Lithic Ejecta: An Important Geothermal Prospecting Tool
Lithic ejecta can provide information about the (a) subvolcanic stratigraphy, (b) aquifer depth and physical properties, (c) aerial extent of the fluid reservoir in a volcanic region, and (d) chemical and thermal regime of rocks at depth. An in-depth study (for example, Barberi et al ., 1988) involves the collection of tephra samples, petrographic microscopic point counts of lithic constituent abundances, comparisons of the abundances with the stratigraphic position in both the tephra deposit and the regional rocks, and x-ray analyses of alteration assemblages found in lithic fragments.
Figure 2.44 schematically illustrates a hydrovolcanic eruption upward through a varied stratigraphic section that consists of three principal rock units. The interaction of water and magma occurs in aquifer rock unit 2, and subsequent eruptions excavate rock unit 1. Through analysis of the resulting tephra deposit, depicted in Fig. 2.45, the basement stratigraphy can be reconstructed. In this case—an eruption sequence that becomes wetter with time—the lowest tephra units contain mostly lithic fragments from the upper rock unit (unit 1) because it is excavated as the crater is formed. Later magmatic pumice-fall units might contain a small percentage of lithic fragments (< 10% by volume); lithic fragments from the deepest unit (unit 3) are most abundant because that unit is fractured by magma intrusion. When the hydrovolcanic dry eruption begins, lithic abundances increase by 5 to 20 vol%; those from the aquifer unit (unit 2) nearly equal those from the other two stratigraphic units combined. Final, wet eruptions produce the greatest abundance of lithic fragments (10 to 50 vol%), most of which are from the aquifer unit.
A good example of the correlation between basement stratigraphy and lithic fragments comes from geothermal investigations on the island of Nisyros, Greece (Barberi, 1985). Figure 2.46 shows the eruptive sequence and a correlation between its lithic constituents and the basement lithology encountered in a geothermal well. The hydromagmatic phases of the eruption produced dune-bedded pyroclastic surge deposits in which lithic fragments came from deep, permeable rock units, whereas the magmatic pumice fall units have lithic fragments from units higher in the basement stratigraphy in which little permeability was found. In this case, the hydroclastic tephra provide evidence of permeable rock units at depth. In addition, the lithic constituents of the hydroclastic units show alteration mineral assemblages that indicate elevated temperatures existed in the permeable strata.
The thermal regime of basement rocks is also reflected by lithic constituents found in pyroclastic strata. By careful study of the paragenesis of alteration minerals in lithic fragments, including stable isotope variations (for example, 18 O and 13 C) and fluid inclusion analysis (such as Cl-, SO4 , B, NH4 , and SiO2 ), it is possible to surmise not only the geochemical nature of hydrothermal fluids at depth but also their evolution with
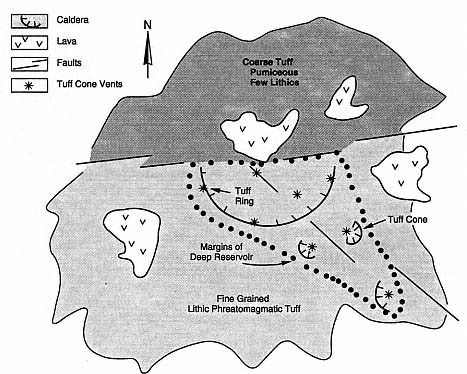
Fig. 2.42
Hydrothermal reservoir geometry (dotted line) inferred from a geological map showing
areas underlain by dry (pumiceous) and wet (phreatomagmatic) volcanic products.
A pyroclastic flow has been erupted from a caldera; it is pumiceous in its northern
regions but lithic-rich and fine-grained in its southern portions. Tuff ring and tuff
cone vents exist within the region underlain by the hydrothermal reservoir, whereas
lava flow vents are outside that region. The caldera lies at the intersection of two fault
systems. The east-west fault system marks the northern boundary of the hydrothermal
reservoir, presumably as an aquitard, and the southeast-northwest-trending fault
apparently localizes the aquifer along its southern extent. Because the caldera straddles
the northern boundary of aquifer rocks, eruptions from its northern side are dry,
whereas those from its southern side are of a phreatomagmatic character.
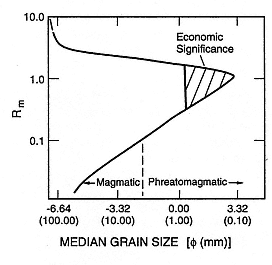
Fig. 2.43
Hypothetical water:magma mass ratio (Rm ) as
a function of the near-vent median grain size of
pyroclastic rocks. Where median grain sizes of
tephra are finest (between 0.1 and 1.0 mm),
the inferred water: magma mass ratio is
between 0.3 and 1.0. In this range of Rm ,
the hydrothermal potential—and therefore
the economic significance—is greatest.
