for survival of young trees.
Whether the result of flooding is an adverse or beneficial effect on the plant is dependent on the frequency, duration, and depth of inundation (Teskey and Hinckley 1978). Susceptibility to flooding affects species location on the floodplain relative to the height of the water table. Tolerance to flooding may also vary between young and old trees of the same species (Lindsey etal . 1961). Inundation may result in the death of young or established plants through mechanical abrasion or through lack of sufficient soil oxygen. For established dormant plants, floods deposit soil nutrients necessary to maintain high productivity rates (Johnson etal . 1976). Time between major disturbances determines the amount of forest stands that will be in early, middle, or late successional stages throughout the floodplain. Both scour and fill processes, resulting from high flows, determine vegetation patterns: a flood may eliminate a portion of a mature forest through bank undercutting with the undercut material forming new depositional surfaces for seedling establishment further downstream. Aside from being the agent of plant mortality, flooding can also cause topping or "flood-training" (Sigafoos 1964) of both young and mature trees, resulting in the formation of sprout groups.
Ecological Characteristics
Ecological characteristics of the dominant tree species are important determinants of successional events in the riparian zone. Of particular importance are the light-weight seeds of the pioneer species dispersed by wind or water. Seed disperal at the time of a falling water level is essential for successful establishment of the pioneer species. These characteristics result in the initial colonization of a site by the pioneer species cottonwood (Populusfremontii ) and willow (Salix spp .). Shade intolerance of cottonwood and willow has been noted to be the limiting factor in preventing their establishment in mature forests as well as the need for a mineral seedbed for germination (Sigafoos 1964; Johnson etal . 1976; Lindsey etal . 1961). Mid-successional stages have species with both light-weight seeds (box elder, ash) and heavy seeds (black walnut, oak). However, they all are able to germinate through litter and under the canopy of a cottonwood/willow forest.
Results
The interaction of fluvial events, landforms, and autecological requirements has led to the development of heterogeneous forest stands along the Sacramento River. The following tree species were encountered frequently in the floodplain forests: box elder (Acer negundo ssp. californicum ), Fremont cottonwood (Populusfremontii ), willow (Salix spp., including S . hindsiana , S . laevigata , S . gooddingii , S . lasiandra , and S . lasiolepis ), and black walnut (Juglanshindsii ). Sycamore (Platanusracemosa ), valley oak (Quercuslobata ) and ash (Fraxinuslatifolia ) occurred less frequently.
Overstory composition associated with each landform in the intensive survey is shown in table 1. This mixed riparian forest (of the species listed above) is found in different successional stages along the majority of the floodplain. As shown by the table, early stages are usually pure cottonwood/willow. Mid- and late-successional stages frequently have a cottonwood/willow overstory and oak and sycamore ocasionally. Box elder, black walnut, and ash comprise the second canopy layer in these later stands. The broad survey also revealed different types of forest stands than encountered in the intensive survey of the river. There are several older groves of pure oak or oak/sycamore on high terraces. Within the later stages of the mixed forest, small stands of pure box elder or box elder/black walnut, approximately 200/m2 or less, were encountered in several locations. In the pure box elder stands average densities were 100 stems per 100 m2 . In these stands, saplings from 2.5 to 7.5 cm. were most frequent with no stems greater than 15 cm. found. Several standing dead stems and many small stems on the ground were evidence of an even higher density at one time. Shade was sufficient to prevent groundcover but a few Prunus sp . and valley oak seedlings were in the stand.
Regeneration
Establishment and survival of riparian species are related to landforms and a sequence of fluvial events (table 2). Most seedling establishment occurs along the newly exposed surfaces of gravelbars and is significantly
|
different in species composition than regeneration in the established forests.
Gravelbar Regeneration
Seasonal variation in flow regimes greatly influences establishment and survival of the pioneer species on gravelbars. During the winter, streamflows must remove humus and freshly fallen leaf litter from the surface so that the seeds land on mineral soil. A receding water level in late spring and early summer must coincide with cottonwood and willow seed dispersal. As establishment is directly related to the low-flow line (McBride and Strahan 1983), a 1-m. wide band of seedlings and saplings is often found along the river's edge. Prior to further flooding, seedlings must achieve sufficient size to withstand mechanical injury. The subsurface of bars must remain moist throughout the summer in order for the seedlings to withstand late summer drought. Late summer desiccation results in the death of many seedlings (McBride and Strahan ibid .). Winter floods often wash away or bury many seedlings. While density in the initial stages of establishment on bars is extremely high (table 3), the latter two factors account for significant mortality.
Floodplain Regeneration
Within the mature riparian forests of the floodplain, the link between regeneration and the flow regime of the river is not as direct. The most influential flows here are the floods which may remove seedlings established for a season or longer and at the same time prepare seedbeds. While low flows have less direct influence on these species than on those of pioneer species, McGill (1979) attributed some losses on high terraces of riparian vegetation to the lack of occasional flooding during the drought of 1976 and 1977.
In the mature forest, young cottonwoods and willows are rare while box elder and black walnut are common (table 2). The latter two species enter at a later successional stage, establishing through litter and under the shade of a cottonwood/willow canopy. While regeneration in the floodplain is currently occurring primarily in swales or on the banks of swales, young trees are much more scattered throughout the forest and much less dense (table 2), than on gravelbars. Some riverside forests 30–40 years old have little reproduction. Thus, distinct compositional differences exist between reproduction in the riverside floodplain forests and the oxbow lake forests with an increase in seedling density occurring in the oxbow lake forests. Factors limiting successful seedling establishment in the floodplain forests appear to be associated primarily with extremely dense groundcover. Grape vines were noted entwined around many dead saplings.
Succession
The successional progression of forest stands in the riparian zone begins with seedling establishment on gravelbars. The amount of available soil moisture may be an important factor governing these zonal sequences, with the younger land surfaces significantly drier than the older ones. Vegetation establishes on fresh surfaces of the point bar when sufficient sediment accumulates above summer low-water levels. Young cottonwood and willow stands do not form a continuous protective cover on the gravelbar because of the river cutting across point bars during floods. Providing floods do not alter the bar significantly, plant colonization creates additional deposits. Several inches of soil may be deposited by a single flood. As the bar builds higher, it is less frequently flooded. This deposition, in combination with channel migration, results in a stabilized floodplain developing from a shifting gravelbar.
If bars remain relatively undisturbed for a number of years, deposition gradually occurs until the floodplain supports mature cottonwood/willow forests. Eventually an understory of shade tolerant species enters the forests. Should the forests be missed by flood scouring
|
for many years, the cottonwood/willow may be replaced by these understory species. In places where the river has moved progressively across the floodplain in a uniform direction, a sequence of stand ages is produced, chronologically arranged in the direction of bend migration with the youngest stands nearest the river.
A broad perspective of Sacramento River successional stages is available through aerial reconnaissance. Bands of vegetation of successive ages can be found to occupy the floodplain (Murray etal . 1978). Channel lateral migration studies (Brice 1977) show the maximum ages of the forests in the intensive survey to be 32 years for the developing forest, 73 years for the riverside forest, and >85 years for the oxbow lake forest. Everitt (1968) noted similar findings for the Little Missouri River with germination and growth of cottonwood intricately related to the discharge of the river, movement of the channel, and development of the floodplain. Tree age increases both upvalley and away from the channel according to Everitt (ibid .) and is the result of the rise of sapling thickets along gravelbars.
Physiognomy
The forest structure and physiognomy differ considerably according to the age of forests and landforms on which they develop. Young cottonwood/willow forests are dense with many small trees, but have few other woody species. These gravelbar forests develop in progressive bands, each associated with a rise in elevation of the ridge-swale topography. In the older cottonwood/willow forests, the trees are tall and widely spaced, allowing sufficient light for shrub and herb development. Lianas are prominent in some stands and non-existent in others. Older forests have a two-layer tree canopy and are denser than
|
the mid-successional stage forests. Forests adjacent to cut banks are more frequently composed of alder, oak, or sycamore than are the forest edges that develop behind bars. Young oaks and sycamores were only found in mixed species stands, while old oaks and sycamores are found in groves without the associates. Diameter-classes (table 4) of the oxbow lake and riverside forests show the difference in species composition and structure of the two forests.
Reproductive Strategies
The most common method of reproduction is by seed. However, throughout the floodplain vegetative reproduction is also common. Sandbar willow (Salixhindsiana ) was frequently observed sprouting on higher portions of the gravelbars. This was explained by Wilson (1970) who noted an adaptive value of vegetative reproduction on sandy soils where seedling establishment is limited by surface soil moisture availability. Sprouting was also recorded on the floodplain in areas infrequently flooded: older sycamore trees frequently had basal sprouts. In areas which undergo severe mechanical abrasion from flows (banks downstream from reservoir flow releases or banks receiving a high degree of wave action from boats), vegetative reproduction was as common as seedling establishment.
Survival
In the developing cottonwood/willow forests, survival is reduced by both drought and winter flooding as well as shade and competition from groundcover. Significant attrition occurs for different stages of cottonwood development (compare tables 2 and 3). Floodplain forests had many dead trees, probably a result of the 1976–1977 drought (McGill 1979).
Discussion
Initial Establishment
The study indicates that the initial establishment of riparian forests is along point bars. Cottonwood and willow can be regarded as classic pioneer species; within this region, their seeds germinate almost exclusively on fully exposed alluvium recently deposited by the river. Not a single seedling of these species was found in any of the floodplain samples (table 3). This indicates that neither functions as a gap-phase species (Watt 1947) by establishing seedlings in forest openings following disturbance. Smaller stems of cottonwood/willow in the floodplain forests (table 4) appear to result from suppression or sprouting (Note: the two size-classes in table 3, plot 4 had all established at the same time). In areas with sufficient light, flood deposits of fresh alluvium may provide areas for a younger age class to develop. Other dominant tree species, such as box elder and black walnut, all have the ability to germinate and grow under the cottonwood/willow overstory. Without disturbance, they in time could replace the cottonwood/willow overstory.
|
Structure and Composition
The data also show that the structure and composition of the overstory are strongly related to stand age and horizontal and vertical position of the floodplain. For example, cottonwood and willow predominate in young stands on low terraces near the river. Ash, box elder, and black walnut enter cottonwood/willow stands over time and predominate in stands away from the river. Oak and sycamore are found in old stands on high terraces with the other dominants and along banks high above the river. Reproduction in these stands is very limited (table 5). Thus, species diversity initially increases as stands age, reaches a maximum in stands with mixtures of both pioneer and later successional species, and may decline slightly in oldest stands.
The high frequency of sapling box elder and black walnut in cottonwood/willow forests suggsts that the next successional stage will consist predominantly of these two species. However, although box elder was found in small pure patches, there is no evidence available at this time that large scale replacement of the cottonwood/willow type along the Sacramento River by these two species is occurring. Despite the establishment of cottonwood only on point bars, mature cottonwoods remain throughout the floodplain. Lateral channel migration occurs frequently enough to retain cottonwoods and willows in most stands except the few high terraces where only oak and sycamore remain.
Cultural Impacts on Regeneration
The Sacramento River riparian system is much altered both in its natural flow regime and floodplain characteristics. Land use and water resource development projects may have a significant effect on the current regeneration situation and on the future regeneration potential. While further research into these areas is necessary to provide quantitative data for the Sacramento River, correlation with other major rivers provides us with clues to changes caused by alteration of the riparian zone. Historical research, although qualitative, provides a picture of the riparian forests of the past upon which we may also draw.
|
Land Clearing
Removal of all but the frequently flooded areas of the riparian forests has had obvious impacts on the reduction of certain species such as oak and sycamore in the Sacramento Valley. Thompson (1961) cites several descriptions of the riparian forests prior to extensive clearing which speak of forests of oak, cottonwood, and sycamore. While the oak is found in large groves in several areas along the upper river, individual sycamores are scattered very infrequently throughout the forests. Ongoing reduction of the high terrace lands (McGill 1979) will contribute to a further reduction of these two magnificent species.
Introduced Species
The introduction of exotic species in the area has also changed species composition. For example, figs (Ficuscarica ) in patches in the forest create such a dense shade that reproduction under them is limited to sprouting figs. While these patches are fairly small in extent (100 m2 ), they have created a major change in the localities in which they are found by their high reproduction (table 2). Prune seedlings (Prunus sp.), and tree of heaven (Ailanthusaltissima ) are also found in many areas along the river.
The native black walnut (Juglanshindsii ), now so common in the riparian forests, appears to have become widespread in the forest through the use of its rootstock for commercial propagation of the English walnut (Juglansregia ). The only population noted along the Sacramento River prior to the arrival of European man was between Freeport and Rio Vista (Fuller 1978). This was discovered by Richard Brindsley Hinds of the Sulphur Expedition in 1837 (Thomsen 1963).
Grazing
Grazing of the forest may lower reproduction densities in floodplain areas. When grazed, forests are kept clear of groundcover and young trees. When grazing is excluded, the regrowth of a thick understory which may prevent seedlings from establishing has occurred in the riparian forests. Thus, grazing could be responsible for the lack of establishment of certain age-classes in the flood-induced age structure through seedling elimination. Further work is necessary to substantiate the degree to which this has affected the Central Valley riparian forests. Carothers (1977) had shown it to be a major cause of reduction in reproduction in the Southwest riparian forests.
Water Resource Development
Levees : Aerial photography of the river reveals a large-scale change resulting from the artificial levees. Above Colusa, artificial levees are either non-existent or are far away from the channel. This allows lateral migration to form point bars at most bends and provides new surfaces for cottonwood and willow establishment. Below Colusa, the levees are adjacent to the river channel preventing point bar formation. Aerial photography (Murray etal . 1978) depicts 18 bars forming in a 20-river-mile (RM) reach above Colusa and only four bars forming in a 20-RM reach below Colusa. Bars below Colusa are much smaller in size than those above Colusa. Without the initial landform on which to colonize, riparian forest formation and regeneration will not continue in the same pattern.
NewLandforms : Development of man-made levees has caused a disruption of gravelbar formation thereby limiting reproduction. However, the levees themselves could provide new habitats for the development of new forests, providing current management practices were discontinued. The following species were common on levees: alder, ash, fig, cottonwood, valley
oak, sandbar willow, and tree willow. Densities for saplings ranged from 3/100 m2 for most species up to 85/100m2 for willows and cottonwood <2.5 cm. stem diameter. Regeneration density was partially dependent on levee management. Survival in burned areas was mainly in swales with sandbar willow and a few sapling oaks near the top of the levee. Species zonation is very noticeable with oaks often lining the tops of the levees; alder, ash, and cottonwood near the water level; and willows in swales. Weirs also provide a place where seasonal water flows and abundant light have created an oak phase of riparian forests along their levees.
FlowRegulation : The impacts of controlled flows on seedling establishment and survival have two effects. On certain rivers, willow encroachment on the streambanks has occurred as a result of controlled flows (Pelzman 1973). Pelzman (ibid .) attributed this to a prolonged soil moisture which allowed greater establishment and survival. McGill (1979) and Brice (1977) have also noted an increase in vegetated bar surfaces for the Sacramento River. They both attributed this to the moderating effect of Shasta Dam which has resulted in the lack of scour. The data for seedling establishment for the Sacramento River as a controlled stream reveals a lower density of seedlings (table 3) than similar data collected for a non-controlled stream (McBride and Strahan 1983). This suggests that the annual falling of the water level that coincided with seed dispersal and allowed abundant germination on the non-controlled stream did not occur on the Sacramento River. Daily flow data (USDI Geological Survey 1978, 1979; 1980 and 1981 data not available) for the Butte City gauging station reveal a wide fluctuation of streamflow with high flows following low flows frequently during the months of May-September. Thus, the absence of a continual lowering of the water level could have resulted in a limited amount of seedling establishment this year. However, the controlled flows may result in a higher survival percentage through lack of scouring. Also, a continual provision of moisture throughout the summer would reduce losses from desiccation for those seedlings which do become established.
LandandWaterEffects : Forest composition for the entire Sacramento River riparian zone must differ from the earlier forests because only frequently flooded areas remain to be sampled. Thus our results probably show a more flood-tolerant community dominating the area than we once had. With the decrease in bank stability of the river (Brice 1877), bank erosion has caused the loss of high terrace lands resulting in further decrease of sycamore and oak forests. Infrequent flooding and higher stands due to controlled flows and levees has probably resulted in the development of a greater proportion of older trees, since flooding of the areas does not clear out the undergrowth and provide bare areas for establishment to occur. As the rate of meandering is a major factor in determining the proportion of the floodplain in pioneer, transitional, and later successional stages, changes in meandering noted by Brice (1977) would suggest different proportions of forest stands in these stages may occur in the future than we had in the past.
