10—
RIPARIAN RESTORATION
PROBLEMS AND OPPORTUNITIES
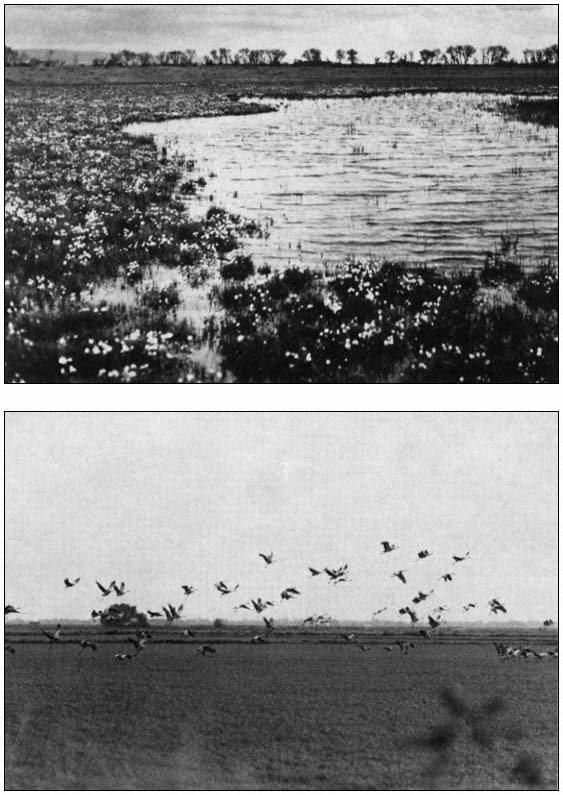
Mortality and Growth of Cottonwood on Dredge-Spoil[1]
Bertin W. Anderson, John Disano, Donald L. Brooks, and Robert D. Ohmart[2]
Abstract.—Efforts to reintroduce cottonwood (Populusfremontii ) trees can be expected to be highly successful at minimal costs. Trees grew 3-5 m. annually and survival approached 100% when planting was accompanied by tillage to a depth of 3 m. in sandy soil. Irrigation for five months during the year of planting was adequate for maximum growth and survival.
Introduction
Cottonwood (Populusfremontii ) trees were formerly a dominant riparian species along the lower Colorado River (Ohmart etal . 1977). Their demise has paralleled construction of major dams along the river and the encroachment of salt cedar (Tamarixchinensis ). Dam construction brought an end to the annual flooding which was necessary to enrich and moisten the soil in which cottonwood seeds could germinate. The annual floods also flushed out litter; incidence of fires has increased with litter accumulation. Hot fires kill cottonwood but not salt cedar. The latter species therefore benefits from hot fires. Cottonwood seed germination has been reduced and hot fires have killed many of the mature trees. Cessation of floods combined with dredging has lowered the water table; mature trees which once thrived with high water tables and flooding are now growing over layers of fine soil, left "perched" high and dry as the water table has receded.
In January 1979 we began planting Fremont cottonwood and other species of native riparian trees (Anderson and Ohmart 1981) on a 30-ha. dredge-spoil site. The site is located next to the Colorado River about 8 km. southeast of Palo Verde, Imperial County, California. One objective was to learn as much as possible about the growth of cottonwoods under as wide a variety of environmental conditions as logistically possible on the 30-ha. plot. Another objective was to determine the economic feasibility of reintroducing cottonwoods along the Colorado River.
Methods
A total of 607 native Fremont cottonwood tree cuttings were started in a nursery. Cutting lengths were about 0.3 m. and diameters were about 1.3 cm. All propagation materials came from trees growing locally to assure the greatest preadaptation to the planting site. Rooted cuttings were planted on the site beginning in late January and extending through February 1979. At planting the trees consisted of one or two shoots about 0.6 m. tall and not over 1.3 cm. in diameter. All young trees were given about 85 gm. of time-release fertilizer at the time of planting.
Tillage
Tillage was accomplished by augering holes to various depths to destroy the soil profile. Holes 20 cm. in diameter augered to 1.2 m. were provided for 125 young trees; an additional 340 holes 30 cm. in diameter were augered 3 m. deep. As controls, 142 trees were planted without any deep tillage. Holes 3 m. deep and 5 cm. in diameter were dug for these trees just prior to the second growing season. Deep tillage facilitates root and water penetration to the water table. The water table was 3–4 m. beneath the soil surface.
Irrigation
All trees were supplied with 121 l. of water each day for a minimum of 150 days. Water was then discontinued for 30 trees; every 15 days thereafter water was terminated for 30 more trees until November 1980, at which time irrigation ceased. In March 1980 we randomly selected 161 trees which we watered for an additional eight months.
[1] Paper presented at the California Riparian Systems Conference. [University of California, Davis, September 17–19, 1981].
[2] Bertin W. Anderson is Faculty Research Associate, Center for Environmental Studies, Arizona State University, Tempe, Ariz. John Disano is Research Biologist, Center for Environmental Studies, Arizona State University, Tempe, Ariz. Donald L. Brooks is Field Biologist, Center for Environmental Studies, Arizona State University, Tempe, Ariz. Robert D. Ohmart is Associate Director, Center for Environmental Studies, Arizona State University, Tempe, Ariz.
Soil Type
The soil varied from sand to sandy loam to a depth of 3 m. to 3.7 m. with narrow clay layers at 0.9 m. and 1.5 m. below the surface. One hundred fifty-three trees were planted in the heavier soils, and the remainder were planted in sandy soil.
Competition from Other Plants
Shortly after drip irrigation began, Bermuda grass (Cynodondactylon ), Russian thistle (Salsolaiberica ), and smotherweed (Bassia hyssopifolia ) germinated at various planting sites (almost exclusively in the areas with heavier soils). The potential for competition from other vegetation thus became an unanticipated additional variable.
Measurements
Trees were measured in May, late August, and late November 1979; each period included about 90 days of growth. The next measurements were taken in June 1980, after about 210 days of growth. Final measurements were made about 90 days later in October 1980. Measurements included the height of the tree from ground to tip. Crown measurements were the average of the north-south and east-west diameters. An index to total biomass was calculated as:

Analytical Methods
The mean growth for trees with varying deep tillage was compared using a two-tailed z-test. Interrelationships between variables were determined with Pearson product-moment correlations. The respective and collective contributions of environmental effects on growth were assessed with stepwise multiple linear regression analysis. In all cases, the accepted level of statistical significance was P £ 0.05.
Sample sizes of trees grown under the various tillage depths, irrigation periods, soil types, and degrees of competition are presented in table 1.
|
Results
Intercorrelations between Growth Variables
All growth variables were highly intercorrelated (table 2). For this reason we present graphic and tabular material for height data only. Mention of other variables is included only where it is particularly significant or facilitates interpretation. Approximations for the other growth variables can be estimated from the equations in table 2. During the 630 days, over two growing seasons, the trees grew at an average rate of more than 0.007 m. per day (fig. 1). The fastest growth rate occurred during GP3 (August–October 1979), when the trees grew an average of 0.014 m. per day. The slowest growth rate occurred during the 90 days immediately after planting; the next slowest growth rate was during GP4 (October 1979–July 1980). This was expected, since these periods included the winter
|
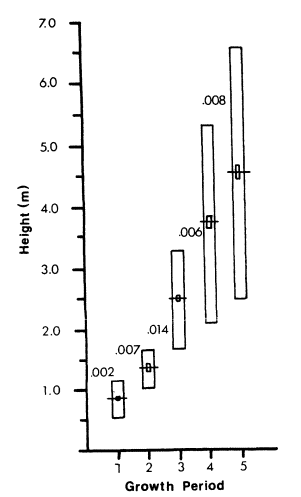
Figure l.
Mean heights of Fremont cottonwood trees planted
on dredge-spoil. Mean growth in m./growing period
(GP) indicated by three-digit numbers (1 m. = 3.28 ft.);
horizontal line represents the mean; large rectangle,
one standard deviation; small rectangle, two standard
errors of the mean. The measurements for the growing
periods were May, August, and October 1979, and
July and October 1980 for GP 1–5, respectively.
months when trees were dormant. While height of trees from GP1 through GP5 increased about 600%, biomass index increased by more than 1600% in the same period. Estimates of growth are conservative relative to the maximum possible growth rates, because some combinations of tillage and irrigation proved to be greater stimulators of growth than other combinations.
Effect of Environmental Variables on Growth
Extent of deep tillage was the overwhelmingly most important environmental variable tested (table 3). Effect of deep tillage, although significantly correlated with growth in GP1 and GP2, did not really become apparent until GP3. This is reasonable because the effect of breaking up the soil profile to a depth of 3 m. does not become important until tree roots begin to penetrate more deeply, a function of growth and age. None of the other environmental variables explained more than 3% of the variance.
Collectively, the environmental variables had little effect until GP3, when they accounted for 49% of the variance in tree growth. By GP5, the environmental variables accounted for 67%. The rest of the variance is presumably due to error introduced with measuring.
|
Effect of Deep Tillage on Growth
The dramatic effect of tillage to 1.2 m. and 3 m. is shown in figure 2. With no soil tillage, the variance (4.41) is greater than with tillage to 1.2 m. (2.07) or 3 m. (1.44). Although trees initially planted without deep tillage, but which received deep tillage at the beginning of the second growing season, did not attain a significantly greater height than the controls, the variance (0.49) was far less (all trees were growing) than for those without augered holes (some trees were growing and some were not). The effect of deep tillage (fig. 3) demonstrates that trees with tillage to 3 m. began to grow much faster during GP3 than did the remainder. During GP3 trees with tillage to 1.2 m. quickly exceeded the height of those with no augered holes but still did not grow as rapidly as those with tillage to 3 m. The biomass index at the end of GP5 was 32.3, 4.5, 1.2, and 0.9 for trees with tillage to 3 m., 1.2 m., 3 m. one year after planting, and no tillage, respectively. Those tilled a year after planting almost immediately began to grow faster than those with no tillage.