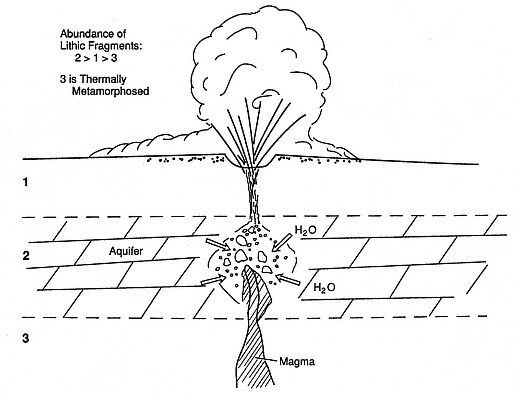
Fig. 2.44
Sketch of a hydromagmatic eruption through basement stratigraphy consisting of three rock types:
1 and 3 are of arbitrary lithology, and rock 2, a limestone aquifer where the water/magma interaction
occurs, is thermally metamorphosed near the volcanic conduit. The abundance of lithic fragments
carried within the ejecta are shown by rock type. Type 2 is most abundant, because of the
explosive fragmentation that occurs in the aquifer. Lithic type 1 is greater than lithic type 3
because it represents near-surface rocks eroded from the conduit walls by
the pressurized mixture of steam and tephra. Lithic type 3 is least abundant
because its deep lithology is little affected by the erosive power of upward migrating magma.
time and thermal regime. In the example from Nisyros (Fig. 2.46), the four zones of secondary minerals found in the geothermal well include—with increasing depth—argillic, argillic-phyllitic, phyllitic-propylitic, and propylitic mineral assemblages, the lowest three of which show up in lithic fragments from the tephra deposit. Figure 2.47 reviews basic hydrothermal alteration facies and equilibrium temperatures represented by the mineral assemblages that characterize each facies. Secondary mineral assemblages in volcaniclastic rocks are also good indicators of burial depth (Viereck et al ., 1982). Glass shards commonly alter during diagenesis to zeolites, feldspars, opal-ct, and quartz as well as smectite clays and consequently can indicate temperature and burial depth (Fig. 2.48).
Excellent examples of the use of hydrovolcanic tephra and lithic constituents in exploration for geothermal reservoirs are provided by studies in the Latium volcanic province of Italy. This area (shown in Fig. 2.49) includes the Vulsini (Latera) volcanic complex, Vico volcano, the Sabatini volcanic complex, and the Albani volcanic complex, all of which exhibit important hydrovolcanic features (De Rita et al ., 1983).
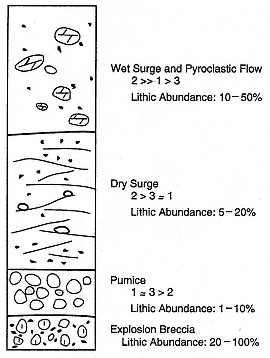
Fig. 2.45
An idealized stratigraphic section produced by
the eruption illustrated in Fig. 2.44. The basement
stratigraphy is represented by lithic fragment
abundances that are sensitive to eruption
sequence. Initial, dry pumice eruptions promote
nearly equal abundances of types 1 and 3 as
a result of vent widening and the relatively
large area of contact between the magma
and type 3. Conduit pressure is too great to
allow much cavitation of rock type 2. As
eruptions become hydromagmatic, the
abundance of type 2 increases significantly.
Funiciello et al . (1976) and Funiciello and Parotto (1978) described the correlation between sedimentary lithic ejecta in pyroclastic deposits of the Albani and Sabatini regions. Figure 2.50 is a sketch geologic map of the Alban Hills south of Rome. The dominant feature of this area is the Tuscolano-Artemisio caldera and the distribution of its phreatomagmatic ejecta, which is mainly to the west of the caldera. In surrounding areas, the sedimentary basement rock, consisting of Mesozoic to Cenozoic marine rocks, is exposed and is also represented by lithic fragments in the tephra of the volcanic field. The abundance of these lithic fragments and the locations of hydrovolcanic vents allowed Funiciello and Parotto (1978) to reconstruct the substrate below the volcanic complex (Fig. 2.51). The hydrovolcanic vents are located above a structurally high block of water-saturated continental shelf and basin rocks. North-northwest faults crossing this block have contributed to fracture permeability.