Summary
Existing riparian forests have been shown to have an overstory and regeneration that corresponds to landforms and fluvial processes as well as successional stages. Establishment, growth, maturation, and death of floodplain trees are merged with the complete flow regime of the river and the erosion and deposition of sediment. The heterogeneity of forests is an indicator of a dynamic fluvial system. Establishment of the forests begins on gravelbars with the development of a cottonwood/willow type, making bars a critical landform in forest development. With deposition and time, the forests develop and mature, with understory species of box elder and black walnut becoming frequent. While regeneration on the bars is almost totally cottonwood and willow, regeneration on the floodplain is predominantly box elder and black walnut, especially on the low terraces. High terraces have minor amounts of oak, sycamore, and ash establishing. Forests surrounding oxbow lakes are older and have higher densities of reproduction than riverside floodplain forests. Water resource development projects and land uses have significant impacts on regeneration potential of riparian forests.
Literature Cited
Bell, D.T., and F.L. Johnson. 1974. Floodcaused mortality around Illinois reservoirs. Trans. Ill. State Acad. Sci. 67(1):28–37.
Brice, James. 1977. Lateral migration of the Middle Sacramento River, California. USDI Geological Survey Water-Res. Investigations 77–43. 51 p.
Bryan, Kirk. 1923. Geology and ground-water resources of Sacramento Valley, California. USDI Geological Survey Water-Supply Paper 495. 285 p.
Campbell, C.J., and Win Green. 1968. Perpetual succession of stream-channel vegetation in a semiarid region. J. Ariz. Acad. Sci. 5:86–98.
Carothers, S.W. 1977. Importance, preservation, and management of riparian habitat: an overview. p. 2–4. In : R.R. Johnson and D.A. Jones (tech. coord.). Importance, preservation, and management of riparian habitat. USDA Forest Service General Technical Report RM-43. Fort Collins, Colo.
Conard, S.G., R.L. MacDonald, and R.F. Holland. 1977. Riparian vegetation and flora of the Sacramento Valley. In : A. Sands (ed.). Riparian forests in California: their ecology and conservation. Institute of Ecology Pub. 15. 122 p. University of California, Davis.
Cottam, Grant, and J.T. Curtis. 1956. The use of distance measures in phytosociological sampling. Ecology 37(3):451–460.
Dietz, R.A. 1952. The evolution of a gravel bar. Missouri Bot. Garden Annals 39:249–254.
Everitt, B.L. 1968. Use of the cottonwood in an investigation of the recent history of a flood plain. American J. of Sci. 266:417–439.
Fonda, R.W. 1974. Forest succession in relation to river terrace development in Olympic National Park, Washington. Ecology 55: 927–942.
Fuller, T.C. 1978. Juglans hindsii Jepson ex. R.E. Smith. Northern California black walnut. Rare plant status report. California Native Plant Society.
Gaines, D. 1974. Review of the status of the Yellow-billed Cuckoo in California: Sacramento Valley populations. Condor 76:204–209.
Hosner, J.F., and L.S. Minckler. 1960. Bottom-land hardwood forests of southern Illinois—regeneration and succession. Ecology 44(1):29–41.
Johnson, W.C., R.L. Burgess, and W.R. Keammerer. 1976. Forest overstory vegetation and environment on the Missouri River floodplain in North Dakota. Ecol. Mono. 46:59–84.
Leopold, L.B. 1973. River channel change with time: an example. Geol. Soc. Amer. Bull. 84(6):1845–1860.
Lindsey, A.A., R.O. Petty, D.K. Sterling, and W. Van Asdall. 1961. Vegetation and environment along the Wabash and Tippecanoe Rivers. Ecol. Mono. 31(2):105–156.
McBride, J.R., and Jan Strahan. 1983. Influence of fluvial processes on patterns of woodland succession along Dry Creek, Sonoma County, California. In : R.E. Warner and K.M. Hendrix (ed.). California Riparian Systems. [University of California, Davis, September 17–19, 1981.] University of California Press, Berkeley.
McGill, R.R., Jr. 1975. Land use changes in the Sacramento River riparian zone, Redding to Colusa. California Dept. of Water Resources, Northern Dist. Report. 23 p.
McGill, R.R., Jr. 1979. Land use change in the Sacramento River riparian zone, Redding to Colusa. An update—1972 to 1977. 34 p. California Department of of Water Resources, Sacramento.
Michny, F.J., D. Boos, and F. Wernette. 1975. Riparian habitat and avian densities along the Sacramento River. California Dept. of Fish and Game Adm. Report 75–1.
Murray, Burns, and Kienlen. 1978. Retention of riparian vegetation. Sacramento River, Tisdale Weir to Hamilton City. California Dept. of Water Resources, The Reclamation Board.
Nanson, G.C., and H.F. Beach. 1977. Forest succession and sedimentation on a meandering-river floodplain, northeast British Columbia, Canada. J. Biogeog. 4:229–251.
Pelzman, R.J. 1973. Causes and possible prevention of riparian plant encroachment on anadromous fish habitat. California Dept. of Fish and Game. Adm. Report 73–1.
Sigafoos, R.S. 1964. Botanical evidence of floods and floodplain deposition. USDI Geological Survey Prof. Paper 485-A. 35 p.
Sudworth, G.B. 1908. Forests trees of the Pacific slope. USDA Forest Service. 441 p.
Teskey, R.O., and T.M. Hinckley. 1978. Impact of water level changes on woody riparian and wetland communities. Vol. 1: Plant and soil responses to flooding. USDI Fish and Wildlife Service. 30 p.
Thompson, Kenneth. 1961. Riparian forests of the Sacramento Valley, California. Ann. Assoc. Amer. Geog. 51(3):294–315.
Thomsen, H.H. 1963. Juglans hindsii . The Central California black walnut, native or introduced? Madrono 19(1):1–10.
USDI Geological Survey, 1978, 1979. Water resources data for California. Vol. 4: Northern Central Valley basins and the Great Basin from Honey Lake to Oregon state line.
Upper Sacramento River Task Force. 1978. Sacramento River environmental atlas. California Dept. of Water Resources.
Watt, A.S. 1947. Pattern and process in the plant community. J. Ecol. 35(1&2):1–22.
Wilson, R.E. 1970. Succession in stands of Populusdeltoides along the Missouri River in southeastern South Dakota. Amer. Midl. Nat. 83(2):330–342.
Plant Succession on Merced River Dredge Spoils[1]
Thomas H. Whitlow and Conrad J. Bahre[2]
Abstract.—One hundred and nine species of vascular plants were collected from 22 stands at six sites in the 2,800 ha. (7,000 ac.) of dredge spoils along the Merced River near Snelling, California. Five sites were dredged, one per year, in 1910, 1928, 1938, 1941, and 1950; one was not dredged. Association analysis of the stand data identified four species groups closely related to dredge-spoil topography and moisture availability. In addition, the program ordinated stand data according to floristic affinities. The ordination showed no age-dependent patterns.
Introduction
Nearly 24,000 ha. (60,000 ac.) of floodplains and terraces in the northeastern part of California's Central Valley were mined by huge gold dredges from 1898 to 1968 (fig. 1). In all, 12 major gold fields were dredged between Butte Creek and the Merced River (Clark 1970; Wagner 1970). The dredge spoils consist of wormlike ridges of unsorted boulders and cobbles with intervening swales of fine-textured soils and standing water (fig. 2). Except for a few areas of limited extent leveled for housing or used as a source of aggregate, the spoils now serve as little more than poor grazing lands and wildlife habitat. Nevertheless, they offer plant ecologists a novel means of studying plant succession because the spoils are of similar structure and can be dated to the week of deposition as far back as 70 years. Summarized here is a preliminary investigation of successional patterns in the Snelling dredge field (fig. 3).
The Snelling dredge field, mined between 1907 and 1951 (Aubury 1910; Davis and Carlson 1952; Clark 1970), consists of 2,800 ha. (7,000 ac.) of spoils paralleling the Merced River near the town of Snelling (fig. 4). Snelling (79 m. [259 ft.] above sea level) has an average annual precipitation of 840 mm. (33.2 in.), most of which falls between November and April (California Department of Water Resources 1980). Local vegetation is valley oak woodland (sensu Griffin 1977), grassland (sensu Heady 1977),

Figure l.
Yuba Dredge No. 2. This dredge, once owned by Yuba
Goldfields, was brought to Snelling from Montana in 1935.
Only 23 m. (75 ft.) long, it was one of the smallest dredges
ever used at Snelling. It was dismantled in 1939 and
taken to Chico.
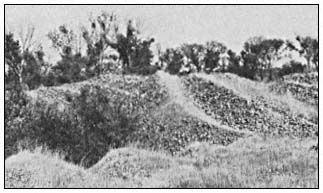
Figure 2.
Dredge spoil mound tops. Note cobbles and boulders
as well as sparse vegetation cover. Trees in the swales
are Salix spp. and Populus fremontii . and riparian forest
(sensu Conard et al . 1977).
[1] Paper presented at the California Riparian Systems Conference. [University of California, Davis, September 17–19, 1981].
[2] Thomas H. Whitlow is Research Associate, Urban Horticulture Institute, Cornell University, Ithaca, N.Y. Conrad J. Bahre is Assistant Professor, Department of Geography, University of California, Davis.

Figure 3.
Map of east-central California. Note the Snelling field.
The gold is mostly flour gold derived from the pocket belt of the Mother Lode in Mariposa Country, which is traversed by the upper course of the Merced River. It is found in the Quaternary gravel deposits of the modern river which range in depth from 5.4 to 11 m. (18 to 36 ft.)
Bucket-line gold dredges, constructed chiefly of steel, were so heavy and cumbersome they had to be assembled in a dry pit. Water from a canal was turned into the pit, allowing the dredge to float (Aubruy 1910). The dredges were only capable of digging 7.6 to 9.1 m. (25 to 30 ft.) below the surface of the dredge pond. Their earth-moving capacity, depending on their size, ranged from 153,000 to 260,000 cu. m. (200,000 to 340,000 cu. yd.) per month.
Individual dredge piles usually differ in slope and elevation. Fines may or may not be found on the surfaces. Whereas some dredge piles are in neat rows. most are rather haphazard. Differences in spoil topography reflect dredging depths, the particulars ways in which the tailing
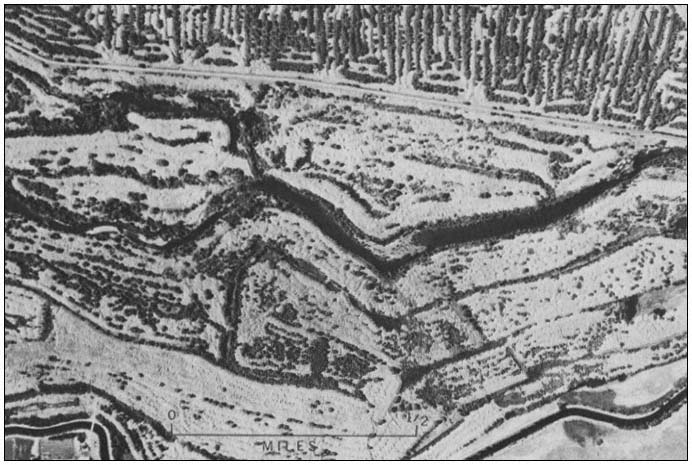
Figure 4.
Vertical aerial photograph of part of the Snelling dredge field. Note tree-lined swales and sparsely
vegetated spoil tops. The oldest spoils do not necessarily have tree-lined swales. In some cases, the
spoils were stacked so that no swales were left for trees to colonize (photograph taken in 1959).
stackers were operated, and whether overburden was sent directly to the stacker without sorting.
Methodology
Five study-sites in the Snelling dredge field were selected and dated using USDI Geological Survey 1:24,000 topographic maps, large-scale aerial photographs, mining progress maps, interviews with local residents, and records in the Merced County Recorder's Office (table 1). These sites were dredged, one per year, in 1910, 1928, 1938, 1941, and 1950. One undredged site was selected as a control.
Stands on mound tops, high swales, and low swales at each dredge site were subjectively chosen to conduct a complete floristic inventory. This inventory was needed to detect floristic distinctions between sites of different ages. The sharp gradients and irregular depositional patterns of the spoils made random and systematic sampling techniques impractical and highly sensitive to artifacts of sample location. Edge effects were minimized by placing the stands no closer than 100 m. (300 ft.) from the edges of the spoils. The stands, which varied in size and shape, were inventoried using the Braun-Blanquet relevé method (Mueller-Dombois and Ellenberg 1974). High swales were inventoried intoto , while mound tops and low swales were inventoried along belt transects whose lengths were designed to include all species in a particular habitat. The sampling method was designed to eliminate minimum area problems arising from the use of discrete sample sizes, to represent the total flora, and to establish within-stand homogeneity while maximizing the possibility of between-stand differences.
The stand data were analyzed using the association analysis program of Ceska and Roemer (1971). This program, which identifies associational relationships between species using pre-established phyto-sociological criteria, arranges species into groups of co-occurrence and ordinates stands according to floristic similarities. Of the program's five different "inside-outside" rules, the 50%-inside: 20%-outside rule was chosen because it included the highest percentage of the entire flora and hence accounted for more variations than the other rules. According to this rule, a species belongs to a species group if it occurs in 50% of the stands in its group and does not occur in more than 20% of the stands outside of its group. A stand belongs to a species group when it contains 50% of the species in the group. Field work was carried out between March and May 1980, during the peak bloom of the annual flora.
Results and Discussion
One hundred and nine species of vascular plants were collected from 22 stands at the six study-sites in the dredge field. The association
|
analysis program organized the stand data into four species groups (table 2). Only 36 of the 109 species were sufficiently well associated to be included in these groups. From left to right in table 2, the stands range from dry mound tops with sparse annual grass and herbaceous cover, to high dry swales dominated by thickets of Salix spp., to low mesic swales with forests of Salix spp. and Populusfremontii surrounding
|
shallow ponds. The four high-swale stands at the far right of the table do not fit within the topographic-moisture gradient of the rest of the ordination or within any single species group. This fact, plus their affinities to Groups 1, 2, and 4, are due to small-scale habitat variation. Stand 2, a high swale covered by grass, also falls within the xeric mount tops, whereas Stand 10, another high swale, contains two species groups.
The nesting of the species groups according to moisture availability and topography is readily apparent in table 2. Group 1, composed primarily of introduced annual species, grows exclusively on mound tops and corresponds floristically and physiognomically to the "California annual type", a climax annual grassland described by Heady (1956, 1958, 1977). Heady's climax annual grassland depends on the development of organic mulch; here it has developed on largely unweathered dredge spoil and completely lacks a litter layer.
Group 2, made up entirely of native tree species, occupies the bottom of nearly every swale with fine-textured soils. It overlaps little with Group 1; only Stand 10 includes extensive representation from both groups. Group 2 species are common pioneers in disturbed riparian zones in the Central Valley, and except for Quercuslobata all have wind-dispersed seeds (Munz and Keck 1959; Thompson 1961; Conard et al . 1977). Except for two large individuals at the 1910 site, the oaks are seedlings growing in the shade of willows. Since no parent oaks grow nearby, the oaks probably germinated from acorns dispersed by animals.
Group 3, consisting of woody perennials and emergent aquatics, occupies the edges of forested sites and shares most of the low mesic swales with Group 2. Group 3 reflects the greater habitat diversity of the low swales because of the presence of perennial ponds and a forest canopy. Vitiscalifornica and Rubusprocerus occupy the landward margins, whereas Typhalatifolia and Juncuseffusus occupy the pond margins.
Group 4 is made up largely of herbaceous annuals growing in semi-shaded, moist places in the swales containing standing water. The species in this group range from emergent and floating aquatics (Cyperusalternifolius , Alisma sp., and Marsileavestita ) to plants usually found in open, droughty habitats (Centaureamelitensis , Brizaminor , and Cynodondactylon ). Group 4, like Group 3, is also associated with a forest canopy.
Sixty-two percent of the species collected in the dredge field are native, 38% are introduced. According to Heady (1977), the percentage of native plants in species lists for individual stands in California's annual grassland ranges between 71% at Hastings Reservation (White 1967) and slightly less than 20% at Hopland (Heady 1956). Our findings indicate that the Snelling dredge field has a relatively high proportion of native species.
In addition to identifying associational groups, the Ceska and Roemer program ordinated the stand data according to floristic affinities (table 2). However, the ordination shows no age-dependent patterns. In some cases, closer affinities occur between stands of the oldest and youngest sites than between stands of the same age.