Effect of Deep Tillage on Survival
Effect of deep tillage on survival through GP5 (table 4) was as dramatic as for growth. With no tillage, 43% of the trees died. With tillage to 1.2 m., mortality decreased to 12%; there was no mortality of trees with deep tillage. Even among trees for which tillage to 3 m. was delayed until a year after planting, none died. Thus for 420 trees with tillage to 3 m., survival was 100%.
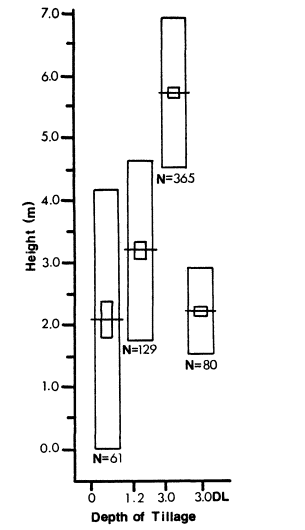
Figure 2.
Mean height after two growing seasons of Fremont+
cottonwood trees with tillage to various depths planted
on dredge-spoil. Abbreviations and symbols as in
figure 1. DL = tillage to 3 m. provided at beginning of
second year.
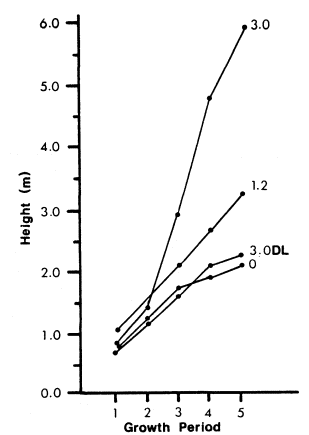
Figure 3.
Mean height for five growing periods with tillage
to various depths, for Fremont cottonwood trees
planted on dredge-spoil. The first three growing
periods are for 1979 and the last two for 1980.
Abbreviations and symbols as in figures 1 and 2.
Effect of Irrigation on Growth
Irrigating for different periods of time had little effect on growth (table 5). Trees with tillage to 3 m. that were watered for 165 days were significantly taller than those watered for 150 days. However, it is unlikely that this observation was biologically meaningful because none of the other irrigation periods produced trees of significantly greater height than those which were irrigated for the minimum amount of time.
Effect of Irrigation on Survival
For trees with tillage to 3 m., survival was not affected by length of irrigation period. When competition from other plants was a potential problem, however, there was significantly less mortality when trees with tillage to 1.2 m. received water for 240 days compared to those that received water for 159 days (table 4). This is the only evidence we have that irrigation in excess of the minimum 150-day period may significantly contribute to the survival of cottonwoods.
Deep tillage promoted growth and survival of cottonwood trees. Although deep tillage is an added initial expense, benefits are realized in terms of increased growth rates, survival, and reduced costs associated with prolonged irrigation. Faster growth rates are reflected in more rapid use of the area by wildlife. Use of irrigation minimizes water wastage. By learning precisely the minimum water requirements for the kind of growth desired, the full efficiency of drip irrigation can be realized. Data from table 5 indicate a 7.4% increase in mean height when trees were watered for 480 days. This amounts to 3 acre-feet (AF) per 40 ha. of trees. But by sacrificing the 7.4% of growth and irrigating for
|
|
only 150 days, the water requirements would be slightly less than 0.8 AF per year—a savings of 52,995,600 l. per 40 ha. In addition, maintenance and labor costs would be reduced. Use of light, portable irrigation systems would permit growing double the number of trees in the same time. This, of course, more than offsets any slight gain in productivity realized by irrigating for a longer period of time.
However, full irrigation efficiency can be realized only when we learn precisely the minimum amount of time irrigation is needed. Our field work, which spanned only 480 days after planting (excluding the dormancy period extending roughly from November 1979 through February 1980), was not long enough (150 days). By the time we realized that the shortest irrigation period in our experimental design still provided more than enough water for 100% survival, it was too late to plant more trees and water them for less time. We know that five months of irrigation is probably longer than necessary, but we do not know by how much. When we designed the study it seemed inconceivable that trees would survive with only five months of irrigation during one year. Every day that can be cut from the five months of irrigation means reduced water consumption and labor requirements, i.e., the total cost per acre of revegetating, will be reduced. A one-month reduction in irrigation time will reduce post-planting costs by 20%; a two-month reduction would cut costs by 40%.
Increased variance in growth among trees with no deep tillage is to be expected because in some places where trees were planted the soil was not as layered or compacted as in other places. Trees may thrive in such places even without deep tillage. In some places, even though soil layering may prevent roots from penetrating to the water table, the soil does not dry out to the depth to which the roots penetrate and the source of water is free of salts, so trees may thrive. Such conditions do occur on the dredge-spoil site. In some places, where the soil is layered at 1.5 m. or 1.8 m., the overlying layer is sand.
By backhoeing a hole along the side of trees that had no deep tillage, we were able to determine that their root systems were highly branched, penetrating the soil to a depth of only 1.2–1.8 m. The salt content of the soil was very low (<100 ppm). The overlying dredge-spoil, probably rich in nutrients, loses litle water through capillary action, contrary to capillary losses in heavier soil. The only source of water is rainfall, which contains no salts. Since the surrounding area is sand with low salt content, there is no runoff to carry salt into the roots. In heavier soils containing more salt, it is possible that irrigation would be required for more than five months. Until experimentation can be fully carried out on these points, we would not recommend extensive planting of Fremont cottonwoods anywhere except in sandy, relatively salt-free areas. We know that 121 l. of water per tree for five months is probably more than adequate.
Planting Recommendations
The following recommendations are based on conclusions drawn from planting under the range of conditions encountered on the dredge-spoil site. Our recommendations are conservative because they reflect a relatively narrow range of experimental conditions. We strongly discourage efforts to plant Fremont cottonwoods on a scale larger than about 2 ha. if any of the conditions included in the recommendations are not met. There is a high probability that failure to adhere to this recommendation would result in failure to establish trees and would be a waste of time and money. Money for revegetation projects outside the constraints listed below should be saved and put into a carefully planned and monitored experimental project. Such a project should be well funded, with adequate guarantees that funding will be available for completion of the project. This type of study would probably lead to a list of recommendations more liberal than those presented here. Our recommendations are based on facts that we learned from our experimental project.
1. Trees should be started from cuttings taken from local native stock. These cuttings should be allowed to take root and develop in a greenhouse for two or three months.
2. Planting should be done when the trees are small; the tallest shoot should not exceed 1 m.
3. The soil should be analyzed prior to planting. At this time we recommend planting only in sandy, relatively salt-free (<1200 ppm) soil.
4. The water table should be no more than 4.6 m. from the surface and the salt concentration in the ground water should not exceed 1200 ppm.
5. Deep tillage is of critical importance. Holes at least 20 cm. in diameter should be augered 3 m. deep or to the water table.
6. As a safeguard against salt damage to newly planted saplings, all holes should be leached 48 consecutive hours prior to planting.
7. Planting should be done only in January, February, and March.
8. Each tree should be supplied with 85 gm. of time-release fertilizer at planting.
9. Irrigation should begin no later than March and should extend for at least 150 consecutive days at about 114 l. per day. (This is not to be construed as meaning five days per week for 30 weeks. Such an interpretation could prove disastrous.)
10. Planting should not be done if Bermuda grass or a significant growth of any other vegetation is present.
11. Trees should be weeded regularly throughout the first summer to keep competition from other vegetation to near zero and to keep salt cedar from becoming established.
12. Rabbits, cotton rats, deer, burros, cattle, beavers, and perhaps other mammals will eat tree parts. At the first signs of damage, prompt action must be taken to protect the trees. Chicken wire placed around the saplings is adequate to keep small mammalian species from damaging the young trees. If weeds, such as Russian thistle, are present, the wind will blow them against the trees. On the dredge-spoil site, Russian thistle provided cover for cotton rats which ate the bark on the trees. We controlled the problem by piling the Russian thistle and burning it.
13. Trees with leaves, girdled by mammals, should be chopped down immediately. The tree will sprout from the root stock.
Acknowledgments
We wish to thank Jeannie Anderson, Susan M. Cook, Jane R. Durham, Dr. Julie K. Meents, and Cindy D. Zisner for editorial assistance. Marcelett Ector and Cindy D. Zisner typed the numerous drafts of the manuscript. Rodney H. Ohmart prepared the illustrations. Kurt Webb carried out the computer work. We are grateful to Dr. F. Aljibury, Les Ede, and Jule Meyer from the University of California Agricultural Extension Service, Riverside, California, and to Wayne Flanagan and the late Charles Morris, District Conservationists, USDA Soil Conservation Service, for their advice and cooperation. We thank Louise Disano for assistance with data collection and handling. The work was supported by the USDI Bureau of Reclamation and the USDI Fish and Wildlife Service Contract No. 7-07-30-V0009.
Literature Cited
Anderson, B.W., and R.D. Ohart. 1981. Revegetation efforts along the lower Colorado River. Final report, in preparation, to USDI Bureau of Reclamation, Boulder City, Nevada.