A second example from Italy is the Sabatini/Cesano region described by Funiciello et al . (1976). In the eastern part of the volcanic field, the authors were able to distinguish both shallow and deep aquifers. In shallow interactions, hydrovolcanic vents were monogenetic maar craters with a diameter of 1 km or less, whereas deep interactions produced poorly defined maar structures and complex caldera structures. These caldera structures appear to represent the coalescence of several maar vents that eventually collapsed together to form a single caldera. An example of this sequence of events is the Baccano caldera, whose walls expose hydrovolcanic tephra from numerous vents (see also Fig. 4.21). Fine tephra, exposed in ridges of phreatomagmatic tephra agglomerates, have a high chloride and sulfate content that is inherited from the deep hydrothermal reservoir fluids involved in water/magma interaction. In addition, ejecta analysis by scanning electron microscopy and energy dispersive x-ray
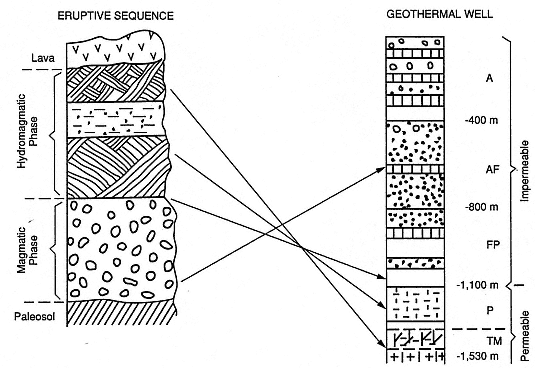
Fig. 2.46
An example of lithic stratigraphy determined by correlations with a geothermal well in Nisyros,
Greece. The eruptive sequence shown is a magmatic-phase pumice fallout on a paleosol
substrate that is overlain by dune- and massive-bedded hydromagmatic tephra capped
by a lava. Lithic fragments from the magmatic-phase deposits correspond to impermeable strata
that are logged in the geothermal well, whereas the lithic fragments in the hydromagmatic-phase
deposits correspond to deeper, increasingly permeable strata. Hydrothermal alteration observed in
the geothermal well increases downward from argillic (A) through argillic-phyllitic (AF),
phyllitic-propylitic (FP), and propylitic (P) to thermally metamorphosed rock (TM).
(Adapted from Barberi, 1985.)
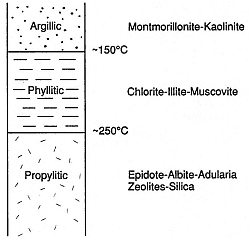
Fig. 2.47
Review of hydrothermal alteration facies
and characteristic mineral assemblages shown
as a function of depth and temperature.
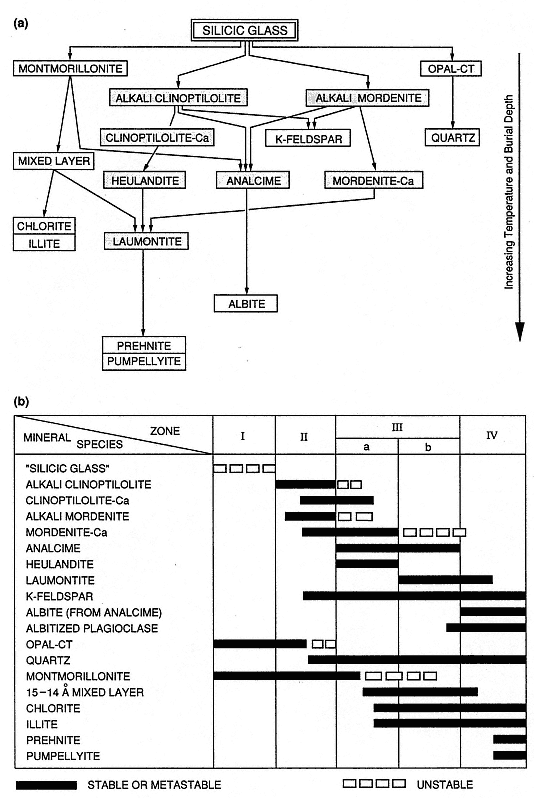
Fig. 2.48
Burial diagenesis of zeolites and clays. (a) This flow diagram shows the development of authigenic
zeolites (shaded boxes) and silicates from silicic glass during burial diagenesis and metamorphism.
(b) In this chart of mineral assemblages in a thick section of marine silicic volcaniclastic rocks,
zones I to IV indicate increasing burial depth. Zone I is characterized by partial alteration of silicic
glass to montmorillonite and opal-A/opal-CT. Zone II shows additions of alkali zeolites formed by
reaction of silicic glass with interstitial water. The transition to Zone III (where alkali zeolites are
transformed into analcime, heulandite, and laumontite) occurs at temperatures of 84 to 91°C.
The transition to Zone IV (analcime transformed to albite) occurs at temperatures of
120 to 124°C and marks a gradation into the thermal metamorphic regime.
(Adapted from lijima, 1978.)
analysis allowed Funiciello et al . to classify secondary mineral paragenesis and determine the most recent temperature and chemistry of deep reservoir fluids involved in the hydrovolcanic eruptions. Their schematic geologic map (Fig. 2.52) was created from lithic ejecta analyses, which show excellent agreement with geophysical studies of regional gravity, electrical resistivity, and heat flow (Fig. 2.53).
The Funiciello et al . (1976) study constitutes a major step toward the application of volcanology to geothermal prospecting. They stated that
"As a first approximation, we assume that the surface covered by products of recent phreatomagmatism, rich in ejecta from the deep sedimentary basement, delimits the minimum dimensions of a potential geothermal field. As a second approximation, the study of the sedimentary ejecta allows [us] to reconstruct the stratigraphic and structural characters of the sector where the phreatomagmatism occurred. This [study] makes it possible to interpret the paleogeographic and tectonic evolution of the area and to fit it within the regional geology."
With respect to thermal regimes, Funiciello et al . (1976) added:
"Furthermore, if a sequence of phreatomagmatic products in several layers is available, the comparative investigation of the mineralizations in the different layers supplies indicators on the evolution of the hydrothermal field in the time."