Seventy-three species were not included in any of the four species groups because they were too frequent (>66% is the threshold value of the program) or non-faithful. Although we lack the quantitative data to fully interpret the significance of these rare species, two conclusions are drawn. First, our sampling was adequate because it included so many rare species. Large stands usually include a high proportion of rare species (Preston 1948). Secondly, rare species are continually being added to the flora for at least 50 years after spoil deposition.
Table 3 summarizes the percentage of rare species in proportion to the total flora of the six study sites. Vicia benghalensis was excluded because its occurence exceeded the 66% threshold of the program. The other species had frequency values of 18% or less. Note that the rare species made up 55% or more of the total species in the undredged and 1910 sites, and only 30–39% of the species in the other sites. A similar trend has been documented by Bazzaz (1975) in his study of old field succession in Illinois. There, he found that species diversity increased most rapidly during the first 15 years after field abandonment and that the species colonization curve maintained a positive slope for at least 40 years. Since the youngest spoils at Snelling are 30 years old, we have probably missed the rapid colonization phase that occurred shortly after dredging. White (1966) in his studies of abandoned fields at Hastings Reservation noted that only 18 to 28 years were needed after initial disturbance for the dominant species of the climax grassland to re-establish themselves.
Changes in species diversity were not quantifiable because the stands were of different sizes. Nevertheless, the 1910 and 1928 sites were floristically richer than the other sites (table 3). Of particular interest is the fact that Chlorogalum grandiflorum , Brodiaeacalifornica , and B . multiflora , all native perennials, were only found at the 1910 site. The occurrence of these perennials and greater species diversity at this site may not be entirely age-dependent. The site, which is periodically flooded by the Merced River, has some alluvium and is exposed to flood-borne propagules. The undredged grassland site contained most of the Group 1 species plus three native perennials: Stipapulchra , Brodiaeahyacinthina , and Calochortus luteus . In general, species
|
diversity was highest in the forests of the mesic swales and lowest in the grasslands of the mound tops.
Conclusions
Only small changes in species composition were noted in the dredge-spoil sequence investigated at Snelling. The dominant species probably colonized rapidly after spoil deposition. The four species groups identified by the Ceska and Roemer (1971) program occur on all sites regardless of age. The groups correlate most closely with dredge-spoil topography and moisture availability. In general, the slow weathering of the dredge spoil has not resulted in enough soil development to affect the vegetation. Only in a few swales with moist, shallow soils and standing water is the vegetation very diverse or structurally complex. Several immature Quercuslobata in the swales and a Quercus wislizenii at the 1910 site are the only evidence of direct species replacement in the study-sites. The data suggest that species richness increases with successional age and that 50 years or more are required for the accumulation of well-developed flora. Structural changes will be much slower, correlating with the slow development of soil.
Acknowledgments
Financial support was provided by a Faculty Research Grant from the University of California, Davis. We thank the following residents of Snelling for their assistance and information: Robert Peirce, H.G. Kelsey, Ed Romero, and Kermit Robinson. Thanks must be extended also to Jack Major and Marlyn Shelton who read and commented on the manuscript.
Literature Cited
Aubury, L.E. 1910. Gold dredging in California. California State Mining Bureau, Bull. 57. 312 p. California State Printing Office, Sacramento, Calif.
Bazzaz, F.A. 1975. Plant species in old-field successional ecosystems in southern Illinois. Ecology 56:485–488.
California Department of Water Resources. 1980. California rainfall summary: monthly total precipitation 1849–1979. Various pages. California Department of Water Resources, Sacramento, Calif.
Ceska, A., and H. Roemer. 1971. A computer program for identifying species-relevé groups in vegetation studies. Vegetatio 23:255–277.
Clark, W.B. 1970. Gold districts of California. 180 p. California Division of Mines and Geology, San Francisco, Calif.
Conard, S.G., R.L. MacDonald, and R.F. Holland. 1977. Riparian vegetation and flora of the Sacramento Valley. p. 47–55. In : A. Sands (ed.). Riparian forests in California: their ecology and conservation. Institute of Ecology Pub. 15, University of California, Davis. 122 p.
Davis, F.F., and D.W. Carlson. 1952. Mines and mineral resources of Merced County. Calif. J. Mines Geol. 48:207–251.
Griffin, J.R. 1977. Oak woodland. p. 383–415. In : M.G. Barbour and J. Major (ed.). Terrestrial vegetation of California. 1002 p. John Wiley and Sons, New York, N.Y.
Heady, H.F. 1956. Changes in a California annual plant community induced by manipulation of natural mulch. Ecology 37:798–812.
Heady, H.F. 1958. Vegetational changes in the California annual type. Ecology 39:402–416.
Heady, H.F. 1977. Valley grassland. p. 491–514. In : M.G. Barbour and J. Major (ed.). Terrestrial vegetation of California. 1002 p. John Wiley and Sons, New York, N.Y.
Mueller-Dombois, D., and H. Ellenberg. 1974. Aims and methods of vegetation ecology. 547 p. John Wiley and Sons, New York, N.Y.
Munz, P.A., and D.D. Keck. 1959. A California flora. 1689 p. University of California Press, Berkeley, Calif.
Preston, F.W. 1948. The commonness, and rarity of species. Ecology 29:254–283.
Thompson, K. 1961. The riparian forests of the upper Sacramento Valley. Ann. Assoc. Am. Geogr. 51:294–315.
Wagner, J.R. 1970. Gold mines of California. 259 p. Howell-North Books, Berkeley, Calif.
White, K.L. 1966. Old-field succession on Hastings Reservation, California. Ecology 47:865–868.
White, K.L. 1967. Native bunchgrass (Stipapulchra ) on Hastings Reservation, California. Ecology 48:949–955.
Historical Vegetation Change in the Owens River Riparian Woodland[1]
Timothy S. Brothers[2]
Abstract.—This study evaluates human-caused vegetation change in the riparian woodland of Owens River (Inyo Co., Calif.). The greatest change has occurred below the intake of the Los Angeles Aqueduct, where drying of the channel has eliminated most native riparian cover and allowed invasion by salt cedar (Tamarixramosissima ) and Russian olive (Elaeagnusangustifolia ). Fire, water management, and other factors may have reduced tree cover above the aqueduct intake and encouraged proliferation of weedy native shrubs. The present scarcity of tree seedlings suggests that one or more of these factors continues to inhibit tree regeneration.
Introduction
Owens River has long been an important source of water for an otherwise arid region. Since settlement of Owens Valley by Europeans in the 1860's it has supported a mix of farming, mining, stock raising, and other economic activities common in the West. Its waters have been diverted for agriculture within Owens Valley and for export to Los Angeles. This study examines how these activities have changed the character and extent of the riparian woodland bordering Owens River.
Physical Setting
Owens River runs from just south of Mono Lake through Owens River Gorge to the head of Owens Valley, and from there the length of Owens Valley to Owens Lake. This study was limited to Owens Valley.
Below Owens River Gorge, Owens River is a meandering, low-gradient stream (fig. 1), winding over a floodplain whose width varies from less than 100 m. to more than 1 km. The floodplain lies at a lower elevation than the rest of the valley floor and is bounded by abrupt bluffs along much of the river. The floodplain surface is crisscrossed in many places by abandoned river meanders, but is otherwise fairly level. The river channel is relatively deep and narrow, bordered in many places by steep banks composed of fine-textured, cohesive alluvium.
The Owens River system is fed almost entirely by runoff from the Sierra Nevada, but even before man altered the hydrologic regime only about a third of its Sierra tributaries maintained perennial flow to the river. The rest normally disappeared short of the river channel as a result of percolation and evaporation. Much Sierra runoff thus reaches the river as subsurface flow. South of the Poverty Hills, however, the 1872 earthquake fault (fig. 1) restricts eastward groundwater movement (Los Angeles Department of Water and Power 1966). No groundwater reaches the river channel where it lies well east of the fault, but seepage increases gradually above the intersection of river and fault near Manzanar Road. From there south to Lone Pine many small springs occur in and along the channel, probably the combined result of channel entrenchment, proximity of the earthquake fault, and inflow from the alluvial fan of Lone Pine Creek.
Under natural conditions, maximum monthly discharge of Owens River normally occurred in June or July with melting of the Sierra snowpack; minimum discharge usually came in August or September. River flow was comparatively regular: the ratio of maximum to minimum monthly discharge at Pleasant Valley from 1919 through 1940 was 2.8 to 1[3] —much lower than, for example, the Kings River at Piedra (46.4 to 1); the Kern at Bakersfield (13.4 to 1); and the Kaweah at Three Rivers (53.6 to 1) (USDI Geological Survey 1959). Factors responsible for this low variation may
[1] Paper presented at the California Riparian Systems Conference. [University of California, Davis, September 17–19, 1981].
[2] Timothy S. Brothers is a Graduate Student in the Department of Geography, University of California, Los Angeles, Calif.
[3] Unpublished streamflow records, Los Angeles Department of Water and Power (LADWP).
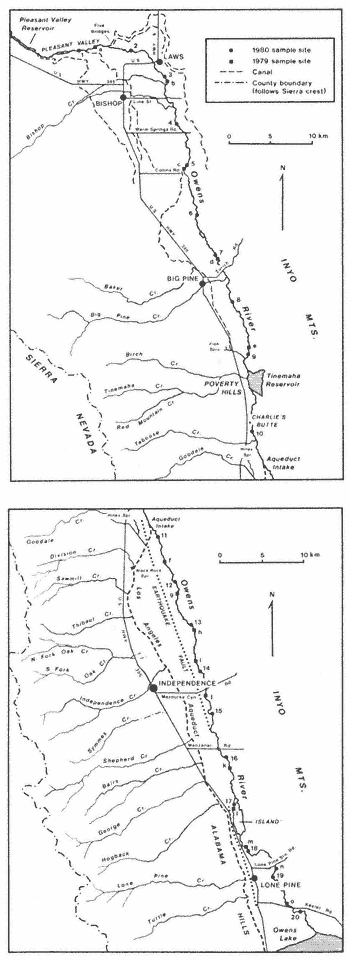
Figure 1.
Owens Valley.
have included the gentle gradient of the river channel; low precipitation east of the Sierra crest and regulation of the discharge by the Sierra snowpack and groundwater. River regime and channel morphology suggest that most floods were relatively mild events, causing little disturbance of the floodplain surface.
Present Vegetation
Sampling Methods
Field sampling of the present riparian woodland was carried out in August, 1979, and in April, May, and June, 1980. (Only data from 1980 are included here.) At each of 20 systemically spaced sites, three parallel l-m.-wide belt transects were extended 20 m. apart at right angles to the river channel. Presence of woody perennial species was noted in each meter of the transect. At sites below the aqueduct intake, transects extended across the channel and 35 m. outward from the bank top on each side. Above the intake, water in the channel limited sites to one side of the river; transects at these sites extended 35 m. from the bank top.
Trees were counted at each site within a plot 50 m. wide, ending 50 m. from the bank on each side of the river (one side only at sites 1–10). Plots thus measured 50 m. by 50 m. above the bank on each side, but area of the channel section at sites 11–20 varied with channel width. Trees less than 2 m. tall were counted as a subset of the total tree population at each site to provide a rough measure of recent reproduction from seed.
Species Composition and Patterning
The Owens River riparian woodland is somewhat species-poor compared to many other riparian areas in California (Roberts, Howe, and Major 1977). Of the 17 woody perennials observed in 1980 (tables 1 and 2), only 10 are primarily members of the riparian woodland: valley willow (Salixgooddingii ), black willow (S . laevigata ), and cottonwood (Populusfremontii ), which make up the tree stratum; and the shrubs narrowleaf willow (Salixexigua ), Rosawoodsii , rabbitbrush (Chrysothamnus nauseosus ), Nevada saltbush (Atriplextorreyi ), salt cedar (Tamarixramosissima ), Russian olive (Elaeagnusangustifolia ), and desert olive (Forestieraneomexicana ). The remaining species are found more often as members of desert scrub communities bordering the floodplain. Of these ten, all except rabbitbrush and Nevada saltbush grow mostly near the present river channel. The latter two also grow down to the water's edge, but they are more abundant on higher ground and are the only woody species present on much of the floodplain outside the immediate vicinity of the river.
Herbaceous species form an understory throughout the riparian woodland. Areas that remain wet most of the year support marsh vegeta-
|
|
tion dominated by Typha , Scirpus , Carex , and Juncus . On much of the floodplain, however, the herb layer is a dense perennial sod of Distichlisspicata , Sporobolusairoides , and Juncus balticus .
At the boundary between floodplain and valley floor, vegetation on both sides of the river changes in most areas to a desert scrub dominated by Sarcobatusvermiculatus , Atriplexconfertifolia , and A . parryi . Less frequently encountered are Artemisiaspinescens , A . tridentata , Atriplexcanescens , A . polycarpa , Daleafremontii , D . polyadenia , Tetradymiaglabrata , and T . axillaris .
Species turnover along Owens River is low: eight of the ten most common riparian woodland species occur at least sporadically throughout the study area. The exceptions are desert olive, not observed north of Manzanar Road, and Russian olive, encountered only between the aqueduct intake and Mazourka Canyon Road. Nevertheless, the character of the woodland varies greatly from one end of Owens Valley to the other because of differences in the abundance of the few species present, as shown in tables 1 and 2. (Sites 11–20 are separated into above-bank and channel sections to allow better comparison with sites 1–10, where transects did not extend into the channel.)
North of Bishop, the woodland consists mostly of dense stands of Rosawoodsii , narrowleaf willow, and rabbitbrush that extend well back from the river on low ground (fig. 2). Tree cover (mostly valley willow) increases below Bishop, and the frequency of R . woodsii and narrowleaf willow declines, though both remain common to the aqueduct intake. Rabbitbrush and Nevada saltbush are abundant everywhere; both were observed at or near all sites north of the intake.
Below the intake an immediate change occurs. Few trees grow on the floodplain surface south to Mazourka Canyon Road; woody vegetation above the
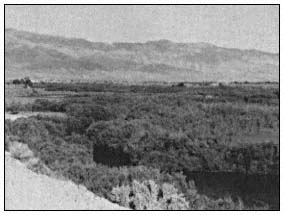
Figure 2.
Owens River north of Bishop.
banks consists almost entirely of Nevada saltbush and rabbitbrush, with salt cedar scattered along dry washes (fig. 3). The dry channel bottom is lined with Atriplex and Tamarix , though trees become more frequent southward. Russian olive grows sparsely on the floor of the channel for several kilometers north of Mazourka Canyon Road. Narrowleaf willow and R . woodsii were observed only in the channel, at places where damp soil indicated a shallow groundwater table.
Below Mazourka Canyon Road, tree cover continues to increase both in the channel and along the banks (fig. 4). Salt cedar becomes less frequent and rabbitbrush more so; Rosa woodsii and narrowleaf willow are also more common, but for the most part do not occur in the large stands found in the Bishop region.
Like the woody overstory, most herbaceous species become less abundant along the dry channel. In contrast to the thick sod present in other areas, much of the floodplain surface in this section is bare except for Salsola iberica , Bassiahyssopifolia , and other weedy annuals.
Few tree seedlings were seen north of the aqueduct intake except at site 8 (table 3). Seedlings are also absent in most of the dry section below the intake, but become more common from site 14 south in the channel bottom.
Human Impact
Early Descriptions of the Riparian Woodland
Descriptions of the riparian woodland as it appeared before European settlement are few and somewhat contradictory. Expeditions escorted by Joseph Walker passed through Owens Valley in 1834, 1843, and 1845 (Goetzmann 1966; Davidson 1976), but apparently none left a first-hand account of vegetation along the river. The 1845 expedition was directed, but not accompanied, by
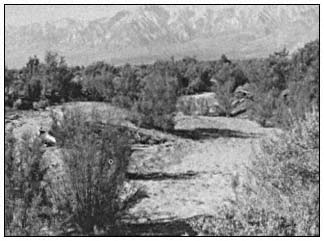
Figure 3.
Salt cedar lining dry bed of Owens River near Independence.
|
John C. Fremont, who later described Owens River as "wooded with willow and cottonwood" (Fremont 1849), perhaps on the basis of descriptions furnished him by members of the expedition. Much of Owens Valley was surveyed by A.W. von Schmidt in 1855, but his field notes only mention riparian vegetation near Lone Pine, where von Schmidt encountered willows along the river.[4] A cor-
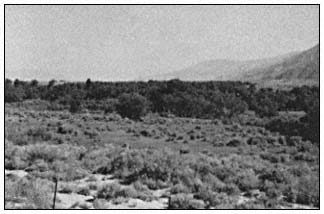
Figure 4.