Ohmart, R.D., W.O. Deason, and C. Burke. 1977. A riparian case history: the Colorado River. p. 35–47. In : R.R. Johnson and D.A. Jones (ed.). Importance, preservation and management of riparian habitat: a symposium. [Tuscon, Ariz., July 9, 1977]. USDA Forest Service GTR-RM-43. 217 p. Rocky Mountain Forest and Range Experiment Station, Fort Collins, Colo.
Riparian Restoration Efforts Associated with Structurally Modified Flood Control Channels[1]
Bernard H. Goldner[2]
Abstract.—For the past five years, the Santa Clara Valley Water District has revegetated flood control channels to benefit wildlife and provide aesthetic improvement. Drought-tolerant ornamental and native riparian species have been planted along the channel banks and levees with mixed results. The most important determinants of successful establishment are a fixed irrigation system and a well-managed weed abatement program. Densely planted, liner-size rooted cuttings irrigated by overhead sprinklers can reduce both installation and maintenance costs.
Background
The semi-arid Santa Clara Valley is bounded on both sides by rolling hills and rugged mountainous uplands, the Santa Cruz Mountains to the west and the Diablo Range to the east. Drainage from both mountain ranges eventually empties into San Francisco Bay to the north and Monterey Bay to the south. The relatively flat valley floor is laced with a network of hundreds of miles of intermittently flowing streams originating in the surrounding foothills and mountain ranges. Because all the larger stream systems have been intercepted below their headwaters by water conservation dams, summer streamflows are enhanced by release of water from the reservoirs for groundwater recharge.
Many miles of riparian woodland streams are still in a relatively pristine or minimally disturbed condition, their banks lined with overstory vegetation of such trees as California sycamore (Platanusracemosa ), Fremont cottonwood (PopulusFremontii ), interior live oak (Quercuswislizenii ), valley oak (Quercus lobata ), box elder (Acernegundo var. californicum ), coast redwood (Sequoia sempervirens ), big-leaf maple (Acermacrophyllum ), alders (Alnus spp.), California black walnut (Juglanshindsii ), California bay (Umbellulariacalifornica ), and California buckeye (Aesculuscalifornica ). The understory of the streambanks consists of vines, shrubs, and herbaceous groundcover, including California blackberry (Rubusvitifolius ), poison oak (Rhusdiversiloba ), wild grape (Vitiscalifornica ), blue elderberry (Sambucuscaerulea ), coyote brush (Baccharispilularis ), toyon (Heteromelesarbutifolia ), California wild rose (Rosacalifornica ), willows (Salix spp.), and mulefat (Baccharis viminea ).
The bottom freshwater marsh community of perennial streams includes cattails (Typha spp.), rushes (Scirpus spp.), sedges (Carex spp.), knotweed (Polygonum spp.), and watercress (Rorippanasturtium-aquatica ). The more disturbed streams are characterized by a mix of native, naturalized, and introduced species such as eucalyptus (Eucalyptus spp.), giant reed (Arundodonax ), various orchard trees, and ornamentals escaped from yards.
In contrast, the freshwater habitat is modified by tidal influence in the baylands surrounding San Francisco Bay, where many of these streams support a distinctive levee or "eyebrow" salt-marsh plant community. Within a vertical distance of a few feet, the entire range of plants normally found in salt marshes typically occurs. Lowermost there is a thin line of cord grass (Spartinafoliosa ), backed by a few inches of pickleweed (Salicorniavirginica ), and finally a succession of salt grass (Distichlisspicata ), brass buttons (Cotulacornopifolia ), and mostly salt-tolerant, non-native upland grasses, herbs and shrubs.
The streams support a rich and varied assemblage of wildlife, some of which are wholly dependent upon the riparian environment for survival. Several of the larger streams still provide spawning grounds for the anadromous steelhead rainbow trout (Salmogairdnerii ). The streams also support a variety of native fish species.
[1] Paper presented at the California Riparian Systems Conference. [University of California, Davis, September 17–19, 1981].
[2] Bernard H. Goldner is Environmental Specialist, Santa Clara Valley Water District, San Jose, California.
On the heavily urbanized valley floor of north Santa Clara County and even in the rural/agricultural south county, streams provide virtually the only habitat remaining for wildlife; their stately trees also provide a greenbelt of visual relief from the surrounding urban setting. The Santa Clara County General Plan recognizes these stream systems as a unique scenic, wildlife, and recreational resource and includes policies designed to encourage their preservation. A number of the more scenic streams have been developed jointly as linear park chains with the cooperation of the county, cities, and Santa Clara Valley Water District (SCVWD); many more are designated in the General Plan as future park chains.
Of the nearly 1,130 km. (700 mi.) of streams within the jurisdiction of the SCVWD (which includes most of the streams in the county), only about one-half can carry the 1% (100-year) floodflow. In the event of flooding of this magnitude countywide, over 32,800 ha. (81,000 ac.) of land would be flooded, with damages estimated in excess of $300 million. Consequently, as the flood management agency in the county, the SCVWD must continually plan and construct various types of flood damage reduction projects, many of which require that the hydraulic capacity of streams be substantially increased by excavation, i.e., widening the banks and deepening the channel bottom (channelization). Channelization results in major structural modification of natural streams by transforming them into flood control channels which include: 1) trapazoidal excavated earth channels; 2) concrete-lined channels; 3) trapezoidal earth channels lined with rock, concrete sack, or gabion (rock-filled wire baskets) riprap for slope stability; and 4) floodways enclosed with levees or floodwalls with or without channel bed excavation. Maintenance roads are located at the top of the channel bank or depressed near the bottom. Where there is sufficient land available, there is the option of excavating the stream from one bank to preserve high-quality riparian vegetation, or constructing a by-pass channel to avoid disturbing the stream. In most developed areas, however, the choice narrows down to removing adjacent homes and buildings or to channelizing the stream.
The types of modifications described above result in impacts on riparian vegetation ranging from slight disturbance to permanent removal with commensurate effects on dependent faunal communities and aesthetic values. While some channelized streams revegetate by natural succession, the extent and rate at which reestablishment occurs is unpredictable and depends upon a number of little-understood variables, including whether the channel slopes are concrete-lined or riprapped, the availability of upstream seed sources, soil temperature and moisture, streamflow regimen and velocities, steepness of the side slopes, fertility and compactness of fill material, and intensity of vegetation and sediment removal in the channel to maintain the constructed flow capacity.
History of the Santa Clara Valley Water District Revegetation Program
Early Projects
Partly as a response to the California Environmental Quality Act (CEQA) of 1970[3] and partly due to public concern, the Board of Directors of the SCVWD adopted a resolution in 1974 that set policy for landscaping of district projects.[4] Prior to 1974, landscaping associated with flood control channels was limited to screening highly visible street crossings. The resolution redefined and broadly expanded the purpose of the landscaping program to include the reestablishment of riparian vegetation and enhancement of aesthetic values for all new projects. The resolution also specified that the landscaping material be of a low-maintenance type, adapted to the climate and soil conditions of the flood control channel, and not require irrigation after a short establishment period. Thus, the plants selected would have to be drought-tolerant species, but not necesssarily California natives.
Following these guidelines, landscape projects were installed in 1976 in conjunction with four flood control projects. One of the landscape projects was installed along a rock-lined excavated earth channel located in a residential area (Randol Creek). A second was located along a partly excavated channel enclosed with levees and a floodwall (San Tomas Aquino Creek). The third was along an earth channel designated as a linear park chain, featuring a trail system and other recreational amenities (Los Gatos Creek). The fourth was located along the banks of a gabion-lined channel located next to an expressway (Guadalupe River).
Although the setting and type of channel varied, these projects shared a number of design features which are discussed below.
Plant Selection
Because of the dual purpose of enhancing aesthetic values of the flood control channels and providing vegetation of value to wildlife, the plants consisted of drought-tolerant species dominated by California natives, but including several introduced species, such as eucalyptus. Not all of the natives were riparian woodland species found in Santa Clara County, but they were considered to be compatible with the flood control channel environment. (Detailed information about the growth requirements and habits of drought-tolerant and California native plants can be found in Stiles [1975] and California Department of Water Resources [1979].)
The sizes of the plants used were 2-in. peatpot liners and 1-, 5-, and 15-gal. container stock selected from commercial nurseries. The
[3] PRC Section 21000–21151.
[4] Resolution 74–40.
park chain project consisted almost exclusively of liners. A seed mix of annual flowers, perennials, and permanent groundcover species was applied to the bare soil between the planting basins by hydroseeding.
Native trees used included: big-leaf maple, box elder, California buckeye, white alder (Alnusrhombifolia ), Pacific madrone (Arbutusmenziesii ), California sycamore, Oregon ash (Fraxinuslatifolia ), coast live oak (Quercusagrifolia ), California black oak, valley oak, red willow (Salixlaevigata ), yellow willow (Salixlasiandra ), blue elderberry, California bay, coast redwood, tanbark oak (Lithocarpus densiflora ), Monterey pine (Pinusradiata ), and mountain dogwood (Cornusnuttallii ).
Native shrubs and vines used included: Parry manzanita (Arctostaphylosmanzanita ), coyote brush, toyon, scrub oak (Quercusdumosa ), California coffeeberry (Rhamnuscalifornica ), sugar bush (Rhus ovata ), California wild rose, purple sage (Salvia leucophylla ), California huckleberry (Vaccinium ovatum ), California wild grape, blue blossom (Ceanothusthyrsiflorus ), flannel bush (Fremontodendron californicum ), California blackberry, virgin's bowers (Clematislasiantha ), and California barberry (Berberisdictyota ).
Not all of the plant species listed above were used for each project.