Owens River near Keeler Road.
respondent accompanying a military expedition through Owens Valley in 1859 described it as untimbered except for a few small cottonwoods (Davidson 1976). William Brewer, of the California Geological Survey, encountered no trees at all in the valley in 1864 (Brewer 1930). An 1886 settler's tract states that before settlement there was "no timber of any kind" in Owens Valley; the river was bordered by "grassy plains" dotted with occasional shrubs (Anon. 1886). An early inhabitant of the valley, however, recalled large willows lining the river east of Independence (Earl 1976).
These accounts suggest that tree cover has always been sparse along Owens River, though it seems unlikely that trees were ever entirely absent. Early explorers may have kept mostly to the west side of the valley, where travel is easier but the river is often hidden from view. Although no clear picture of the presettlement riparian woodland emerges from these descriptions, examination of the history of land use and water management in Owens Valley provides indirect evidence of the changes that may have occurred since settlement.
Mining
Between 1860 and 1864 numerous claims were located in the Inyo and White Mountains, and four mining towns were established near Owens River (Chalfant 1922). Reduction works were built at five sites near the river, at least two of them
[4] USDI Bureau of Land Management. Surveyor General of California. 1855. Unpublished surveyor's field notes, books 115, 203, 296. On file at Bureau of Land Management, Sacramento, Calif.
requiring wood or charcoal for operation (Raymond 1869). Although additional discoveries were made in the valley after 1864, the early excitement died out rapidly. By 1870, three and perhaps all four of the small mining towns were deserted, and most of the mills were idle (California State Mining Bureau 1888). The impact of this short-lived mining boom on the riparian vegetation of Owens River was probably small. Canals were dug from the river to at least two mills, but their construction entailed little disturbance of the riparian zone. Trees may have been cut near the river to supply the mills, but most wood no doubt came from the mountain slopes flanking Owens Valley.
Browsing
Livestock
Livestock were driven through the valley as early as 1859; by 1861 permanent ranches were established near present-day Bishop (Chalfant 1922; Davidson 1976). Local stock were wintered on the meadows of the Owens River floodplain and other shallow-groundwater areas on the valley floor, then moved to mountain pastures in summer (Earl 1976). In addition, large herds of sheep were driven through Owens Valley to mountain ranges each spring (Anon. 1886), and in dry years cattle and sheep were driven into the valley to take advantage of its subirrigated grasslands (California State Mining Bureau 1888).
Stock raising suffered along with the rest of the valley economy as Los Angeles bought up land for the Los Angeles Aqueduct. The greatest decline took place after 1923, when the city began to buy land in the Bishop-Big Pine region. Collapse of the industry was averted by Los Angeles' decision to lease some land back to ranchers. Expansion of the lease program was encouraged by a series of wet years beginning in 1936 and by completion of the aqueduct extension to Mono Lake in 1940 (Los Angeles Department of Water and Power 1966). Most of the valley floor, including the floodplain of Owens River, is now occupied by large grazing leases devoted almost entirely to cattle production. Animals are still moved seasonally to mountain range, but some ranchers keep stock in the valley year-round. In general, stock graze the river lands from October or November to March or April, and are absent the rest of the year.
Without detailed historical records, the impact of livestock on the riparian woodland must largely be surmised. The river grasslands provide a perennial water supply and abundant forage, and have probably always been used more heavily than the surrounding desert range. Grazing pressure may have become severe in dry years before institution of the present lease program, but if overgrazing occurred it seems not to have been recorded. Creation of large leases reduced fluctuations in the size of the valley's livestock population, and probably also reduced summer grazing intensity near the river. Determination of range carrying capacity has been left to leaseholders, however, and some overstocking may have occurred since leasing began.
Regardless of past stocking levels, livestock have probably exercised selective pressure on the composition of the riparian woodland. All of the shrub species in the woodland are browsed, but some are of low palatability or have weedy characters that enable them to persist despite browsing. Narrowleaf willow spreads by means of root suckers, in some areas forming dense stands that appear to exclude livestock. Rabbitbrush is well known as an invader of overgrazed rangeland and other disturbed areas in the West. Presence of livestock in the riparian zone may have favored these shrubs at the expense of other species. Livestock browse mature willow trees very lightly, according to local ranchers—perhaps partly because most animals are removed from bottomland pastures when the trees are in leaf. Browsing does not appear to injure mature trees greatly, though some have browse lines. Damage to seedlings may be greater: many of those observed during field work appeared to have been cropped by browsing animals.
Tule Elk
An initial herd of 54 tule elk (Cervuselaphus nannodes Merriam) was released north of Independence in 1933 and 1934. The herd multiplied to 189 head by 1943, but has since fluctuated between 150 and 500 because of hunts held to control population size (McCullough 1969). At least four herds of elk now browse near Owens River. Although seasonal movements vary from one herd to the next, elk may use most of the bottomland from Owens Lake to the bend north of Bishop in the course of a year (Curtis etal . 1977).
The elk subsist on browse plants, particularly on willows, to a much greater degree than do livestock (McCullough 1969). They may have contributed to development of browse lines on some trees, but like livestock are more likely to have damaged seedlings than mature trees. Nevertheless, the elk population is small in relation to the livestock population, and its effect on the riparian woodland is probably small by comparison.
Beaver
Beaver were introduced to Owens Valley by the California Department of Fish and Game at Baker Creek in 1948.[5] They have since spread to Owens River, both above the aqueduct intake and in the spring-fed section below it.
Beaver feed on cottonwood and on both willow tree species. They often gnaw willows completely through, but the willows are able to stump-sprout if not further disturbed. Large cottonwoods are often girdled and completely killed. Beaver are
[5] Unpublished records, California Department of Fish and Game.
found the entire length of the upper river, but their activity is localized. In some areas almost all trees have been affected; in others they remain untouched. Approximately 24% of the trees at sites 1–10 showed signs of gnawing by beaver. Despite the presence of beaver in the lower river, none of the trees at sites 16–20 appeared to have been damaged.
Crop Cultivation
Cultivation of crops began in the 1860's, largely as an adjunct to the livestock industry. Farms were at first clustered along creeks on the west side of the valley, but later spread onto the valley floor following construction of large irrigation canals from Owens River. Expansion of irrigated cropland was greatest near Bishop and Big Pine, where approximately 17 canals began operation between 1872 and 1890.[6]
Los Angeles' acquisition of valley lands halted irrigation of cropland from Owens River by about 1930.[7] Although Los Angeles began to make water available again in the late thirties for irrigation of pasture and fodder crops on grazing leases, local irrigation allotment has depended on annual runoff and cultivated acreage has remained small (Los Angeles Department of Water and Power 1979).
Land-use maps compiled for most of the valley floor early in this century[8] suggest that, despite the extensive canal system, little land was ever farmed near Owens River. A few fields were cultivated on the floodplain at Big Pine and Bishop, but there as elsewhere most of the bottomland was left in native pasture, probably because of its shallow water table and salt-affected soils.
Agricultural land clearing thus caused no wholesale reduction of the riparian woodland in Owens Valley. Cultivation and abandonment of the few fields on the floodplain may have encouraged the growth of rabbitbrush—a frequent invader of abandoned fields in the Bishop area—but the maps cited above show vegetation patterns approximating those of today: the floodplain largely covered by herbaceous growth, with willows confined to the river and other runoff channels.
Fire
Intentional burning has been practiced at one time or another along most of Owens River. Ranchers probably burned the bottomland pastures occasionally in pre-aqueduct days, and present leaseholders say that intentional burning occurred on many leases from shortly after institution of the lease system until about 1970. During the sixties, for example, the floodplain was burned between Lone Pine Station Road and Mazourka Canyon Road, and between Pleasant Valley Reservoir and Five Bridges (north of Bishop). The latter area has been burned several times, and part of it sprayed with herbicides, to improve livestock access to the river.
The incidence of wildfires has probably increased along the upper river with greater use of the riparian zone by campers and fishermen in the last 30 years. Local residents recalled several recent wildfires on the floodplain, including a large one northeast of Bishop in about 1968. I found evidence of burning at 12 of the 20 sites sampled in 1980 (sites 1–3, 6, 12, and 14–20).
The riparian woodland may have been affected most by fire north of Bishop. Repeated burns there could have eliminated willow and cottonwood trees and favored faster-maturing shrubby species like rabbitbrush and narrowleaf willow, which resprout readily after fire. The impact of burning is difficult to assess, however, without better information about the post-fire responses of the other woodland species.
Plant Introductions
Two Eurasian shrubs, Russian olive and salt cedar, have become established in the riparian woodland as an indirect result of human dispersal. Both were probably introduced to the Southwest as ornamental plants in the nineteenth century, but have since become naturalized along many southwestern watercourses (Christensen 1963; Robinson 1965). Earliest evidence I have found of Russian olive in Owens Valley is a 1942 collection from naturalized plants growing along Lone Pine Creek,[9] but it had probably been introduced in cultivation long before then. Russian olive appears to have become established on Owens River only in the Independence region, where it is scattered along the bottom and sides of the dry channel. Salt cedar reached Owens Valley at least by 1944, when aerial photographs show it growing along the 1872 earthquake fault near Mazourka Canyon Road.[10] It too may first have been introduced as an ornamental, but has now become naturalized throughout Owens Valley and is a notable element of the riparian woodland
[6] Los Angeles Department of Water and Power [n.d.]. History Owens Valley irrigation ditches. Unpublished report by J.L. Graham.
[7] Unpublished canal discharge records, LA DWP.
[8] Los Angeles Department of Water and Power. Map of Bishop region in Owens Valley [unpublished]. Prepared under the direction of C.H. Lee. 6 sheets. Scale 1:12,000.
Los Angeles Bureau of Water Works and Supply [n.d.]. Detail map of a portion of Owens Valley near Lone Pine, California [unpublished]. 2 sheets. Scale 1:6000. On file at LADWP.
[9] Constance, Lincoln. 1980. Personal communication.
[10] Los Angeles Department of Water and Power. 1974. Vegetational resource inventory and potential change assessment, Owens Valley, Calif. Unpublished report by Earth Satellite Corporation.
south of the aqueduct intake. Establishment of salt cedar and Russian olive along Owens River appears related to alteration of the river's natural regime by the Los Angeles Aqueduct system (discussed below).
Water Management
Irrigation
Most irrigation diversions in Owens Valley began before 1890; by 1904 it was estimated that over 75% of the annual runoff in the valley was diverted for irrigation.[11] These diversions reduced the total discharge of Owens River and altered its natural regime: maximum and minimum flows were both reduced downstream by large summer irrigation diversions, but winter flow was almost doubled by agricultural drainage.[12] Flood frequency and magnitude doubtless diminished as well.
These changes may have affected regeneration of willows and cottonwoods, whose reproduction is tied to the natural runoff regime. Reduction of maximum and minimum flows could have decreased the area of freshly exposed alluvium available for colonization during the growing season or shifted the zone of seedling establishment downward in the channel, where young plants would be more susceptible to damage by the increased winter discharge. Consumptive use of water by agriculture would also have decreased the total water supply to riparian vegetation downstream. The effects of irrigation have since been obscured, however, by changes associated with more recent water management practices.
The Los Angeles Aqueduct System
Operation of the Los Angeles Aqueduct system began with completion of the aqueduct in 1913. The aqueduct had little effect on Owens River above the intake until 1929, when Tinemaha Dam began to regulate flow to the aqueduct at the Poverty Hills. The rest of the upper river remained unregulated until 1941, when Long Valley Dam was completed at the head of Owens River Gorge. Pleasant Valley Dam was added in 1957 to smooth out fluctuations caused by powerplants below Long Valley Dam.
Natural river discharge has been increased above the intake by interbasin transfers and groundwater pumping. The Mono Basin extension of the aqueduct system began diverting water to Owens River at Long Valley in 1941, and diversions increased following construction of the Second Los Angeles Aqueduct (located outside the study area) in 1970. These increases can be seen in the record of annual river discharge at Pleasant Valley (fig. 5).
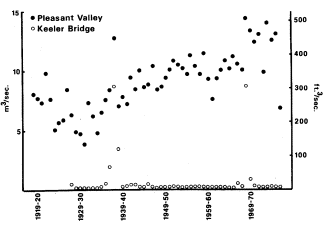
Figure 5.
Annual discharge of Owens River at
Pleasant Valley and Keeler Bridge.
Source: unpublished streamflow records, LADWP.
During a prolonged dry period in the twenties and thirties, Los Angeles drilled approximately 170 wells in Owens Valley to increase supply to the aqueduct (Los Angeles Department of Water and Power 1966). Many of these wells were located north of the intake and discharged water into the river channel rather than the aqueduct. Pumping occurred from 1919 through 1935, then halted until the dry years 1960–62 (Los Angeles Department of Water and Power 1979). More wells were drilled after completion of the Second Aqueduct, and pumping now occurs every year.
South of the aqueduct intake, Owens River and most other streams have been diverted by the aqueduct since 1913, though some water continued to flow down the lower channel past the intake in most years until Tinemaha Dam provided a means of storing high flows. Since then water has been shunted downstream on just a few occasions, mostly when runoff has exceeded system capacity: in 1936–39 (before completion of Long Valley Dam), then again in 1967, 1969, and 1975.[13] The 1938 and 1969 flows were exceptionally large, as can be seen from the record at Keeler Bridge east of Lone Pine (fig. 5).
Groundwater seepage has maintained some surface flow in the lower channel south of its intersection with the 1872 earthquake fault. This discharge has been quite small, often dropping to almost nothing from July through September, but relatively constant from year to year when no water is released past the intake.
Vegetation change associated with operation of the aqueduct system should be greatest in the section of channel between the aqueduct intake and the river-fault junction, which has remained dry since 1929 except for scattered seeps and irregular flood discharges. Field data (tables 1
[11] U.S. Reclamation Service. 1904. Report on the Owens Valley, California. Unpublished report by J.C. Clausen. On file at the Los Angeles Public Library, Water and Power Branch.
[12] Unpublished streamflow records, LADWP.
[13] Unpublished streamflow records, LADWP.
and 2) support this conclusion: diversion of surface flow appears to have eliminated most of the native riparian woodland from this area, except for the species least closely associated with riparian zones. Riparian taxa persist mainly in the channel bottom just north of the fault, where groundwater is most readily available. South of the fault, the small surface flow provided by spring discharge has evidently been sufficient to maintain much of the original riparian cover.
Establishment of salt cedar and Russian olive in the woodland is also best explained as a consequence of aqueduct system operation. Although salt cedar was perhaps naturalized near the river by 1944, local residents say that it became common only after flooding of the lower river in 1967 and 1969. The shrub spread rapidly into flooded areas, becoming especially dense in the dry section below the intake. Salt cedar is similar to the native willows and cottonwoods in being adapted for establishment after such fluvial disturbances, but here as elsewhere in the Southwest it has been better able than the native flora to colonize a habitat created by alteration of the natural runoff regime. Its success may be partly attributable to such inherent ecological advantages as more prolonged annual seed production and lower moisture requirement than the native riparian species (Horton 1977), Water was released down the old channel during the entire summer of both 1967 and 1969, perhaps delaying most seedling establishment until only salt cedar was still dispersing seed. On the other hand, willow and cottonwood seedlings may have been present initially but failed to survive without a permanent water supply.
When Russian olive reached the river is not certain, but the small size of all individuals observed during field work suggests that it too may have become established since 1969. It has not become as widespread as salt cedar, perhaps because its olive-like fruits are not wind dispersed.
Above the aqueduct intake, operation of the aqueduct system has changed the natural regime of Owens River (fig. 6): instead of cresting early in summer and falling rapidly to a late-summer minimum, the river now remains high from spring through fall, and maximum monthly discharge often occurs at the time of former low water. Flood magnitide and frequency have decreased, because flood discharge can be diverted or stored upstream. Similar changes in natural river regime have been found to hinder reproduction of riparian species elsewhere by eliminating the substrate necessary for seedling establishment (Johnson etal . 1976), and may partly account for the scarcity of young cottonwood and tree willow seedlings along the upper river channel. (The single exception, at site 8, is difficult to explain since other, apparently similar sites are devoid of young trees, but it does show the potential for seed reproduction where favorable conditions exist.) Seed reproduction appears more active in the channel itself below the earthquake fault, the only area in which river discharge still rises and falls more or less naturally.
Since 1970, tree regeneration along the upper river may also have been reduced by erosion associated with increased import for the Second Aqueduct. Bank slumping has in many areas produced vertical, unstable channel sides on which seedlings are unable to take root.
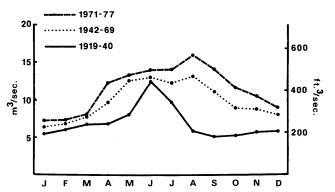
Figure 6.
Mean monthly discharge of Owens River at Pleasant Valley.
Source: unpublished streamflow records, LADWP.
Conclusions
The most obvious human-caused changes in the Owens River riparian woodland have stemmed from diversion of the river by the Los Angeles Aqueduct. Elimination of much of the native riparian vegetation between the intake and the earthquake fault has created an open niche that has been partly filled by salt cedar and Russian olive with recent flooding of the old channel. Both species will probably continue to spread in the future because of the need to waste water from the aqueduct system in very wet years.
Below the fault, native riparian vegetation has been maintained by spring flow, and has in fact spread into the channel with reduction of river discharge. The riparian woodland may be as dense now in some parts of the lower river as it was before completion of the aqueduct.
North of the intake, the combined effects of browsing, burning, clearing, and water management may have caused a reduction in the tree cover and an increase in such weedy shrubs as rabbitbrush and narrowleaf willow—especially near Bishop, where human impact has been most concentrated. Lack of seed regeneration by native tree species along the upper river may be due to browsing or the disruption of the natural river regime. Nevertheless, sexual regeneration of riparian species could be naturally uncommon along Owens
River. Historical records suggest that the river's unusually small annual discharge range, together with a shallow groundwater table and relatively erosion-resistant floodplain material, allowed development of a dense growth of herbaceous perennials that covered much of the floodplain and often extended, as at present, right to the water margin. Competition from this herbaceous cover could have limited most seedling establishment to areas of intense fluvial disturbance, perhaps present only after infrequent major floods. In this regard the dynamics of the riparian zone along Owens River may have differed from those of many other western streams, which annually rework a much wider expanse of alluvium because of their greater discharge range and might provide greater opportunity for seed regeneration. Comparison of the relative success of vegetative and seed reproduction in relation to discharge regime in other riparian systems might clarify processes in the Owens River woodland.
Regardless of whether tree regeneration is being artificially suppressed or is naturally uncommon, the present scarcity of young trees along the upper river may portend further decline of the tree cover. Management of the river discharge for aqueduct operations will no doubt continue to prevent most flooding, and tree establishment may be increasingly confined to the immediate river channel, which is now near bankful stage much of the time.
Literature Cited
Anon. 1886. Inyo County, its resources and attractions for settlers. Inyo Independent Printers, Independence, Calif.
Brewer, W.H. 1930. Up and down in California in 1860–1864. F.P. Farquhar (ed.). Yale University Press, New Haven, Conn.
Chalfant, W.A. 1922. The story of Inyo. Published by the author.
Christensen, E.M. 1963. Naturalization of Russian olive (Elaeagnusangustifolia L.) in Utah. American Midland Naturalist 70:133–137.
Curtis, B. etal . 1977. Owens Valley tule elk habitat management plan. 2 sections.
Davidson, J.W. 1976. The expedition of Capt. J.W. Davidson from Fort Tejon to the Owens Valley in 1859. In : P.J. Wilke and H.W. Lawton (ed.). Publications in archaeology, ethnology, and history, No. 8. Ballena Press, Soccorro, N.M.
Earl, G.C. 1976. The enchanted valley and other sketches. Arthur H. Clark Co., Glendale, Calif.
Fremont, J.C. 1849. Notes of travel in California. James M'Glashan, Dublin, Ireland.
Goetzmann, W.H. 1966. Exploration and empire: The explorer and the scientist in the winning of the American West. Alfred A. Knopf, New York, N.Y.
Goodyear, W.A. 1888. Inyo County. p. 224–288. In : Eighth annual report of the state mineralogist. California State Mining Bureau.
Horton, J.S. 1977. The development and perpetuation of the permanent tamarisk type in the phreatophyte zone of the Southwest. p. 124–127. In : R.R. Johnson and D.A. Jones (ed.). Importance, preservation and management of riparian habitat. USDA Forest Service General Technical Report RM-43.
Johnson, W.C., R.L. Burgess, and W.R. Keammerer. 1976. Forest overstory vegetation and environment of the Missouri River floodplain in North Dakota. Ecological Monographs 46:59–84.
Los Angeles Department of Water and Power. 1966. Report on water supply management in Inyo and Mono Counties. Prepared by R.V. Phillips etal .
Los Angeles Department of Water and Power. 1979. Final environmental impact report: Increased pumping of the Owens Valley groundwater basin. 2 volumes.
McCullough, D.R. 1969. The tule elk: Its history, behavior, and ecology. University of California publications in zoology, Vol. 88. University of California Press, Berkeley, Calif.
Raymond, R. 1869. Statistics of mines and mining in the states and territories west of the Rocky Mountains. First annual report of the U.S. commissioner of mining statistics. Government Printing Office, Washington, D.C.
Roberts, W.G., J.G. Howe, and J. Major. 1977. A survey of riparian forest flora and fauna in California. p. 3–21. In : A. Sands (ed.). Riparian forests in California: Their ecology and conservation. Institute of Ecology Pub. 15. 122 P. University of California, Davis, Calif.
Robinson, T.W. 1965. Introduction, spread and areal extent of salt cedar (Tamarix ) in the western states. U.S. Geological Survey Professional Paper 491-A.
USDI Geological Survey. 1959. Compilation of records of surface waters of the United States through September, 1950. Part 11-B. Pacific slope basins in California, Central Valley. Water-Supply Paper 1315-A.
The Transitional Nature of Northwestern California Riparian Systems[1]
R. Chad Roberts[2]
Abstract.—Within the region between the Sacramento Valley and the northwestern California coast, riparian vegetation undergoes a change in species composition apparently related to floristic history, climate, and local conditions. While forest areas appear structurally convergent, there may be significant regional taxonomic differences. Each plant species shows an individualistic response. Broad-niched nonriparian species may constitute the riparian forest in some sites. The vegetation type is used by many bird species distributed throughout the area; northcoast forests appear to have more resident species, but lack oak tree specialists.
Introduction
This paper presents a first look at biogeographic patterns in northwestern California riparian systems. Sacramento Valley riparian studies were just being planned a decade ago, motivated by the imminent demise of the native forests. To many northwestern California residents, riparian forests are a nuisance, an income source from logging, or a hindrance to agriculture. At best, the riparian strips are known to provide flood protection, and may be considered picturesque. Given these local viewpoints, it is not surprising that no studies similar to those of the last decade in the Sacramento River system have been done.
The 1980's should see such studies; northwestern California riparian systems are both different from those in the Sacramento Valley, and are subject to similar conversion pressures. These attributes raise a plea for attention from academic scientists, conservation biologists, and agency personnel. This paper is motivated to extend the concern that has developed for riparian systems elsewhere to include northwestern California. I show here how the biogeographic face of riparian forest changes within this region, and suggest preliminary explanations for the changes.
Interested readers are encouraged to investigate the area for themselves.
Methodology
Because this was a preliminary survey, no attempt was made to be quantitative or to rigorously test alternative hypotheses. These actions are logically deferrable to more detailed secondlevel studies. Information used herein was derived from field sampling and literature descriptions. Field sampling involved recording tree species, most shrub species, and common herbaceous species at sample sites within the study area. In most cases, notes were made of adjacent nonriparian vegetation. Additionally, (given my training and long-standing predilection) I recorded all bird species encountered.
Sample site choice was decidedly nonrandom. The coastal areas in Humboldt and Del Norte Counties are better covered than interior areas, and these counties are better sampled than inland counties. The Eel River's South Fork, the lower Mad River, and the Trinity River drainage are better sampled than are other basins.
The area covered by this survey extends from the lower Sacramento Valley (Yolo County; see figure 1) north to western Siskiyou County. West of that transect, the study area includes Del Norte, Humboldt, Trinity, northern Mendocino, Lake, and eastern Napa Counties. The area from southern Mendocino County to San Pablo Bay and nearby areas is specifically excluded.
Numerous literature sources proved useful, including descriptions accompanying mapping, planning documents and agency descriptions, published papers, and textbook descriptions. Some of these are cited below; others will be cited in subsequent papers.
[1] Paper presented at the California Riparian Systems Conference. [University of California, Davis, September 17–19, 1981].
[2] R. Chad Roberts is a Staff Environmental Analyst with Oscar Larson & Associates, P.O. Box 3806, Eureka, Calif.
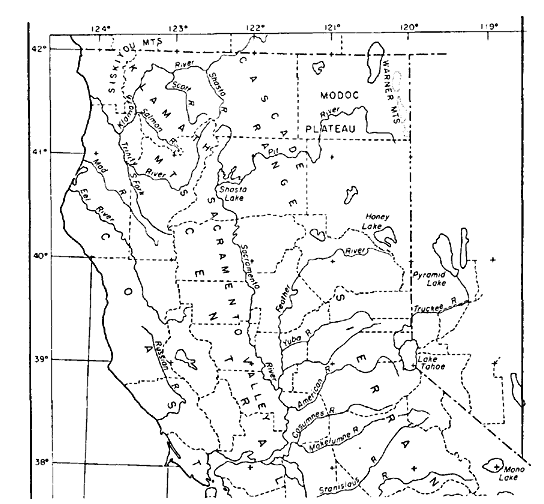
Figure 1.
County outline map of northern California, showing study area and its
relationship to other areas. Adapted from Griffin and Critchfield (1972).
Results
Plant Biogeography
Species
Certain tree and shrub species are often considered characteristic of riparian systems, due either to requirements for high soil water content or to a tolerance of it. In this paper I assume that this requirement and/or tolerance is the criterion of riparian species in general. Other factors (light intensity, flood-scour potential, seed-source proximity, history, and competition) are likely to affect species distribution as well. Two distinct species groups comprise riparian forests in the study area. The following summaries deal only with tree species, but shrubs and herbaceous plants show similar (or even greater) regional differentiation.
SouthernGroup .—The study area includes parts of the Central Valley Riparian Forest region and most of the North Coast Riparian Forest region of Roberts etal . (1980). The entire west side of the Sacramento River Valley below elevations of 914 to 1218 m. (3000 to 4000 ft.) contains Foothill Woodland (Munz and Keck 1959), the riparian face of which includes species such as valley oak (Quercuslobata ), interior live oak (Q . wislizenii ), blue oak (Q . douglasii ), California buckeye (Aesculuscalifornica ), Fremont cottonwood (Populus fremontii ), and digger pine (Pinussabiniana ). In the study area's southern end, Hind's walnut (Juglans hindsii ) is a conspicuous element, and larger creeks may have Oregon ash (Fraxinuslatifolia ) and box elder (Acernegundo ).
The small creeks draining the inner Coast Ranges largely lack the extensive and diverse flora found beside major rivers (Conard etal . 1980). Larger creeks (Putah Creek, for example) have riparian forests containing many typical riparian species, but these disappear from riparian systems to the northwest. Cache Creek resembles the smaller creeks in its riparian borders. Figure 2 shows a typical riparian section from a southern creek.
NorthernGroup .—As a useful generalization, the opposite extreme of southern group creeks is the small coastal streams of Del Norte and Humboldt Counties. Elk Creek in Del Norte County has a relatively undisturbed, secondgrowth riparian forest, with red alder (Alnusrubra ), Pacific wax-myrtle (Myricacalifornica ), California bay (Umbellulariacalifornica ), and madrone (Arbutusmenziesii ) the most abundant hardwoods. North coast riparian forests contain a significant conifer element; Elk Creek includes Sitka spruce (Piceasitchensis ), Douglas-fir (Pseudotsugamenziesii ), redwood (Sequoiasempervirens ), and several other conifer species (Del Norte County Planning Dept. 1979). The same species are characteristic of small, coastal streamchannel forests from southern Oregon as far south as Ft. Bragg in Mendocino County. Since most coastal streams have been logged at least once, however, the conifer element is often less conspicuous, or is missing entirely.
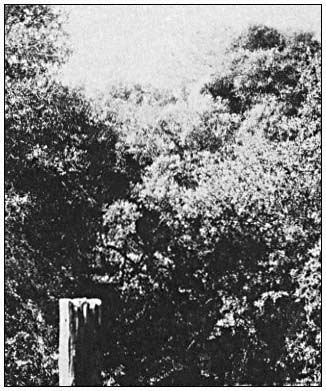
Figure 2.
Riparian vegetation bordering Chickahominy Slough, Yolo
Co. Blue oak, interior live oak, and digger pine are evident.
North coastal riparian forests also contain diverse shrub and understory floras, including several willow (Salix ) species, several berry (Rubus ) species, two huckleberry (Vaccinium ) species, Pacific red elder (Sambucus callicarpa ), cascara (Rhamnuspurshiana ), and a number of others (Del Norte County Planning Dept. 1979; Roberts etal . 1980; McLaughlin and Harradine 1965). Most of these species are equally characteristic of other nonriparian zones near the streams, suggesting that they may not be obligate riparian species. This appears to be true of most riparian tree species listed above, though the species appear to achieve their best growth in riparian situations.
South or inland of coastal Del Norte County, additional species occur which are characteristic of the northern species group. Bigleaf maple (Acermacrophyllum ) is an important redwood forest understory component, reaching maximum growth as a riparian species. Bigleaf maple occurs from the coast to the Sierra Nevada foothills (Griffin and Critchfield 1972); in eastern Shasta County it is often a shrub restricted to stream vicinities. Figure 3 shows a typical north coastal riparian forest.
Inland from the coast, additional riparian species are encountered that appear to be part of the northern species group. Oregon ash is a major riparian component in the Klamath River region of eastern Humboldt County, as well as in the upper Eel basin in northern Mendocino County. It occurs throughout the Trinity River basin and is still prominent in the Cottonwood Creek basin southwest of Redding. Oregon ash is present, but not prominent, in the North Fork, Cache Creek drainage, Lake County.
White alder (Alnusrhombifolia ) replaces red alder away from the coast, assuming the riparian alder role. It is present in the upper Eel River basin of southern Humboldt, where its range overlaps the red alder (A . rubra ), and is common throughout the Klamath, Trinity, and

Figure 3.
Riparian forest, Mad River, Humboldt Co.; including
red alder, bigleaf maple, and Pacific elderberry.
upper Sacramento River basins. I have noted it in the upper Russian River drainage in eastern Mendocino County, but am uncertain of its abundance south and east of Clear Lake.
A major northern group species is the black cottonwood (Populustrichocarpa ). It is largely restricted to riparian zones, and forms the dominant structural component in floodplain forests in the lower Eel, Klamath, and upper Trinity River systems. It is not common in coastal areas, per se , reaching the Pacific only in the Eel, Mad, and Klamath River floodplains (fig. 4). The same gallery forests in the Eel (and probably the Klamath) River valley contain large willow trees, up to 24 m. (80 ft.) tall. At least Pacific willow (Salixlasiandra ) is present as a tree, along with black cottonwood and red alder. These riparian gallery forests are impressive for their luxuriance and structural diversity (fig. 4).
A third group of species exists which is technically related to the northern group. Generally part of the mixed evergreen association, it is composed of species found in the Klamath and Siskiyou Mountains and the interior Coast Ranges. Several species in this group occupy the riparian zone along creeks or rivers throughout much of the study area, in much the same way foothill woodland species do in the southern group. The most prominent facultative
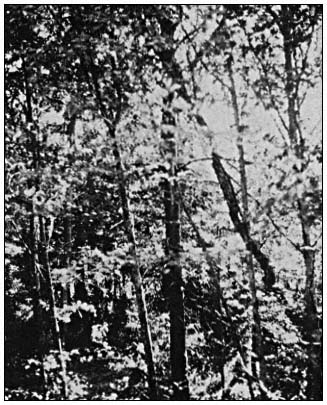
Figure 4.
Riparian gallery forest, Eel River, Humboldt Co.
Red alder, Oregon ash, black cottonwood, and
willow constitute the dominant vegetation.
riparian species appear to be canyon live oak (Quercuschrysolepis ), tanoak (Lithocarpusdensiflorus ), and to a lesser extent black oak (Q . kelloggii ) and garry or Oregon white oak (Q . garryana ). Riparian species such as white alder, bigleaf maple, and dogwood may or may not be present, as is true for Douglas-fir, redwood, madrone, and other facultative riparian species noted above. Figure 5 shows a typical area where these ecologically eurytopic species occupy riparian zones.
Physiography
The words "riparian forest" usually evoke an image of a floristically and structurally diverse community growing by a large river. It is immediately clear that most northwestern riparian forests do not fit that mold. The following three community types appear to reflect on-the-ground conditions.
HeadwatersAreas .—Throughout the study area, the uppermost stream reaches have a border mostly comprised of the common species in the region. Southeastward in the region, chaparral species are prominent in this role; northward and westward, mixed-conifer forest species fill the role. In the Sacramento, Trinity, and Eel River headwater reaches, white alder, bigleaf maple, and various riparian shrubs are usually present.