Design Concept
Because flood control channels are expected to maintain their designed flow capacity and remain hydraulically efficient, woody vegetative growth on the channel bottom which could lead to log jams, obstructions, or snags during high flows is removed. Such requirements dictate where plantings can remain and the types and number of plants that can be used. Flood control projects, if they are to be revegetated, should ideally be constructed with sufficient extra capacity to allow for the growth of a certain amount of mature trees and shrubs within the channel. Groundcover and smaller shrubs are acceptable along the lower portions of the channel side slopes, but trees must be placed near the top of the slopes or along the top of the banks or levees. Plants must be located so as to avoid interference with vehicle and equipment access along the maintenance roads.
These constraints preclude any attempt to simulate a terraced plant community characteristic of natural riparian ecosystems. Because the cross section of the channel bed is drastically altered by channelization, the land/water interface is affected. This in turn alters the complex relationships between the various vegetative zones that exist within riparian plant communities. Most of the plantings must be located along the upper channel slopes and the tops of banks and levees, far above the saturated water zone. This not only modifies the natural conditions of indigenous riparian species, but increases their reliance on a source of supplemental water during the establishment period. However, in spite of these design limitations, revegetated channels can provide an "edge effect" attractive to wildlife and provide cover, food, and roosting and nesting opportunities for wildlife. Moreover, revegetation, whether with riparian species or not, enhances the aesthetic values of flood control channels and provides erosion protection for the banks.
Irrigation
Largely as an economy measure, none of the projects had a permanent irrigation system. The plants were truck-watered during the dry season with the aid of large watering basins constructed around each plant. The hydroseeded areas were not irrigated.
Maintenance
During the establishment period, maintenance activities were carried out either by SCVWD personnel, another public agency, or a landscape contractor. The program consisted of watering, basin repair, tree stake repair, pest and weed control, erosion repair, trash removal, replacement of diseased or dead plants, and protective fence repair. As expected, watering and weeding required the most labor.
Evaluation
Unfortunately, planting all four projects in 1976, at the beginning of one of the severest two-year droughts in California history, coupled with the lack of a permanent irrigation system, proved to be disastrous to the survival of the plants. A survey of survivors taken three years after installation showed that losses for three of the projects were about 75% on the average. The park chain project which relied mostly on liners suffered an almost total loss. The hydroseed mix either did not germinate or germinated poorly with only scattered California poppies, lupine, and a few daisies evident among the multitude of weeds.
It was apparent that the truck-watering regimen was not efficient or timely enough to compensate for the lack of adequate rainfall; competition from the profuse growth of weeds for water and nutrients contributed to the demise of the badly stressed plants. Disease organisms and insects probably took their toll as well. Aggravating the situation were complaints from public officials and citizens who viewed the projects as expensive weed patches with no aesthetic benefit to the community. Criticism was especially strong regarding the park chain project where the objective of revegetation was perceived by the public as primarily aesthetic improvement of the channel.
The most important lesson learned from the design of these projects was that natural rainfall cannot be relied upon to establish plantings. A fixed irrigation system is mandatory to ensure a high rate of survival until the plants are well established. The irrigation system can then be abandoned or possibly salvaged for use in other projects.
Intermediate Projects
The next two flood control channel revegetation projects were not installed until 1979, three years later. One project was installed along a realigned excavated earth channel with depressed maintenance roads. It was located in a commercial-industrial park; the primary objective of the project was replacing a riparian habitat lost just upstream due to channelization of the stream (Calabazas Creek). Due to its high visibility, aesthetic enhancement of the channel was an additional goal. California native riparian species were used, together with several native upland species. Trees (1- and 5-gal. size) consisted of California buckeye, Oregon ash, knobcone pine (Pinusattenuata ), coast live oak, and California black oak. Shrubs (1-gal. size) and groundcover (liner size) were California sage brush (Artemisiacalifornica ), quail bush (Atriplexlentiformis ), blue blossom, California buckwheat (Eriogonumfasciculatum ), toyon, California coffeeberry, and dwarf coyote brush (Baccharis pilularis 'Twin Peaks').
The second project was installed along an excavated earth channel enclosed with levees in a developing area (Berryessa Creek). The purpose of revegetation in this case was not primarily to restore wildlife habitat, since the creek had low habitat value to begin with due to previous channelization. Rather, the purpose was to provide an aesthetic asset to the community. A citizen's committee assisted in developing the design concept of the landscape project. The plants selected were largely drought-tolerant species and included several California natives. Trees (5-and 10-gal. size) consisted of red horsechestnut (Aesculuscarnea ), Bailey acacia (Acacia baileyana ), Sydney golden wattle (Acacia longifolia ), coast redwood, California sycamore, allepo pine (Pinushalepensis ), holly oak (Quercus ilex ), and coast live oak. Shrubs (1-gal. size) were bearberry (Arctostaphylosuva-ursi ), Point Reyes ceanothus (Ceanothusgloriosus ), crimson spot rockrose (Cistusladanifer ), toyon, English lavender (Lavandulaangustifolia ), Pacific wax myrtle (Myricacalifornica ), glossy privet (Ligustrumlucidum ), California fuchsia (Zauschneriacalifornica ), Italian buckthorn (Rhamnusalaternus ), and Japanese barberry (Berberisthunbergii ).
Both projects included a drip irrigation system with an emitter head placed under the mulch of each watering basin. All irrigation pipe was buried.
Evaluation
In terms of plant survival, growth rate, and community acceptance, both projects are considered quite successful. Losses have amounted to only about 10–15%, with vandalism and predation by rabbits and squirrels responsible for most of the losses. Although the projects are fenced to discourage public entry, youngsters have repeatedly climbed or cut the chain link gates and fences to break tree stakes and destroy emitter heads and irrigation lines.
These projects have been well maintained, so that weed growth has not detracted from the appearance or overgrown the planted materials. Weed abatement was accomplished by hand labor and application of herbicides. Although the level of maintenance was higher than the earlier projects, the success achieved undoubtedly can be attributed to the advantages afforded by a fixed irrigation system. Figure 1 shows the projects two years after planting.

Figure l.
Revegetated flood control channels two years after planting.
Top: reach of Calabazas Creek. Note pedestrians on depressed
maintenance road. Bottom: reach of Berryessa Creek. Note
wide spacing of plantings on levees and freshwater marsh
vegetation on channel bottom.
Recent Project
The drastic reduction in revenue engendered by the passage of Proposition 13 in 1978 required a reevaluation of all SCVWD programs, to determine where expenditures could be reduced. The
landscape program evaluation led to the development of a new policy, formalized in a resolution adopted by the board of directors in 1979.[5] In contrast to the 1974 policy, which stated that all flood control projects would be landscaped for both aesthetic enhancement and environmental improvement, the new policy did not recommend revegetation if the impacts of channelization on existing habitat are minimal, or if it is likely that natural reestablishment of vegetation will occur in a reasonably short period. Under this policy, the loss of mature riparian woodland, for example, would be mitigated, but a freshwater marsh community dominated by willows and cattails would probably not be revegetated, due to their rapid regrowth. Planting primarily for aesthetic purposes would be carried out only if a flood control project resulted in significant visual impacts unacceptable to the community.
Thus, the emphasis of the program has shifted in focus to mitigation of substantial adverse impacts, in conformance with CEQA. The term "revegetation" is more descriptive of the current program than "landscaping" since the goal is to reestablish riparian habitat primarily for the benefit of wildlife. Obviously, as the plants mature, aesthetic benefits will accrue, but the public must now be educated to understand that the unique quality of streams is their natural appearance and that a park-like setting is not appropriate in most cases. Weeds will be allowed to coexist in spite of their unsightly appearance, as long as they do not gain a competitive advantage over the plantings or result in a fire hazard.
Between March and May, 1981, a revegetation project was installed along an excavated earth channel partially lined with gabions and located adjacent to the SCVWD administration building (Guadalupe River). The project was the first to follow the current revegetation policy; it also presented an opportunity to test some new concepts designed to reduce the costs of both installation and maintenance.
Plant Selection
The plants chosen were predominantly California natives associated with riparian communities in Santa Clara County and included three cultivated upland species (cultivars). Trees consisted of California buckeye, interior live oak, valley oak, and California black walnut. Shrubs and groundcover included California wild rose, blue elderberry, coyote brush, and the cultivars—dwarf coyote brush (Baccharis pilularis 'Pigeon Point'), ceanothus 'Frosty Blue,' and ceanothus 'Concha.' The plants were obtained by contract with the Saratoga Horticultural Foundation, which specializes in growing drought-tolerant and California native plants. This was done to ensure the availability of the large number of healthy plants that were required for the project. The shrubs were started from stem cuttings; the tree species were collected as seeds. Except for the cultivars, cuttings and seeds were taken in the wild. The objective was to plant vigorously growing rooted cuttings (liner-size) of local genetic stock, rather than nursery-grown specimens that might be less adapted to the harsh conditions of the planting site. An additional advantage of liners and seeds over larger container stock is their considerably lower cost.
Unfortunately, due to a delay in completing the plans, the cuttings outgrew their containers and had to be repotted into 1-gal. size containers. All the buckeye and most of the valley oak seed sprouted and therefore were potted and planted as liners. Dwarf coyote brush was repropagated and also planted as liners. Instead of a more desirable early winter planting, planting occurred in late spring. Nevertheless, the plants were still vigorous and not rootbound, as might be the case for more mature 1-gal. size nursery stock.
Design Concept
Earlier irrigated projects spaced the plantings 3–6 m. (10–20 ft.) apart and depended upon a relatively high level of maintenance to control weeds in the unplanted areas. In this project, plants and seeds were installed on 1.5-m. (5-ft.) centers arranged in a random pattern to achieve a natural effect. Although such high density required a relatively larger number of plants, it was expected that they would rapidly fill in the voids, overgrow and suppress the weeds, and thereby reduce short- and long-term maintenance costs.