The small headwaters streams are often actively eroding their channels at or close to bedrock. It appears that a significant physical parameter affecting the plants is the ability to find a foothold and nourishment in the thin alluvial soils of these mountain canyons. In most cases, the streamflow regime provides adequate year-round water.
MidlevelAreas .—As the stream grade flattens, most north coast rivers show gravelbars and sand flats supporting riparian vegetation. Often these are narrow strips squeezed between the river and bedrock hillslopes (fig. 5). Fluctuating water levels and flood scour may
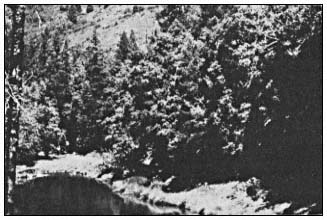
Figure 5.
Midlevel riparian zone, upper Eel River, Mendocino Co. Canyon
live oak, white alder, and Douglas-fir are the major riparian species.
leave relatively little riparian vegetation in these areas. Where a valley is wide enough terraces form, which often support riparian groves.
Community composition on the terraces appears to result from a dynamic process of elimination, colonization, and exploitive competition. There communities typically have "nonriparian" canyon live oaks, tanoaks, or madrones growing in the riparian corridors, with or without alders, Oregon ashes, and other species. Apparently, individuals are eliminated from the community through bank scour or competitive death. Recolonization depends on seed source proximity and dispersal factors. In the midlevel Eel and Trinity River drainages, the result is a mosaic of riparian community types. Throughout much of northwestern California, the characteristic riparian species are white alder, canyon live oak, and Douglas-fir. The same process apparently leads to dominance of foothill woodland species in the Sacramento Valley area riparian communities.
Broad-ValleyFloodplainAreas .—Deposition of a thick sediment layer near abundant water leads to the formation of riparian gallery forests. In the Sacramento River drainage, this is the community primarily addressed in Sands (1980), which is present in this study area only in a few major, interior Coast Range channels. In the westward drainages, the broad floodplains are largely confined to the lower reaches (except in the Weaverville and Scott Valley areas where they are in large part due to gold dredging). Apparently, these areas once supported mixed conifer/deciduous redwood communities such as that on Elk Creek. Those riparian plant communities still present for the most part lack conifers, and consequently resemble deciduous gallery forests in interior river basins (fig. 6).
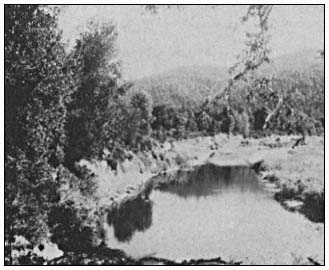
Figure 6.
Floodplain gallery forest, Eel River near Miranda, Humboldt
Co. Black cottonwood and willow have reached large sizes
on terrace probably dating from 1964 flood.
Colonization processes on the major floodplains appear to occur very rapidly. However, these floodplain areas are the primary agricultural areas in the north coast, and barring effective land use regulation, many of the gallery forests are likely to be cleared for agricultural purposes or in flood-control projects.
Bird Biogeography
Several Sacramento Valley studies have catalogued riparian bird use (Gaines 1980, and included references; Motroni 1979; Hehnke and Stone 1979). No studies have been done to date specifically on riparian birds in northwestern California. Gaines (1980) noted 69 species during the breeding season and 66 wintering species in Central Valley riparian forests. Motroni (1979) observed 71 species. Hehnke and Stone (1979) apparently recorded 90 species. By contrast, Harris (1974) listed 142 species for mid-Humboldt County riparian systems, and (1979) 157 species for this type of system in the "North Coast/Cascade Zone" that includes this study area. Taken as a whole, northwestern riparian forests are apparently used by more species than are Central Valley riparian forests.
Gaines (1980) recorded 26 migrant species in the 69-species breeding avifauna (38%), and 30 migratory or sporadic visitors among the 66 wintering species (45%). Harris' (1974) list contains 36 summer visitors and 27 wintering species of the 142 total species, the remainder being residents. After the appropriate adjustments, 31% of the 115 summer species are visitors and 25% of the 106 wintering species are not permanent residents. As initial hypotheses, north coastal riparian forests appear to support more total bird species and more resident bird species than do the Sacramento River forests.
In comparing Harris' (1979) listing with Gaines (1980) the Falconidae, Rallidae, Hirundinidae, Strigidae, and Charadriidae are better represented in northwestern forests. Very likely, recent habitat simplification in the Central Valley is the basis for the difference. However, one ecological (but not taxonomic) group, namely oak woodland birds, appears to be under-represented in the north. Throughout the Sacramento River basin, the Plain Titmouse (Parusinornatus ), White-breasted Nuthatch (Sittacarolinensis ), Scrub Jay (Aphelocomacoerulescens ), Nuttall's Woodpecker (Picoides nuttallii ), and Acorn Woodpecker (Melanerpes formicivorus ) are common resident riparian species. Of these only the Scrub Jay and Acorn Woodpecker regularly occur in Humboldt County, and use systems other than riparian forests (Harris 1974, 1979; personal observation). A likely hypothesis to account for this is the lack of riparian valley oaks north of northern Mendocino County.
Discussion
Geofloristic History
When Axelrod (1958, 1959) postulated the Arcto-Tertiary and Madro-Tertiary Geofloras, he separated northwestern California from the Central Valley and its fringes. The former was called the Border-Redwood Forest (Axelrod 1959: 7); this association apparently dates to Pliocene age and represents a combination of floristic elements from Arcto- and Madro-Tertiary backgrounds.
As Robichaux (1980) showed, elements in the present riparian flora can be linked to fossils within both these geofloristic associations. Oregon ash and Pacific willow appear to be Arcto-Tertiary in origin and California sycamore (Platanusracemosa ) and arroyo willow (S . lasiolepis ) are more likely to have come from the southeast. Nonetheless, since the several species are all adapted to conditions near streams, they can be found intermixed to greater or lesser degree. For example, Fremont cottonwood is a Madro-Tertiary species now found along Central Valley watercourses, including those in the southeastern part of the sample area. Black cottonwood is an Arcto-Tertiary species; in the sample area, it is both coastal and higher elevation. Nonetheless, the two species overlap in the Trinity River basin at least from Douglas City to west of Weaverville. This site is in a drainage where black cottonwood are expected. Even if the present overlap were an artifact, there are dense stands of Fremont cottonwood along Cottonwood Creek, less than 33 air km. (20 air mi.) from the Trinity River stands, over a low ridge.
Axelrod (1977) discussed the range alterations induced in California vegetation by climatic change. In northwestern California there is little to impede north-south range shifts induced by changing temperature and rainfall regimes. The Franciscan Formation, the major geological substrate for the region, is faulted in a northwestward-trending direction; the north coastal rivers have followed the faultblock alignment (fig. 7). It appears that this alignment would be conducive to riparian species mixing, if species showed differential climatic responses along this north-south transect.
Substrate availability within the river channel should modify the pattern induced by climate. Narrow, rocky canyons could prove an obstacle to species requiring thick sediment deposits. Extreme channel scouring through high runoff during winter rainy periods could prevent seedling establishment and remove parent trees. Conversely, high erosion levels (typical of north coast rivers) could provide alluvial sediment deposits favoring riparian growth.
Present Communities
Given the origins shown in geological records, combined with other factors affecting
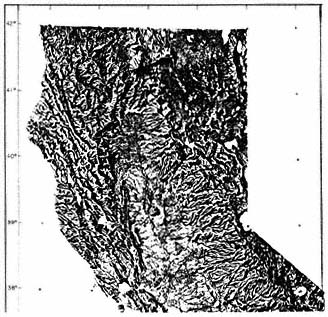
Figure 7.
Raised relief map of northern California.
Note northwest-trending mountain blocks.
Modified from Griffin and Critchfield (1972).
plant evolution in California (Raven 1977), it follows that present riparian communities reflect species' tolerances of recent geoclimatic conditions. As an hypothesis, communities along a southeast-to-northwest transect show decreasing cold (or wetness) tolerance toward the southeast end.
Riparian plant associations at opposite ends of the transect appear quite different in species composition, though there are great structural similarities. However, the changes along the transect appear gradual; species composition changes by percentages, rather than in sharp discontinuities. Both the lower Sacramento and lower Eel Rivers have forests dominated by broad-leaved deciduous trees; the forests are structurally similar but taxonomically different. Between those endpoints, tree taxa respond to local ecological conditions, and one finds conifers mixed with broad-leaved riparian species (both evergreen and deciduous). The forest composition at a particular site within the sample area depends on ecofloristic factors reflecting species ranges, and on physical conditions at the site. Hence, one expects to find an isolated Fremont cottonwood among the white alder, Oregon ash and canyon live oak in the upper Eel River drainage in Mendocino County; or sparse-foliaged Oregon ash among the oak and digger pine in Chickahominy Slough in Yolo County; or California buckeye within three kilometers of the Pacific Ocean in the lower Mattole River in Humboldt County.
It is time that biologists and conservationists interested in riparian forests investigated these northwestern sites. This holds for zoologists as well as botanists. The absence of oak forest birds from Humboldt County riparian forests suggests it may be profitable to consider the evolutionary association of birds such as Nuttall's Woodpecker and Plain Titmouse with such California endemics as the blue oak (Quercusdouglasii ) and valley oak. I am unaware of California studies of riparian small mammal communities, such as that by Geier and Best (1980) in Iowa, or those of the Lower Colorado River Project. Comparisons between the structurally similar but taxonomically distinct forests in the Sacramento Valley and the lower Eel or Klamath River basins could serve to test biogeographic habitat-diversity models. These studies should be initiated soon, however, for the north coast riparian forests, like those elsewhere, are under duress, and the situation will likely get worse rather than better.
Literature Cited
Axelrod, D.I. 1958. Evolution of the Madro-Tertiary Geoflora. Bot. Rev. 24:433–509.
Axelrod, D.I. 1959. Geological history. p. 5–9. In : P.A. Munz and D.D. Keck, A California Flora. 1681 p. University of California Press, Berkeley, Calif.
Axelrod, D.I. 1977. Outline history of California vegetation. p. 139–193. In : M.G. Barbour and J. Major (ed.). Terrestrial vegetation of California. John Wiley & Sons, New York, N.Y.
Conard, S.G., R.L. MacDonald, and R.F. Holland. 1980. Riparian vegetation and flora of the Sacramento Valley. p. 47–55. In : A. Sands (ed.). Riparian forests in California—their ecology and conservation. Institute of Ecology Pub. 15. 122 p. University of California, Davis, Calif.
Del Norte County Planning Department. 1979. Elk Creek wetland special study; Del Norte County Local Coastal Program. 51 p.
Gaines, D.A. 1980. The valley riparian forests of California; their importance to bird populations. p. 57–85. In : A. Sands (ed.). Riparian forests in California—their ecology and conservation. Institute of Ecology Pub. 15. 122 p. University of California, Davis, Calif.
Geier, A.R., and L.B. Best. 1980. Habitat selection by small mammals of riparian communities: evaluating effects of habitat alterations. J. Wildl. Manage. 44:16–24.
Griffin, J.R., and W.B. Critchfield. 1972. The distribution of forest trees in California. USDA Forest Service Research Paper PSW-82. 114 p. Pacific Southwest Forest and Range Experiment Station, Berkeley, Calif.
Harris, S.W. 1974. No title. p. II-94– II-105. In : Eureka-Arcata Regional Sewage Facility Project Environmental Impact Report. Environmental Research Consultants, Inc.
Harris, S.W. 1979. Bird narratives. In : B. Marcot (ed.), California Wildlife/Habitat Relationships Program—North Coast/Cascades Zone, Vol. 2. USDA Forest Service. Washington, D.C.
Hehnke, M., and C.P. Stone. 1979. Value of riparian vegetation to avian populations along the Sacramento River system. p. 228–235. In : R.R. Johnson and J.F. McCormick (ed.). Strategies for protection and management of floodplain wetlands and other riparian ecosystems. USDA Forest Service GTR-WO-12. Washington, D.C.
McLaughlin, J., and F. Harradine. 1965. Soils of western Humboldt County, California. 85 p. Department of Soils and Plant Nutrition, University of California, Davis, with Humboldt County, Calif.
Motroni, R.S. 1979. Avian density and composition of a riparian forest—Sacramento Valley, California. 172 p. M.S. Thesis, Sacramento State University.
Munz, P.A., and D.D. Keck. 1959. A California Flora. 1681 p. University of California Press, Berkeley, Calif.
Raven, P.H. 1977. The California flora. p. 109–137. In : M.G. Barbour and J. Major (ed.). Terrestrial vegetation of California. John Wiley & Sons, New York, N.Y.
Roberts, W.G., J.G. Howe, and J. Major. 1980. A survey of riparian forest flora and fauna in California. p. 3–19. In : A. Sands (ed.). Riparian forests in California—their ecology and conservation. Institute of Ecology Pub. 15. 122 p. University of California, Davis, Calif.
Robichaux, R. 1980. Geological history of the riparian forests of California. p. 21–34. In : A. Sands (ed.). Riparian forests in California—their ecology and conservation. Institute of Ecology Pub. 15. 122 p. University of California, Davis, Calif.
Sands, A.(ed.) 1980. Riparian forests in California—their ecology and conservation. 122 p. Institute of Ecology Pub. 15. University of California, Davis, Calif.
Classification and Dynamics of Subalpine Meadow Ecosystems in the Southern Sierra Nevada[1]
Nathan B. Benedict[2]
Abstract.—Subalpine meadow ecosystems are an important high elevation riparian vegetation type in the Sierra Nevada. The study of meadows has proceeded in two directions: classification; and studies of meadow dynamics. This paper reviews current research on these two topics in the southern Sierra Nevada.
Introduction
At high elevations in the southern Sierra Nevada, California, one of the most frequently referred to riparian systems is meadow ecosystems. Although there are other high elevation riparian systems, meadows are often chosen for study due to their visual attractiveness and importance for grazing and camping. High elevation meadows, though, are not exclusively riparian in that they can occur in any area with a sufficient amount of moisture, e.g. spring-fed meadows. This correlation between meadow ecosystems and moisture suggests that meadows play an important role in the hydrology of high elevation watersheds. As a result, a discussion of meadow classification and dynamics is vital to a thorough understanding of Sierran high elevation watersheds and riparian systems. This paper reviews current research on the classification and dynamics of southern Sierran meadow ecosystems.
Classification
Until recently, the classification of Sierran meadows was based on a simplistic scheme first suggested by Sumner[3] and subsequently followed and modified by Sharsmith[4] , Benedict (1965), and Strand (1972). The classification consists of three basic meadow types: 1) wet; 2) short-hair; and 3) woodland. Harkin and Schultz[5] proposed a topographic classification of meadows in the Rock Creek drainage, Sequoia National Park which consists of three meadow types: 1) level meadows; 2) hanging meadows; and 3) elongated stringers. More recently three meadow classifications have been presented for the southern Sierra Nevada (Benedict 1981; Benedict and Major 1980, 1981; Ratliff 1979). These three recent classifications look at meadows from three different and complementary points of view. Vegetation studies in other parts of the Sierra contain scattered descriptions of additional meadow communities and those described in the southern Sierra are discussed by Benedict (1981).
Ratliff (1979) presents a classification of meadow sites based on floristic composition. A meadow site is an area of meadow homogenous within itself and having a general species composition which is visually different from that of the adjacent areas (Ratliff ibid .). The classification was derived using various cluster analysis procedures until a final optimum classification was developed. Fourteen "site-classes" were described (table 1). Ratliff (ibid .) notes several problems with the classification. He states that the current site-classes are at least two levels above the individual meadow site. This increases the variability between sites within one site-class making it difficult to assign sites in the field to a given site-class. Another problem is that the actual number of sites in the classes is small in most cases. A total of 82 meadow sites were sampled for the classification and only 71 of over 200 species present were selected for use in the analysis proceedures.
[1] Paper presented at the California Riparian Systems Conference. [University of California, September 17–19, 1981].
[2] Nathan B. Benedict is with the Biology Department, University of Nevada, Reno, Nevada.
[3] Sumner, E.L. 1941. Special report on range management and wildlife protection in Kings Canyon National Park. Unpublished report. Sequoia National Park, Three Rivers, Calif.
[4] Sharsmith, C.W. 1959. A report on the status, changes, and ecology of back country meadows in Sequoia and Kings Canyon National Parks. Unpublished report. Sequoia National Park, Three Rivers, Calif.
[5] Harkin, D.W., and A.M. Schultz. 1967. Ecological study of meadows in Lower Rock Creek, Sequoia National Park. Unpublished report. Sequoia National Park, Three Rivers, Calif.
|
Benedict (1981) presents a classification of meadow plant communities based on floristic composition of stands. Stands are defined as homogenous units of vegetation of variable size. Sampling was done using the Braun-Blanquet releve technique (Mueller-Dombois and Ellenberg 1974). The classification was derived using tabular association analysis of the 134 stands sampled. All species present (141) were used in the analysis procedure (Benedict 1981). Three main meadow types were described on the basis of floristic composition: 1) hydric; 2) mesic; and 3) xeric (table 2). Within each of these larger units, 19 plant associations were described with more narrowly defined species composition (table 2). The plant associations are at the stand or "site" (Ratliff 1979) level thus making them readily useable in the field. The geographic distribution of these plant associations is not known at present, but is thought to include most of the southern Sierra. Other meadow plant associations remain to be described (Benedict 1981).
|
Benedict and Major (1980, 1981) present a classification of whole meadows on the basis of physiographic characteristics (table 3). Two major physiographic meadow types are described: Type I meadows with predominately vegetated margins (fig. 1), and Type II meadows with predominately sandy margins (fig. 2). Type I meadows occur in areas glaciated relatively recently and are usually surrounded by forests composed mainly of Pinus contorta subsp. murrayana . Type II meadows typically occur in areas of relatively more ancient glaciation or in areas that have not been glaciated, and are surrounded by forests composed of either pure Pinus balfouriana or a mixture of P . balfouriana and P . contorta subsp. murrayana . Both major types have a variety of subtypes distinguished by topographic position and rock type (Benedict and Major 1980, 1981; table 3). The geographic distribution of the different physiographic types is not completely
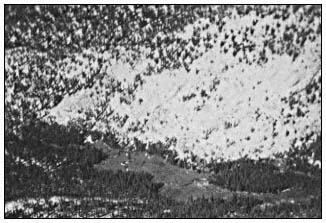
Figure l.
Rock Creek Meadow #3, Sequoia National Park. Example
of physiographic Type I subalpine meadow.
known. Type II meadows, though, are correlated with the southern boundary of mountain glaciation in the Sierra Nevada (Benedict and Major 1980, 1981).
Meadow Dynamics
The dynamic description of meadows until recently was based largely on inferences from spatial patterns and on the presence or absence of decreasers, increasers, and invaders (Sharsmith4 ). Recently Wood (1975) has described long-term changes in seven montane meadows based on the soil stratigraphy revealed in deep erosion gullies. DeBenedetti (1980) and DeBenedetti and Parsons (1979a) have been following meadow recovery after a natural wildfire in 1977. Benedict (1981) has initiated a study of the long-term development of subalpine meadows as revealed in the stratigraphy of soil cores collected in the Rock Creek drainage, Sequoia National Park, which will be directly comparable with Wood (1975).
|

Figure 2.
Siberian Outpost, Sequoia National Park. Example
of physiographic Type II subalpine meadow.
Classically meadows have been viewed as a seral stage in the hydrosere of a lake developing into a forest (Oosting 1956, and many others). Recent evidence suggests that this interpretation of meadow dynamics may be too restrictive (Benedict 1981). Two other possible hypotheses are: 1) meadow ecosystems, like any ecosystem, have changed through time as the climatic factors influencing meadows have changed; and 2) meadow ecosystems have changed the same amount or less than the surrounding forest vegetation over a given period of time. These two hypotheses are not necessarily alternatives to each other (Benedict 1981).
Evidence in support of the first hypothesis comes from three sources. Wood (1975) describes a generalized montane meadow stratigraphic sequence as: 1) a basal layer of alluvium deposited by pre-Holocene streams; 2) a paleosol dated at between 8,705 and 10,185 years B.P. developed under a mesic montane forest; 3) stratified sandy deposits dated at between 8,700 and 1,200–2,500 years B.P. and deposited under a fir, yellow pine, and lodgepole pine forest; and 4) stratified sedge peat, loams, and grus deposited since 2,500–3,000 years B.P. in a meadow environment. Based on this stratigraphic evidence, Wood (1975) suggests that meadow ecosystems can develop from, and develop to forest ecosystems, and that this is a result of climatic changes.
The second source of evidence for the first hypothesis comes from the widespread invasion of forest trees into meadows throughout the western United States (Dunwiddie 1977; Franklin etal . 1971; DeBenedetti and Parsons 1979b; Vale 1981 a,b). It has been suggested that this widespread tree invasion has resulted from excessive meadow grazing and climatic changes. It is only infrequently suggested that this is the result of successional processes. This implies that meadow ecosystems are in dynamic equilibrium with their total environment and that it is an oversimplification to view meadows only as stages in a hydrosere.
The third source of evidence that meadows are dynamically adjusted to their environment and climate comes from a man-induced experiment at Osgood Swamp near South Lake Tahoe, California (Benedict 1981). Osgood Swamp occupies a wet basin formed behind a morainal dam (Physiographic Type IA2, Adam 1967). When the morainal dam was artificially breached, the basin became drier simulating a dramatic change in climate. Subsequently, there was a massive invasion of Pinuscontorta subsp. murrayana into the meadow. This indicates that meadow vegetation changes not only as a result of successional processes but as a result of climatic and environmental changes. These changes can be either man-induced or natural.
Evidence for the second hypothesis that meadows are as stable as the surrounding forest has been discussed previously by Benedict (1981). Two sources of evidence support this hypothesis. Adam (1967) presents pollen diagrams from Osgood Swamp, and Soda Springs (near Tuolumne Meadows). These diagrams suggest that meadow vegetation is as stable or more stable than the surrounding forest vegetation. The second source of evidence comes from Wood (1975). From his stratigraphic work, seven of the meadows studied have been in existence since 1200–3000 years B.P., and two since 7700–9800 years B.P. This suggests that these meadows have been unstable over the past 10,000 radiocarbon years. In a similar manner, the forest vegetation at these same sites has also been unstable over the past 10,000 radiocarbon years (Wood 1975).
Conclusions
Meadows are variable in space and time. Spatial variation can be described from a static viewpoint and has resulted in both floristic and physiographic classifications. Temporal variation can be described from a dynamic viewpoint. Current evidence suggests that meadows have variable development patterns. The classic dynamic description of meadows as a seral stage in a hydrosere may apply to some meadows. Other meadows, though, may have developed in areas previously occupied by forest vegetation while still other meadows may be developing into forest as a result of climatic changes. Some meadows may have been in existence for the entire Holocene and are as stable or more stable than the surrounding forest vegetation. More studies are needed to determine if there are other as yet undescribed meadow development patterns.
Literature Cited
Adam, D.P. 1967. Late-Pleistocene and recent palynology in the Central Sierra Nevada, Calif. p. 275–301. In : Quaternary paleoecology. 433 p. Yale University Press, New Haven, Conn.
Beguin, C., and J. Major. 1975. Contribution a l'etude phytosociologique et ecologique des marais de la Sierra Nevada (Californie). Phytocoenologia 2:349–367.
Benedict, N.B. 1981. The vegetation and ecology of subalpine meadows of the southern Sierra Nevada, California. 128 p. Ph.D. Thesis, University of California, Davis.
Benedict, N.B., and J. Major. 1980. A physiographic classification of subalpine meadows of the Sierra Nevada, California. p. 323–336. In : Proceedings of the conference on scientific research in the national parks (2nd.). [San Francisco, Calif., November 26–30, 1979]. Volume 4: Resource analysis and mapping. 363 p. N.T.I.S., US Department of Commerce, Springfield, Va.
Benedict, N.B. and J. Major. 1981. A physiographic classification of subalpine meadows of the Sierra Nevada, California. Madrono [in press].
Bennett, P.S. 1965. An investigation of the impact of grazing on ten meadows in Sequoia and Kings Canyon National Parks. 164 p. M.S. Thesis, San Jose State College.
Burke, M. 1980. The flora and vegetation of the Rae Lakes Basin, southern Sierra Nevada: an ecological overview. 166 p. M.S. Thesis, University of California, Davis.
DeBenedetti, S.H. 1980. Establishment of vegetation following fire in a subalpine meadow of the southern Sierra Nevada: one year post-burn. p. 325–336. In : Proceedings of the conference on scientific research in the national parks (2nd). [San Francisco, Calif., November 26–30, 1979]. Volume 10: Fire ecology. 403 p. N.T.I.S., US Department of Commerce, Springfield, Va.
DeBenedetti, S.H., and D.J. Parsons. 1979a. Natural fire in subalpine meadows: a case description from the Sierra Nevada. Journal of Forestry 77:477–479.
DeBenedetti, S.H., and D.J. Parsons. 1979b. Mountain meadow management and research in Sequoia and Kings Canyon National Parks: a review and update. p. 1305–1311. In : Proceedings first conference on scientific research in the national parks. Volume 2. 1325 p. National Park Service Transactions and Proceedings. Series no. 5.
Dunwiddie, P.W. 1977. Recent tree invasion of subalpine meadows in the Wind River Mountains, Wo. Arctic and Alpine Research 9:393–399.
Franklin, J.F., W.H. Moir, G.W. Douglas, and C. Winberg. 1971. Invasion of subalpine meadows by trees in the Cascade Range, Washington and Oregon. Artic and Alpine Research 3:215–224.
Klickoff, L.G. 1965. Microenvironmental influence on vegetational pattern near timberline in the central Sierra Nevada. Ecological Monographs 35:187–211.
Mueller-Dombois, D., and H. Ellenberg. 1974. Aims and methods of vegetation ecology. 547 p. John Wiley and Sons, New York, N.Y.
Oosting, H.J. 1956. The study of plant communities: an introduction to plant ecology. 440 p. W.H. Freeman and Co., San Francisco, Calif.
Pemble, R.H. 1970. Alpine vegetation in the Sierra Nevada of California as lithosequences and in relation to local site factors. 247 p. Ph.D. Thesis, University of California, Davis.
Ratliff, R.D. 1979. Meadow sites of the Sierra Nevada, California: classification and species relationships. 288 p. Ph.D. Thesis, New Mexico State University.
Strand, S. 1972. Investigation of the relationship of pack stock to some aspects of meadow ecology for seven meadows in Kings Canyon National Park. 125 p. M.S. Thesis, San Jose State University, California.
Taylor, D.W. 1976. Ecology of the timberline vegetation of Carson Pass, Alpine County, California. 124 p. Ph.D. Thesis, University of California, Davis.
Vale, T.R. 1981a. Tree invasion of montane meadows in Oregon. American Midland Naturalist 105:61–69.
Vale, T.R. 1981b. Ages of invasive trees in Dana Meadows, Yosemite National Park, California. Madrono 28:45–47.
Wood, S.H. 1975. Holocene stratigraphy and chronology of mountain meadows, Sierra Nevada, California. 180 p. Ph.D. Thesis, California Institute of Technology, Pasadena.
Composition and Trend of Riparian Vegetation on Five Perennial Streams in Southeastern Arizona[1]
Michael G. Rucks[2]
Abstract.—Composition and trend of 78 km. (49 mi.) of riparian vegetation on five watercourses was determined. Aravaipa Creek has been excluded from cattle since 1973 and was the only study area with a dominant broadleaf riparian community and a trend towards maintaining this community.
Introduction
There are only 1119 km2 (437 mi2 ) of riparian vegetation in Arizona of which 404 km2 (158 mi2 ) are within the Gila River drainage (Babcock 1968; Minckley and Sommerfeld[3] ). It is imperative we assess the composition and trend of these remaining riparian areas.
The Gila River, San Francisco River, Bonita Creek, Mescal Creek and Aravaipa Creek were chosen for study because they are the major riparian areas on public lands administered by the USDI Bureau of Land Management's Safford District. There are also numerous smaller riparian areas associated with short perennial stream reaches and springs not discussed in this paper.
The five riverine riparian systems were studied in the summer of 1980 to establish baseline data to be used for management decisions and future monitoring.
Methods
A point-sample pace-transect with 10- to 20-pace interval (approx. 18–36.5 m. (60–120 ft.)) was used to sample the selected riparian systems.
|
A zig-zag pace route was followed to distribute sample points across the width of the riparian community from terrace to terrace. No sample points were established in the river itself.
Relative abundance of woody species for delineation of the riparian vegetation into mappable vegetation types was determined by recording the nearest woody species in a 180º -arc in front of each sample point. If the nearest woody species was not a broadleaf riparian species, the nearest broadleaf riparian species was recorded. This method maximized the amount of data collected on the broadleaf riparian species. Species recorded were designated as belonging to one of four plant communities: broadleaf riparian, riparian scrub, adjacent desert, and higher elevation. A 20-point running mean of the frequency of the members of each community was plotted to depict the mappable community at each point along the reach.
Relative abundance of herbaceous perennials was determined by recording the herbaceous perennial species closest to the sample point, expressed as a percent of the total points.
Population data on all tree species were obtained by recording the diameter-at-breast-height (DBH), or the diameter below the lowest branch of each tree recorded at each sample point. Diameters were estimated using a Biltmore scale and recorded to the nearest inch. Tree height was recorded for each tree under l-in. diameter.
Canopy coverage, density, and frequency of perennial species were determined using a line-
[1] Paper presented at the California Riparian Systems Conference. [University of California, Davis, September 17–19, 1981.]
[2] Michael G. Rucks is a Wildlife Biologist, Bureau of Land Management, Safford, Ariz.
[3] Minckley, W.L., and M.R. Sommerfeld. 1979. Resource inventory for the Gila River complex, eastern Arizona. Unpublished report to USDI Bureau of Land Management. Contract No. YA-512-CT6-2166. Arizona State University, Tempe, Ariz. XXV + 570 p.
plot method. From each 10th sample point on the pace transect, a 43.56-foot tape was stretched as close to the ground as possible in a predetermined direction roughly parallel to the channel. Canopy coverage of all woody species whose canopy intercepted a vertical plane above the tape was recorded by distance on the tape to the nearest 0.1-foot by species. Canopy coverage of a species is expressed as the percent of the total length of line.
The 43.56-foot tape also served as the center of rectangular 0.01-acre plot (435.6 ft2 ) in which species density was determined. Each plant with at least 50% of its basal area within 5 ft. of either side of the tape was tallied by species. Because of the difficulty of determining actual plant number of the sodforming Bermuda grass (Cynodon dactylon ), density of this species was recorded as one (1) when it was present in the plot.
Frequency of a species is expressed as the percent of 0.01-acre plots in which it occurs.
An importance value was determined for each woody species. This value incorporates three parameters of a species in relation to its community. Relative coverage (size or biomass), relative density (number), and relative frequency (ubiquitousness) of each species are combined in one value for comparative purposes. The importance value is determined as:
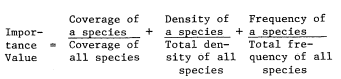
The maximum importance value of a species would be 300 if it were the only species present.
Utilization of a plant species by browsing wildlife or domestic livestock was recorded at each sample point.
The following is a summary of the amount of line intercept and number of 0.01-acre plots recorded on each riverine riparian system.
|
Results and Discussion
Table 1 lists perennial species recorded in the riparian inventory described in this paper. Plant common names are from the USDA Soil Conservation Service National Plant List (May 1980).
|
|
Community Classification of Woody Species
Occurrence on each system studied and the assignment of woody species to one of four plant communities (broadleaf riparian, riparian scrub, adjacent desert, higher elevation) is also shown in table 1.
Figures 1, 2, 3, 4, and 5 depict the relative abundance of the woody species communities in each
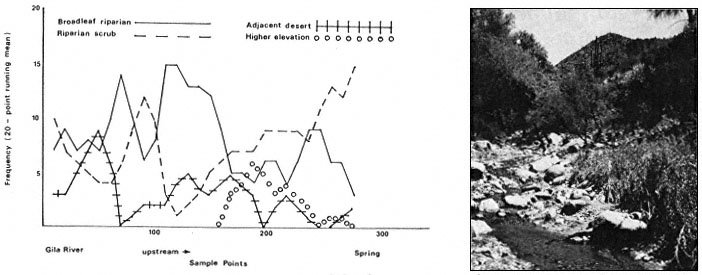
Figure l.
Community classification of woody species and photograph of Mescal Creek.
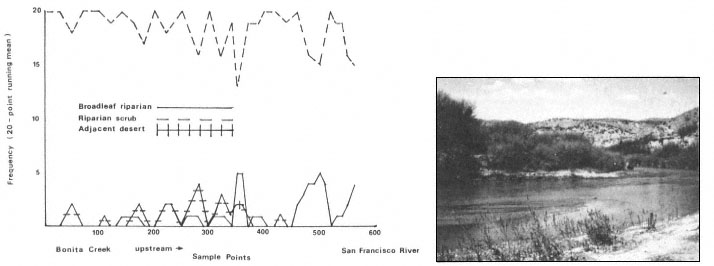
Figure 2.
Community classification of woody species and photograph of the Gila River.