Low-growing shrubs were also placed in the occasional planting voids left between the gabions protecting the lower channel slopes.
Preparation of Planting Site
To reduce early weed competition and subsequent maintenance effort, the site was prepared first by mechanical weed removal. This was followed several months later by application of a contact herbicide to new weed growth, prior to planting. All planting holes were backfilled with a mixture of native soil, soil amendments, and a slow-release fertilizer.
Irrigation System
Because there were about 2,500 planting basins, it was far less expensive to install an overhead impact irrigation system rather than a drip irrigation system. The irrigation pipe, as in previous projects, was buried.
Maintenance
The installation contractor was awarded a 12-month contract to provide all maintenance necessary for survival of the plants. As an incentive to ensure good-quality work, the con-
[5] Resolution 79–76.
tractor was made responsible for a 70% minimum survival rate of all plants and germinated seeds. If after the 12-month period losses exceed 30%, the contractor must replace the plants in kind or pay a penalty of $4 per planting hole. This arrangement allowed the watering schedule and level of weed control to be left to the discretion of the contractor. A further incentive is the possibility of being awarded a follow-on 12-month maintenance contract. After a two-year period, the plants should be established, and only minimal maintenance should be required of the SCVWD maintenance crew which will assume responsibility for the project. The irrigation system will be left in place for a few additional years in case supplemental summer watering is required.
Evaluation
Although only three months have passed, judging by survival rate and vigor of the plants, the project is off to an excellent start. About 90% of the plantings have survived and most species have added 0.6–0.9 m. (2–3 ft.) of new growth. The liner-sized plants have grown especially vigorously and have proved their value. The blue elderberry has grown so profusely that it threatens to overgrow and suppress several slower-growing species. The one disappointment is the poor rate of seed germination. Only a few of the black walnut and interior live oak seeds have sprouted. It is probable that the seeds lost viability due to the long delay that occurred between gathering and planting. Replanting with fresh seed is being considered since this cost-saving technique deserves a fair trial.
Weed control has been accomplished by a combination of manual labor and use of a pre-emergence herbicide, Trifluralin, and a post-emergence herbicide, Diquat, applied between the planting basins. Because the irrigation system has worked effectively and the plants have grown rapidly, weed competition has been minimal even though the weeds, at times, have grown abundantly both in the voids between the plants and within the planting basins.
Resident ground squirrel and rabbit populations, feared at first to be a threat to the new plants, have not proven to be deterimental. A squirrel control program begun before installation was discontinued.
As might be expected, the project has suffered several incidences of vandalism, evidently from youngsters who frequent the area for various activities. The sprinkler heads, which are located at the top of the banks next to a maintenance road, have been damaged or stolen on several occasions. Brightly colored plastic markers placed in the seed planting basins promptly disappeared, but colored wooden stakes securely driven into the basins as replacements have remained in place. Unlike several previous projects, the plants themselves have escaped significant vandalism, possibly because their small size and the large number of weeds makes them difficult for the untrained eye to identify.
Figure 2 shows the project three months after planting. Although the project is still in the very early stages of development, the indications are encouraging that this design concept will prove to be the most successful approach to revegetation attempted thus far.
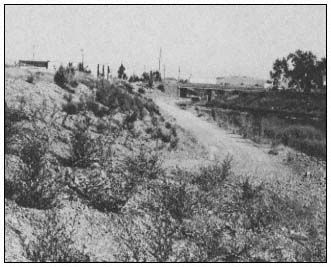
Figure 2.
Revegetated reach of Guadalupe River flood control
project three months after planting. Note plantings
on slopes above depressed maintenance road.
Summary
1. Even with the constraints that the design, operation, and maintenance of flood control channels place on revegetation with riparian woodland species, it is feasible to benefit wildlife and provide aesthetic improvement.
2. To ensure that most of the plantings survive through the establishment period, a fixed irrigation system must be provided. To reduce installation and maintenance costs, an overhead sprinkler system will provide effective irrigation, although exposed sprinkler heads may be more prone to vandalism than drip emitters hidden within the planting basins.
3. It is practical to establish plants from rooted cuttings (liners) rather than larger container stock as a significant cost-saving measure. Placing smaller-size plants at high densities, in contrast to widely spaced larger plants, appears to be a good method of reducing weed competition and rapidly providing cover for wildlife. An additional advantage is the vigorous growth of smaller plants.
4. The detrimental effects of weed competition during the establishment period can be minimized by installation of an effective irrigation system, as well as a weed management program com-
bining hand labor and the judicious use of selected herbicides.
5. Vandalism has been a serious and costly problem in all projects and is likely to increase. Fencing, posting with warning signs, and similar passive methods are generally ineffective. Some design suggestions to reduce vandalism are: 1) placement of sprinkler heads in less accessible areas, such as midway down the steep side slopes instead of at the top of the banks; 2) using liners or 1-gal. size trees that do not require staking; and 3) involving the surrounding neighborhood in the planning, implementation, and monitoring of revegetation projects.
Acknowledgments
I am indebted to Steve Renfro, former District Landscape Architect, and Winthrop Stiles III, former District Assistant Environmental Specialist, who pioneered and contributed so much effort to the landscape and revegetation program of the SCVWD. They have left behind a living legacy.
Special thanks to Barrie Coate and the staff of the Saratoga Horticultural Foundation who provided invaluable advice about the cultivation of native plants.
Literature Cited
California Department of Water Resources. 1979. Plants for California landscapes. Bulletin 209, California Department of Water Resources, Sacramento.
Stiles, W.A. 1975. A landscaping guide to native and naturalized plants for Santa Clara County. Prepared for the Santa Clara Valley Water District, San Jose, Calif.
Streambank Stabilization Techniques Used by the Soil Conservation Service in California[1]
David W. Patterson, Clarence U. Finch, and Glenn I. Wilcox[2]
Abstract.—Streambank protection techniques used by the USDA Soil Conservation Service (SCS) have evolved over many years of experience. Structural and vegetative protection measures are generally used in combination. SCS designs are based on national standards promoting protection measures which are sound from environmental, economic, and engineering perspectives. The general principles for planning streambank protection measures are presented as well as brief descriptions and typical costs of several structural and vegetative methods.
Introduction
Streambank protection techniques are technical conservation practices requiring the combined planning efforts of engineers, biologists, plant material specialists, and agronomists. The USDA Soil Conservation Service (SCS) provides technical assistance through local resource conservation districts for the planning and design of streambank stabilization systems on America's smaller streams and rivers. It is fair to say that the techniques SCS now uses for protecting and revegetating streambanks have evolved over many years of trial, error, and success.
The response of resource agencies and the public to streambank protection projects has often been emotional. However, those who have closely evaluated SCS work are gaining confidence in our approaches to streambank protection. In several instances representatives from the USDI Fish and Wildlife Service (FWS) have used SCS methods for placement of natural rock riprap and reestablishment of vegetation as examples for streambank protection methods.
SCS is aware of its responsibility to design conservation practices which are sound from both environmental and engineering perspectives. Toward that end SCS has developed designs and techniques which serve as standards for streambank protection work.
Resources Worth Protecting
Throughout this conference a great deal of time and effort will be rightfully expended extolling the many inherent values of riparian systems. However, often overlooked, taken for granted, or at least underemphasized, is the value of soils and other substrates within riparian systems. Soils and other substrates located within and adjacent to water bodies and waterways provide the substrates on which riparian vegetation grows. In addition, deep alluvial soils of high agricultural value are often found within and immediately adjacent to riparian zones.
The erosion of riparian soils and substrates acts as a double-bitted axe. Erosion within riparian systems not only permanently removes productive soils and substrates and associated vegetation, but also results in a reduction in downstream water quality (fig. 1). Streambank erosion and associated stream meander and soil loss are unacceptable to most private landowners, especially for owners of high-value specialty crops such as grapes, as well as in urban areas. Streambank erosion and meander within private lands can result in the loss of highly productive and valuable agricultural soils, loss of or damage to expensive irrigation systems, levee failure, loss of real property, desiccation of meadows, and loss of riparian vegetation (fig. 2). Head cutting (fig. 3) in streams and gullies is another major source of erosion and sedimentation, leaving scars on the face of the earth that are difficult and expensive to heal.
[1] Paper presented at the California Riparian Systems Conference. [University of California, Davis, September 17–19, 1981].
[2] David W. Patterson is Biologist, Red Bluff, California; Clarence U. Finch is Agronomist, Fresno, California; Glenn I. Wilcox is Biologist, Salinas, California; all are with the USDA Soil Conservation Service.
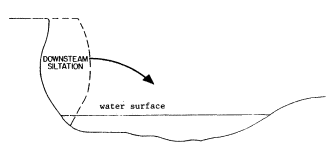
Figure 1.
Streambank erosion results in the loss of streamside
soils, substrates, and associated riparian vegetation,
which enter the waterway, resulting in siltation
and reductions in downstream water quality.

Figure 2.
Active streambank erosion destroys valuable riparian vegetation.
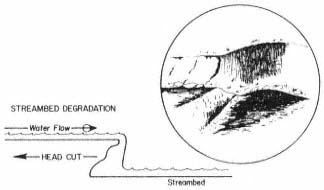
Figure 3.
Head cutting and streambed degradation are major causes
of erosion and streambank sloughing in waterways.
Some General Principles
SCS recognizes and supports the need for proper land-use planning on land areas containing waterways. This is especially important in areas where inadequate planning has allowed urbanization up to the edges of eroding and meandering streams. SCS also encourages the practice of setbacks and appropriate use where agricultural lands contain or are traversed by riparian systems.
Several principles are observed by SCS in the design of streambank protection systems. Channelization of waterways must be avoided, otherwise upstream repairs may create new problems downstream. As an example, adequate natural meander must remain to dissipate flow energy (fig. 4 and 5). Protection work must be carefully planned to avoid removing or damaging any more vegetation than is absolutely necessary. It is especially important to determine if apparent streambank erosion is actually a symptom of streambank degradation. Streambank protection efforts are doomed to failure if streambed degradation and undermining are creating evermore critical angles of repose on associated streambanks (fig. 6).
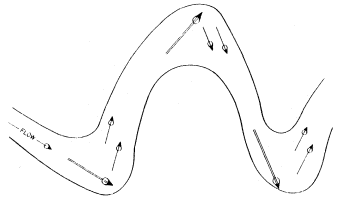
Figure 4.
Stream meanders dissipate flow energy naturally.
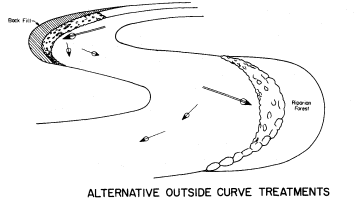
Figure 5.
Streambank protection measures for outside curves
must retain adequate meander for energy dissipation.
The environmental attributes of streambank protection projects must be acceptable in the long-term. Too often people judge the environmental acceptability of projects immediately upon their completion. The streambank shown in figure 7 appears to be aesthetically undesirable, but protection measures were completed just before the photograph was taken. Figure 8 shows the same project five years later. In most cases it is five years before shrub and tree plantings achieve enough growth to reflect the total planning effort put into a typical project. It is important to keep this longer-term time frame in
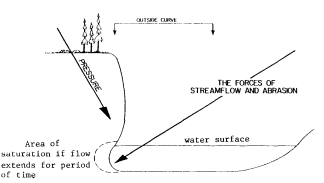
Figure 6.
The forces of streamflow and abrasion combined
with streambed degradation and gravity are
the key causes of streambank erosion.
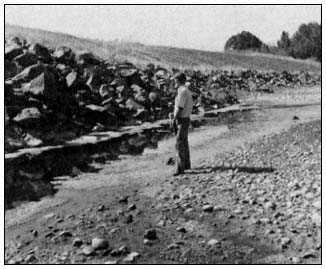
Figure 7.
A riprapped outside curve on Anderson Creek,
Mendocino County, just after construction was completed.

Figure 8.
The same riprapped outside curve on Anderson
Creek, Mendocino County, five growing seasons
after construction. Willow and alder are becoming
well established. Before the project the streambank
was a 3- to 6-m. (10- to 20-ft.) tall 90º cutbank
threatening a schoolyard.
mind when commenting on proposed and newly completed projects.
SCS does not recommend the use of either car bodies or concrete slabs for streambank protection. Uncompressed car bodies are unsightly and unsound from an engineering standpoint. Concrete slabs are unsightly, often lack adequate density, and, because of the typical flat configuration of the material, are subject to tumbling in high flows.
SCS and landowners consider opportunities to create or re-create fish habitat. On many projects summer habitats for both game and nongame fish are actually improved over preproject conditions. Large riprap rocks which fall to the stream bottom during construction are generally left where they fall near the toe of the bank. Water working around the instream rock, and between the rock and the armored bank, creates pools which, when combined with reestablished overhanging vegetation, provide quality summer habitat. In comparison, "naturally eroding" 90° cutbanks not only have little to offer, but serve as sources of downstream pollution and sedimentation (fig. 9).
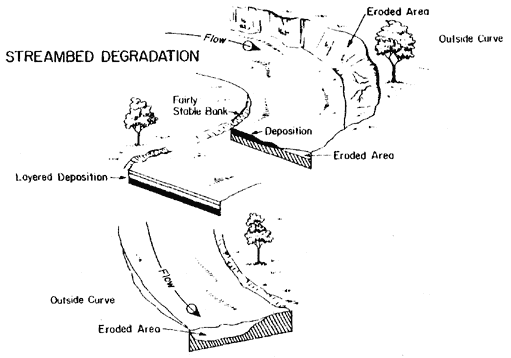
Figure 9.
Streambank erosion is a major factor in the
loss of riparian vegetation and substrates, as
well as sedimentation and water pollution.
Design and Planning Considerations
During project planning SCS engineers and planners consult SCS biologists and invite input from other agencies including the California Department of Fish and Game (DFG) and FWS. Cooperation during planning and design is now required under formal Channel Modification Guidelines (USDI Fish and Wildlife Service and USDA Forest Service 1978) which were jointly written by SCS and FWS. SCS conducts formal environmental evaluations for all streambank protection projects for which it provides designs. Both DFG and FWS are asked to participate in the evaluation process.
Landowners requesting SCS project designs are required to obtain all necessary permits before starting construction. Streambank protection work is carried out by cooperators during lowest flow periods or in dry streambeds when conditions for construction are best and the potential for downstream pollution is the lowest.
Streambank protection designs are normally limited to armoring outside curves, protecting instream structures, and protecting straighter reaches where loss of streamside resources is unacceptable. All designs must comply with appropriate SCS, state, and national conservation practice standards and specifications.
These design standards and specifications include:
1) channel vegetation;
2) critical area planting;
3) grade stabilization structure;
4) stream channel stabilization;
5) open channel;
6) streambank protection;
7) sediment basin.
Design criteria are also drawn from SCS engineering handbooks, engineering field manual, field office technical guides, and other technical resource material.
Streambank Protection Techniques
Although most often used in combination, SCS streambank protection techniques can be divided into two types: a) physical protection; and b) vegetative protection.
Physical Protection Techniques
Grade Stabilization
A dam, wall, or drop is used to reestablish or maintain streambed elevation (fig. 10). Maintenance of streambed elevation is necessary in stabilizing streambanks. Environmental considerations in designing grade stabilization measures include choice of construction materials, fish passage, plunge pools, number of structures used and their locations, and incorporation of vegetation.
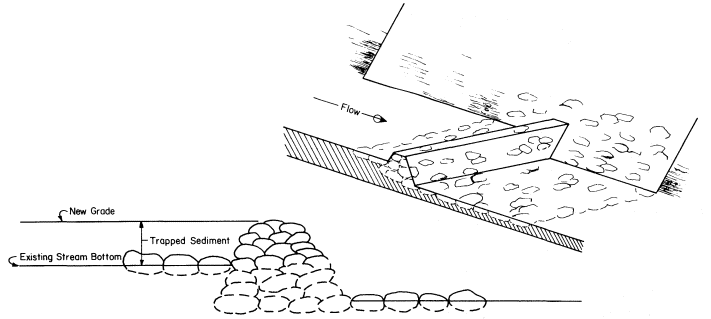
Figure 10.
Grade stabilization structures used to either maintain existing grade or raise streambottom by trapping sediment.
Rock Riprap
Natural rock of appropriate size, density, and gradation is placed on shaped streambanks and in open-toe trenches. A gravel filter is placed under the rock to prevent erosion of soil material from beneath (fig. 11). Extensively eroded outside curves are generally realigned with enough "bend" left for necessary energy dissipation. Environmental considerations include impacts at sites where rock is obtained; preservation of as much existing streamside vegetation as possible during construction; construction during low-flow or dry stream conditions; preservation or enhancement of fish and wildlife habitat; fencing; and seep trenches. Stabilization of streambed grade may be an integral part of this measure.
Concrete grout may be incorporated where channel alignment problems and/or flow velocities require greater protection from the influence of scouring or high-energy flows. Environmental considerations include the use of vegetation,

Figure 11.
A typical streambank in cross section protected with natural rock over gravel bedding and willow cuttings.
The toe trench is used to trap bank seepage and encourage better growth of cuttings and natural vegetation.
visual impacts, aquatic life, and use of colored grout.
Post-and-Wire Revetment
Wire mesh strung on four-inch steel diameter or wooden posts, braced with steel cables along the top and bottom edges of the wire mesh, are used where flow, abrasion, and instream debris conditions will allow. Post-and-wire revetments are commonly used where rock is unavailable or not cost-effective in comparison. Riparian plantings immediately behind wire revetments are an integral part of revetment establishment and maintenance.
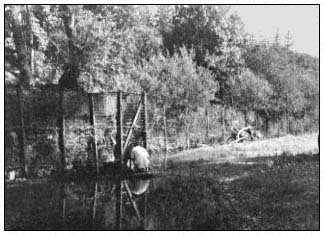
Figure 12.
A typical rail-and-cable revetment located
on Rancharia Creek in Mendocino county.
Rail-and-Cable Revetment
Rail-and-cable revetments (fig. 12) are similar in general appearance and function to post-and-wire revetments but are usually taller and made of heavier material to withstand greater water velocity, more abrasion, and debris problems. The revetment is covered with wire mesh with cables strung along the bottom, middle and top of the revetment. Riparian plantings are important components of rail-and-cable revetments.
Rock-and-Wire Revetment
Rock-and-wire revetments are essentially a well-braced double post-and-wire revetment with rock or very coarse gravel placed between the revetment walls. Riparian plantings are an important component of this type of revetment. Although they are expensive, properly located and constructed rock-and-wire revetments are quite stable as long as the wire withstands abrasion.
Pilot Channels
Pilot channels are dug in streambed material and used to "train" water away from newly treated streambanks, keep water out of construction areas, and increase chances of establishing newly planted vegetation.