Figure 3.
Community classification of woody species and photograph of the San Francisco River.
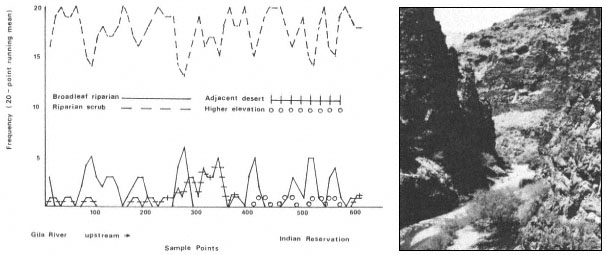
Figure 4.
Community classification of woody species and photograph of Bonita Creek.

Figure 5.
Community classification of woody species and photograph of Aravaipa Creek.
riparian system, expressed as a running mean of the woody species per 20 sample points. Also included are photographs of the watercourses. A good distribution of mature broadleaf riparian trees without successful reproduction in the open areas made it more likely for riparian scrub, adjacent desert, or higher elevation species to be the nearest woody species to the sample point. Mescal Creek was found to be an example of fluctuation in community type caused by seedling absence. The Gila River and San Francisco River showed a very clear dominance of the riparian scrub community type. Bonita Creek also showed a riparian scrub community type with a slightly greater broadleaf riparian component.
Aravaipa and Mescal Creeks were the only systems with a dominant broadleaf riparian component. No apparent correlation appeared to exist between broadleaf riparian dominance and channel or terrace width. Broad areas on the watercourse might be expected to correspond to broadleaf riparian dominance, but this was not always the case.
Since no broadleaf riparian species was recorded if one was not present before the next sample point, the percent of sample points on each watercourse where broadleaf riparian species were recorded illustrates the ubiquity of these species. Broadleaf riparian species were recorded at the following percentages of sample points on the watercourses: Aravaipa Creek 99.5%; Mescal Creek 84.9%; Bonita Creek 73.1%; Gila River 22.5%; San Francisco River 21.1%.
Relative Abundance of Woody Species
Table 2 shows the relative abundance of woody species occurring at a frequency over 1.0%. Aravaipa Creek had a high percentage of broadleaf riparian species as would be expected from the community classification in figure 1. The San Francisco and Gila Rivers had a very high percentage of riparian scrub species. Salt cedar, burro brush, mesquite and seep willow account for 90% of the woody species recorded on the San Francisco River.
Relative Abundance of Herbaceous Perennials
Table 3 shows the relative abundance of the principal species of herbaceous perennials recorded. Bermuda grass is clearly the dominant herbaceous perennial on each of the watercourses. All the herbaceous perennials in table 3 are grazing-resistant with the exception of bent grass, a minor component of Bonita Creek.
After seven years of cattle exclusion on Aravaipa Creek, palatable grasses other than bermuda grass still occurred at negligible frequencies. Trend plot photos for Aravaipa Creek show bermuda grass in sparse clumps extending to form a solid mat after the cattle were removed. This extensive sod formation by bermuda grass has apparently limited establishment of other grasses.
|
|
|
Population Data
Figures 6–9 depict the size-class data for broadleaf riparian trees and mesquite on the five watercourses.
Aravaipa Creek
Aravaipa Creek (fig. 6) has been excluded from cattle since 1973 and shows a high percentage of seedlings in every species population. The survival of seedlings to the 1- to 3-inch size-class is good for the broadleaf trees with the exception of sycamore and walnut. Sycamore seed production and establishment is sporadic and sycamores often rely on sucker sprouts for reproduction. It would require a follow-up study to determine the number of sycamore seedlings surviving to the 1- to 3-inch size-class. The absence of walnuts in the 1- to 3-inch size-class may be due more to the small walnut sample (31 trees) than to ecological factors.

Figure 6.
Size-class data for Aravaipa Creek (DBH size-classes in inches).
Gila and San Francisco Rivers
The Gila River and San Francisco River (fig. 7 and 8) show virtually no successful broadleaf riparian reproduction. The only trees in the 1-to 3-inch size-class are cottonwoods on the Gila River, and they comprise only 2% of the cottonwood sample. The low percentage or absence of 1-to 3-inch size-class trees indicates low seedling survival. On the San Francisco River, 82% of the cottonwood seedlings were browsed by cattle. On the Gila River, 62% of the cottonwood and willow seedlings were browsed, resulting in seedlings 6–10 in. tall with up to 0.6 in. diameters indicating the seedlings had been browsed for more than one growing season. Mesquite, however, is reproducing successfully on both of these rivers.
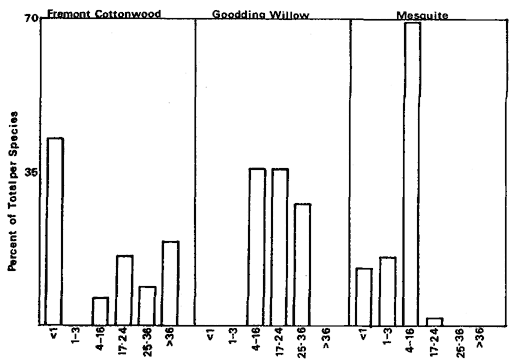
Figure 7.
Size-class data for the San Francisco
River (DBH size-classes in inches).
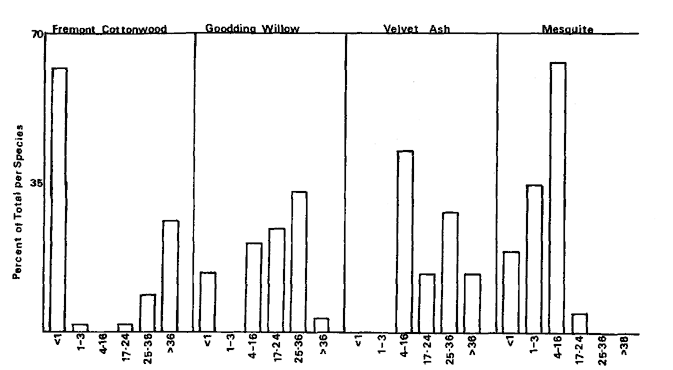
Figure 8.
Size-class data for the Gila River (DBH size-classes in inches).
Very poor broadleaf riparian tree establishment and successful mesquite establishment will eventually lead to the replacement of the broadleaf riparian community by mesquite and other riparian scrub species. This trend is already well established on the Gila and San Francisco Rivers (figs. 2, 3, 7, and 8).
Mescal Creek
All the broadleaf species on Mescal Creek (fig. 9) showed good representation in the seedling size-class. However, willows, sycamore, and walnut showed a total absence of 1- to 3-inch size-class trees. Cottonwood had 1.5% in this size-class and ash 5.5%. This strongly indicates seedlings were not surviving. Cottonwood seedlings were 70% browsed by cattle, willows 9%, sycamores 62%, ash 1%, and no walnut seedlings were recorded as browsed. Mesquite is reproducing successfully on Mescal Creek.
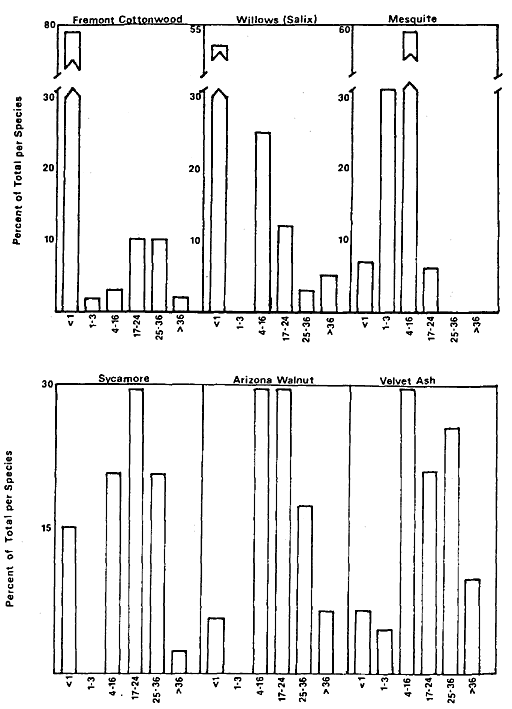
Figure 9.
Size-class data for Mescal Creek
(DBH size-classes in inches).
Poor broadleaf riparian establishment and successful mesquite establishment will eventualy lead to the replacement of the broadleaf riparian community on Mescal Creek. A good distribution of mature broadleaf riparian trees on Mescal Creek results in a dominant broadleaf riparian community (fig. 1). However, poor reproduction will not maintain this broadleaf riparian community over time.
Bonita Creek
Data from Bonita Creek (fig. 9) indicated poor reproductive success of all broadleaf riparian species:
1) only 4% of the cottonwoods were in the seedling size-class and all of these seedlings were browsed by cattle. The 1- to 3-inch size-class represented only 2% of the sample;
2) only 1.5% of the willows were in the seedling size-class and 67% of these were browsed. No 1- to 3-inch size-class willows were recorded;
3) only 1.5% of the sycamores and no velvet ash were in the seedling or 1- to 3-inch sizeclass.
Grazing has occurred on Bonita Creek for over 100 years. The palatability of broadleaf riparian seedlings in descending order of
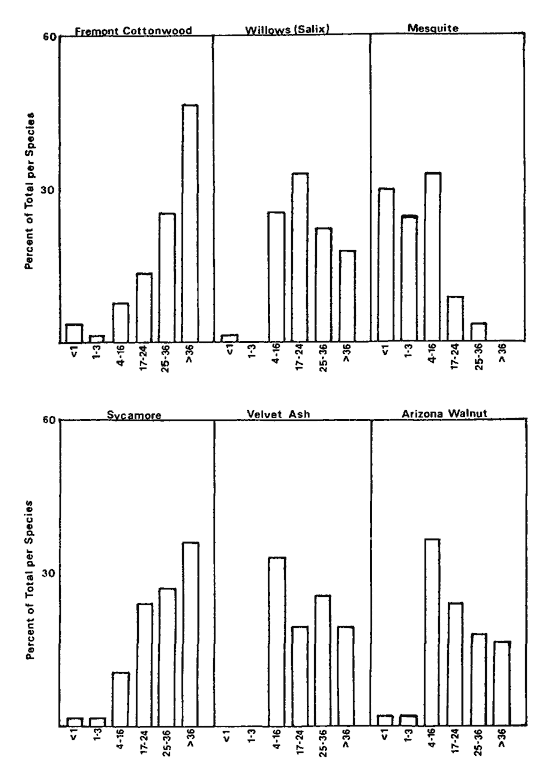
Figure 10.
Size-class data for Bonita Creek
(DBH size-classes in inches).
preference is: cottonwood, willow, sycamore, ash, and walnut.[4] Prior to 1972, grazing pressure on Bonita Creek was perhaps severe enough to affect the most palatable species, but not severe enough to affect ash and walnut. Since 1972, grazing pressure has apparently increased sufficiently to affect the less palatable ash and walnut seedlings.
Sycamore, willow, and cottonwood populations indicate a size-class distribution the reverse of one needed to maintain these species in the community.
Arizona walnut and velvet ash are slow growing species. On Bonita Creek, size-classes for these trees show a normal size-class distribution from 4- to 16-in. and larger. This indicates successful seedling reproduction prior to about 8–10 years ago to establish the 4- to 16-in. trees. The grazing allotment history on Bonita Creek indicates a change in grazing allottees in 1972. The change in grazing allottees corresponds to the downward trend in successful reproduction of walnut and ash.
The trend on Bonita Creek is toward replacement of the broadleaf riparian community by mesquite and other riparian scrub species. This trend is already well established as illustrated in fig. 4 (community classification).
Summary
Aravaipa Creek is the only system where the trend is not toward replacement of the broadleaf riparian community by riparian scrub. Aravaipa is also the only system where cattle have been excluded.
Flooding
Flooding is another factor that influences reproductive success. In the winter of 1978–79, all five watercourses experienced severe floods. Mesquite is not damaged by cattle browsing, but is as susceptible to flooding as the broadleaf species. In each of the systems, mesquite indicated successful reproduction. Mesquite trees were recorded in all parts of the riparian systems from high on the upper banks to within feet of the water. Mesquite seedlings and 1- to 3-inch trees were found under mature broadleaf riparian species and in habitats suitable for broadleaf riparian reproduction.
Aravaipa Creek experienced severe flooding, yet did not indicate poor broadleaf riparian reproduction. Flooding does have adverse effects on tree seedlings, but is not, apparently, as detrimental to the broadleaf riparian communities as cattle browsing upon seedlings.
Importance Values
Table 4 shows the importance values for the major woody species on the five systems. An analysis of these data confirm the inferences drawn from the population analysis.
Aravaipa Creek
High coverage, density, and frequency of the cottonwoods indicate a mature population evenly distributed along the river with very successful reproduction. High coverage indicates large mature trees. High frequency indicates uniform distribution and high density indicates numerous seedlings and small trees. Walnut is the only broadleaf riparian tree with a low importance value, but Aravaipa Creek is the only system in the study where walnut is recorded in the 0.01-acre samples. High density relative to frequency of seep willow indicates clump-like concentrations. Nearly equal density and frequency of burro brush indicates a fairly uniform linear distribution.
[4] Steve Bingham. Personal communication.
|
San Francisco River
The two broadleaf riparian species recorded on the San Francisco River have very low importance values compared to the riparian scrub species. High coverage and low but fairly equal density and frequency of the cottonwoods indicate large mature trees growing singly and sparsely along the river with very few seedlings. Low coverage of willows with none recorded in the
0.01-acre samples indicate very sparse distribution of mature trees with no successful reproduction. Very high coverage of mesquite and nearly equal, but high frequency and density indicate mature mesquite and successful reproduction. Extremely high density of salt cedar relative to its coverage indicates an abundance of young plants. The frequency is also high indicating uniform distribution and strongly suggesting salt cedar will become dominant.
Gila River
High coverage of cottonwood relative to its frequency indicates large mature trees sparsely distributed along the river. Low density of cottonwoods indicates few seedlings. Willow was recorded in only one of the 57 0.01-acre samples and this was a browsed seedling. Very high coverage of mesquite and nearly equal, but high frequency and density indicate mature mesquite and successful reproduction.
Mescal Creek
Data from Mescal Creek show a predominantly broadleaf riparian community (fig. 1). The very high coverage of ash relative to its density indicates large mature trees without successful reproduction. The low frequency value indicates these large trees are sparsely spread along the creek. The density of cottonwoods relative to frequency indicates good tree establishment. The coverage value for cottonwood is not disproportionately high indicating seedlings are fairly numerous. However, coverage, density, and frequency of cottonwoods on Mescal Creek are much lower than cottonwoods on Aravaipa Creek. Successful reproduction and establishment of the broadleaf riparian community on Mescal Creek is apparently less than on Aravaipa Creek.
Bonita Creek
Very high coverage of sycamore relative to its density and frequency indicates large mature trees distributed sparsely along the creek with very few seedlings. Sycamore was third in importance only because of its relatively high coverage. Riparian scrub species are clearly dominant. Willows, cottonwood, walnut, and ash all have low density compared to their coverage indicating a mature broadleaf riparian vegetation which is not successfully maintaining itself.
General Conclusions
Aravaipa Creek is the only system of the five studied with a dominant broadleaf riparian community and successful reproduction. Mescal Creek is also dominated by a broadleaf riparian community, but has poor reproductive success. The Gila River, San Francisco River, and Bonita Creek all show a well established trend toward replacement of the broadleaf riparian community by riparian scrub.
Cattle browsing appears to be the major contributing factor to the downward trend of broadleaf riparian communities. The only system in this study with an upward trend in the broadleaf riparian community is Aravaipa Creek, where cattle have been excluded since 1973.
Summary
Riparian vegetation along five perennial watercourses in the USDI Bureau of Land Management's Safford District was studied. Community classification of woody species, relative abundance of woody and herbaceous perennial species, and size-class data were recorded using a pace-transect. Coverage, density, and frequency were determined for each species.
These data were analyzed to determine condition and trend of the five riparian vegetation communities. Aravaipa Creek, where cattle have been excluded since 1973, was the only system with a dominant broadleaf riparian community and successful reproduction.
Acknowledgements
I appreciate the guidance of Steve Bingham, Botanist, Eastern Arizona College, Thatcher, Arizona, for the design of this study and identification of plant specimens. I would also like to thank Cindy French for her assistance with the field work.
Literature Cited
Babcock, H.M. 1978. The phreatophyte problem in Arizona. Arizona Watershed Symposium, Proceedings 12:34–36.
USDA Soil Conservation Service. 1980. National Plant List (May, 1980). USDA Soil Conservation Service. Washington, D.C.