Gabion Baskets
Gabion baskets are rectangular, heavy-mesh wire baskets filled with coarse gravel, which are placed and then wired together in series. Gravel size must be large enough to prevent leakage through basket mesh. Abrasion may break the wire mesh, requiring periodic maintenance. Gabions are used where large rock is unavailable or not
cost-effective. Plant material, generally in the form of willow cuttings, should be placed under, behind, and within baskets where moisture is adequate for growth.
Jacks and Cabled Trees
Past experience has shown these two methods to be less effective than many other methods. Cabled trees may be considered where ample trees are available and cost of other methods is absolutely prohibitive.
Vegetative Protection Techniques
Woody Cuttings
Cuttings are taken from locally adapted and locally growing plants when possible. Species which have been used include willow (Salix spp.), athel (Tamarixaphylla ), and tamarisk or salt cedar (Tamarixgallica ). Methods and procedures for taking and planting cuttings are provided in construction plans and specifications written for each project. In general, cuttings are laid horizontally in open-toe trenches and on shaped banks, as shown in figure 13, or planted vertically. If natural moisture is inadequate for growth, irrigation must be provided to insure establishment. Vertical plantings should be placed at a minimum depth of 1 m. (3 ft.) with 0.3 m. (1 ft.) of the cutting extending above the ground. The growing tip must extend aboveground. The best results have been obtained by augering holes for cuttings and watering cuttings in by washing soil material in around them. Watering in insures that cuttings are completely surrounded by soil which is devoid of air spaces. Immediate and generous irrigation should be provided at the time of planting. Water jetting can be used to both dig holes and water in plants where adequate water supply and pressure are available and soil texture allows.
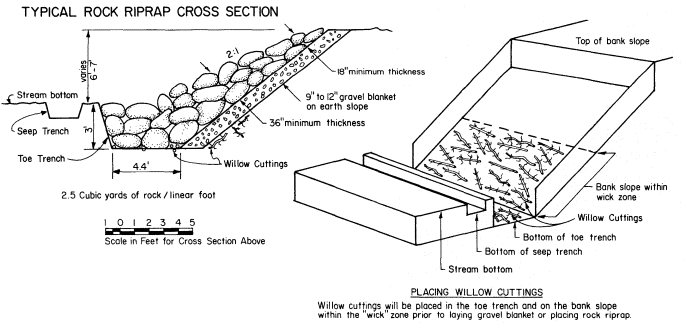
Figure 13.
Placement of willow cuttings on bottom of toe trench and on moist areas (wick zone) of sloped banks.
Rooted Woody Plants
Rooted woody plantings are made on the bank side of revetments or on levees or streambanks above bank protection materials. Rooted plants can also be placed in planters set in concrete or gunnited rock. Unless natural seepage is adequate, plantings must be irrigated for at least two or three years for establishment. Plant species selection is influenced primarily by site conditions, soils, climate, plant availability, price, and wildlife habitat needs. Mulching is strongly recommended around and between plants. Herbaceous plants can be used in combination with rooted woody plants but should not create competition for the woody plants.
Herbaceous Plants
Perennial and annual grasses and forbs are seeded on sloping banks where mineral soil is exposed and on any disturbed areas on top of banks. Species selection, site preparation, timing, and seeding are all critical elements. If planted in combination with rooted woody plants, herbaceous seedings should be kept several feet away from establishing woody plants to avoid competition for space, moisture, and nutrients.
Mulching
Mulching around woody plants and over seedlings with straw and erosion control blankets
made of jute, fiberglass, wood fiber, and other materials is recommended on steep and erosive streambanks and levees. Straw should not be chopped in lengths shorter than 15 cm. (6 in.) and should be anchored either mechanically or with fiber mulch and a tackifier. Fiber mulch should be wood cellulose fiber containing no germination- or growth-inhibiting properties. Fiber mulch should be hydromulched as a slurry containing tackifier at a rate of 1,500 pounds per acre. Seed can be either mixed with the slurry or the fiber slurry applied over the seeded area.
Costs
Location, situation, type of contract, contractor, and project size greaty influence the price paid for a particular job. The price ranges of some common streambank protection methods are given below. When used in combination, the costs of each operation or structure type must be added together. Maintenance costs must also be taken into consideration.
Dumped rock (quarry run)—$20.00 or more per foot.
Livestock fence revetment (metal fence posts with braces and hogwire)—$0.40 to $0.60 per foot.
Four-inch by four-inch treated wood fence post-and-wire mesh revetment with braces—$12.00 to $15.00 per foot.
Four-inch double steel pipe, wire, and rock revetment with braces and ties—$15.00 to $30.00 per foot.
Rail-and-cable revetment (9-pound rail and one-inch or larger cable)—$30.00 per foot.
Combination seed, fertilizer, and mulch—$600.00 to 1,000.00 per acre with higher costs for smaller areas.
Planted woody cuttings—a) watered in—$2.00 to $3.00 per cutting; b) jammed in—$0.50 to $1.00 per cutting.
Planting one-year-old rooted woody plants—$2,400.00 to $2,700.00 per acre (includes materials).
Streambank protection projects are expensive in terms of dollars spent. Furthermore, riparian resources provide few direct benefits to private landowners relative to the cost of protecting and maintaining the resource. The conservation of streamside soils must be viewed as a long-term investment for the good of future generations. Values such as natural beauty, fish and wildlife habitat, and water quality enhancement directly benefit the public in both the short and long term. For this reason, the financial participapation of the public in riparian conservation programs is strongly encouraged. In the Scott Valley alone since 1968, over $1 million has been spent protecting streambanks along the Scott River. Approximately 70% of the cost has been provided by cost-shares under the USDA Agricultural Conservation Program (ACP). Much more work and much more cost-sharing are needed on the Scott River and throughout the state and nation.
Conclusion
For years SCS seemed to wear, and to some degree deserved, a black hat when it came to "doing its thing" in streams and waterways. Things have changed for a number of reasons. SCS has, through experience, learned some lessons and developed creative and feasible methods of streambank protection and conservation of riparian systems. Figure 14 shows an example of quality streambank protection work utilizing natural rock riprap designed by SCS. After 13 years the project is nearly mature, providing quality habitat. A woman who passes this site every day reported that she could not believe the riverbank had been shaped and riprapped. The SCS standards and specifications and other technical materials are available at local, area, and state SCS offices. Public participation in planning and evaluating streambank protection systems is always invited.
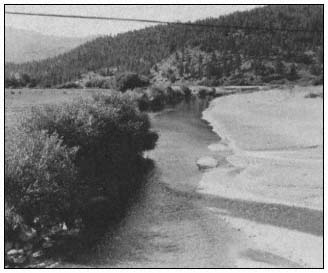
Figure 14.
A fully mature project creating quality terrestrial
and aquatic habitat, as well as providing strong
visual appeal. It takes time and money.
Literature Cited
USDI Fish and Wildlife Service and USDA Soil Conservation Service. 1978. Channel modification guidelines. 15 p. Limited publication for agency use. Washington, D.C.
Environmental Resource Conservation
Riparian System Enhancement Through Water Reclamation[1]
Ronald LaRosa[2]
Abstract.—A proposed project would provide for the establishment of 2,000 native trees over 4 ha. (10 ac.) of floodplain. These public lands would be irrigated with wastewater from a planned water reclamation facility. The irrigation regime would be based on data from ongoing reclamation projects in the San Diego region.
Introduction
One of the most sensitive environmental resources in San Diego is the riparian or streamside woodland. Comprised of some five representative tree or tall shrub species, this plant community has been adversely affected by land-use development. Even where woodlands have been spared by open space easements or low-density development, environmental impacts include degradation through disturbances, clearing of understory vegetation, and interruption of continuous tree cover necessary for the systems' use as wildlife corridors.
Streamside vegetation occurs in areas subject to over- and/or underground water flows and can be seen in both inland and coastal drainages within the San Diego region. Species such as elderberry, willow and cottonwood (see table 1 for specific names) are common to woodlands occurring in these mesic or moist habitats (Sands 1977). Along the coast, sycamore and coastal oak replace species adapted to the montane climates of higher elevations.
One resource value of a dense woodland growing along a watercourse (intermittent or year-round) is in the habitat afforded various species of animals which live in, among, and under the vegetation. Where surface water is available year-round, an even greater diversity of fauna can be supported in addition to the trees and shrubs which provide food, nesting sites, and roosting cover.
|
These biological zones are so critical to diverse wildlife populations, for watersheds, and as aesthetic open spaces, that they have been the subject of extensive environmental legislation and local ordinances. In the San Diego region, the most effective measure yet developed to conserve these woodlands is the creation of open space easements and other withholding of development rights. To date no major effort has been made to create or enhance riparian woodland by establishing native trees and shrubs which would be irrigated until their root systems could utilize groundwater.
An environmental enhancement plan at the Naval Ocean Systems Center, Point Loma, resulted in the upland (not streamside) planting of 1,000 irrigated trees and shrubs. In addition, the USDI Forest Service in San Diego County annually installs thousands of seedlings without irrigation. However, these are not riparian system restoration efforts, as drought-tolerant species are planted in upland locations.
Water is a requisite for riparian woodland. Whether on or near the surface, soil moisture must be sufficient to carry the trees and shrubs through summer months and seasonal tropical dry winds. However, even with available water, continued intensive grazing by livestock, followed by repeated flooding can eliminate riparian vegetation and prevent recolonization of the woodland species. The trees give way to grasses which are in turn succeeded by weedy plants. In other
[1] Paper presented at the California Riparian Systems Conference. [University of California, Davis, September 17–19, 1981].
[2] Ronald LaRosa is Associate Water Quality Biologist, San Diego County Department of Public Works, San Diego, Calif.
instances, introduced tree species such as Eucalyptus can out-compete native trees. This combination of factors has led to the loss and degradation of streamside vegetation along most of the Escondido Creek drainage and is particularly evident on county-owned lands in the floodplain east of the San Elijo Lagoon, a regional park and ecological reserve (fig. 1).
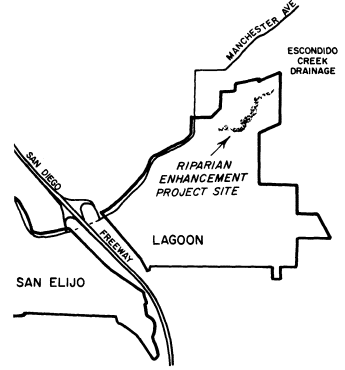
Figure 1.
Vicinity map and proposed project location, San Elijo Lagoon.
Water Resources and Riparian Enhancement
Although there are a number of drainages in San Diego County which could benefit from year-round reclaimed water flows[3] and a wild area landscape plan, Escondido Creek—one candidate area—will be discussed here for the following reasons.
1. The drainage system has the greatest potential to enhance wildlife habitats within an established public park and ecological reserve.
2. The drainage system could accommodate a woodland landscape without jeopardy from flooding.
3. The drainage system could receive surface water from existing and/or proposed wastewater facilities.
4. The drainage system has extensive areas degraded by exotic vegetation, overgrazing, and erosion/sedimentation.
Environmental Design
Although this report discusses the environmental enhancement of a floodplain through water reuse,[4] the interdisciplinary considerations of water quality planning, landscape architecture, environmental planning, hydrologic engineering, and woodland management are recognized.
The plan for the proposed environmental enhancement project would call for the installation of native shrubs and trees and provide year-round surface water to recreate the components of high-quality riparian systems. Figure 2 shows a conceptual schematic of the riparian woodland, including surface water and area subject to planned inundation.
Environmental design considerations in the enhancement plan include, but are not limited to,

Figure 2.
Conceptual schematic plan.
[3] Reclaimed water is the product of domestic wastewater treatment which is suitable for a beneficial/controlled use that would not otherwise occur.
[4] Water reuse is the term used to describe the application of reclaimed wastewater for irrigation, groundwater recharge, industrial uses, recreation, or wildlife habitats.
existing wildlife populations and ecological systems, water resources, health and water quality requirements, hydraulics, and fluvial conditions, as well as funding sources for capital expenditures and maintenance/management. The riparian woodland must satisfy specific biological parameters if it is to provide high-quality habitats for diverse wildlife species. In addition, the woodland must incorporate elements of visual resource management in order to have value as aesthetic open space and greenbelt zone. A summary of these design considerations follows.
Wildlife Habitats
A woodland must include trees and shrubs which provide food (nuts, fruit, nectar, insects), nesting sites (hollows, cavities, etc.), and roosting/perching cover (upper- and under-story branches/foliage), as shown in figure 3. Vegetation must be sufficiently continuous, broad (deep), and dense to provide wildlife corridors or access routes (fig. 4). Trees and shrubs must be grouped to provide optimum habitat by providing a mixture of irregular edges and random openings in proximity to weedy sites and thickets. And last, plants must be irrigated for at least three growing seasons or until they can survive on groundwater and supplemental rainfall.
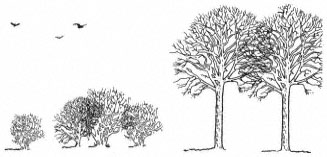
Figure 3.
Wildlife habitats provided by upper-story branches
and understory cover of shrubs and small trees.
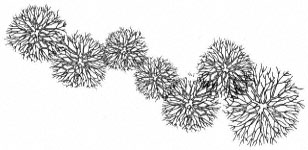
Figure 4.
Wildlife corridor provided by linear vegetative cover.
Visual Resource
Line, form, color, and texture must be provided by plant species; these should include evergreen and deciduous species with erect and procumbent forms, and columnar and round shapes (fig. 5). Groups of trees installed outside of the primary woodland to provide shade for hikers and equestrian riders are designed to encourage use of recreational trails away from sensitive habitats.

Figure 5.
Visual resource criteria include low-growing broad-leaved
species, columnar evergreens, and deciduous round-headed trees.
Fluvial and Hydraulic Systems
In addition to planting trees and shrubs, a low dam is proposed to inundate a portion of the floodplain and create a marshland environment for diverse populations of wildlife including migratory and visiting birds, many of which are threatened species (table 2). Most of trees would be installed outside the floodway and further protected by an earthen bank or berm to avoid loss of irrigation systems and plants due to higher-than-normal flows (fig. 6).
|
Woodland Management
Water, in addition to nominal flows in Escondido Creek, will be required for the success of the proposed riparian enhancement project for the following reasons.
1. Seasonal runoff is subject to rainfall cycles and soil moisture would be insufficient to support the woodland during growing seasons.
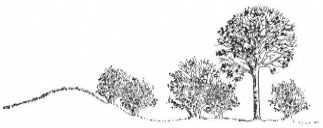
Figure 6.
Landscape profile showing earthen berm
to protect plants from high-water flows.
2. Aquatic systems are dependent upon year-round flows for the maintenance of pools and other surface water.
3. Vegetation "weaned" from irrigation would be augmented by the establishment of more plants.
4. The nutrients in wastewater would provide the vigor for vegetation to overcome the handicaps of characteristically poor soils.
Wastewater Re-Use
A possible source of water to enhance a riparian system and/or create a riparian woodland habitat is the liquid wastes (sewage) processed by a water pollution control facility (WPCF). In the San Diego region, facilities which process liquid wastes and produce an effluent of sufficient quality to be applied for irrigation, wetland replenishment, industrial make-up water, and other uses are called water reclamation plants. Wastewater can be reused if it meets state and local health and water-quality requirements. In the San Diego region, these regulatory agencies include the California Department of Health Services (DHS), State Water Resources Control Board (SWRCB), and San Diego County Department of Health Services. The fundamental intent of wastewater reclamation requirements is to provide guidelines to establish acceptable levels of constituents (chemical, physical, bacterial and other biological properties) and to prescribe processes which insure reliability, such that the reuse of wastewater for specified purposes does not impose undue risks to public health.
Federal and State Regulations
Laws have been enacted to establish requirements for adequate planning, implementation, and management of water quality control. Relevant portions of both federal and state laws, plans, and policies pertaining to water quality planning and control of wastewater discharges are summarized below.
Federal Water Pollution Control Act
The Federal Water Pollution Control Act: 1) sets forth the national strategy for controlling water pollution, including uniform effluent limitations, and requires states to set ambient water quality standards; 2) establishes various levels of water quality planning; and 3) sets up a National Pollutant Discharge Elimination System permit program.
National Environmental Policy Act of 1969
In general, this law directs the preservation of acceptable environments and the restoration of those that have been degraded.
Porter-Cologne Water Quality Control Act
The basic tenor of the Porter-Cologne Water Quality Control Act was set by a policy stating that the waters of the state shall be protected for use and enjoyment by the people of the state, and that activities and factors which may affect the quality of the waters of the state shall be regulated to attain the highest water quality which is reasonable; it further provides that the statewide program for water quality control can be most effectively administered regionally, within a framework of the SWRCB and the nine Regional Water Quality Control Boards (RWQCB). These agencies were established as the principal state agencies with primary responsibility for water quality control. SWRCB is responsible for formulating and adopting state policy for water quality control, including guidelines for long-range planning of groundwater and surface water and the use of reclaimed water. Each RWQCB must formulate and adopt, for its region, water quality control plans establishing such water quality objectives as in its judgment will ensure the reasonable protection of beneficial uses and the prevention of nuisance.
The Porter-Cologne Act addresses water reclamation and reuse through a policy in which the state has a primary interest in the development of facilities to reclaim water to supplement existing water supplies. Anyone proposing to reclaim or reuse wastewater must file a report with the RWQCB. After consulting with the DHS, the board may prescribe water reclamation requirements for either the individual reclaiming the water or the user, or both.
California Water Code
The California Water Code contains provisions controlling almost every consideration of water and its use.
California Environmental Quality Act of 1970
The California Environmental Quality Act of 1970 (CEQA) requires consultation with and comments from any governmental agency with jurisdiction or special expertise with respect to any environmental impact involved.
Water Reclamation and Riparian Systems
A conventional wastewater system collects wastewater in sewers, transports it to a treatment plant, processes it, and then releases it into the ocean or evaporation ponds, or onto land. A reclamation facility instead treats the wastewater to a level suitable for reuse and then conveys it directly to the area of application.
The degree of treatment for reclaimed water depends on the intended use. Regulations covering this are issued by the DHS and are summarized in table 3. Most uses require at least second-level treatment, which is the minimum level required for discharge to surface waters. In addition, reclaimed water often must undergo coagulation and filtration to make it acceptable for many uses.
Although most reclaimed water in California is used to irrigate fodder crops, greenbelts, golf courses, orchards, and vineyards, its use in the creation of woodlands as part of a riparian system is the focus of this discussion.
SWRCB and RWQCB are encouraging reclamation projects which generally improve the beneficial use of water, e.g., those which create a new water supply, preserve recreational and aesthetic resources, or benefit fish and wildlife habitats (California Department of Water Resources 1979). However, reclamation is not universally feasible or practical. Even highly treated wastewater can contain more salts (defined as total dissolved solids [TDS] in milligrams per liter [mg/l] or parts per million [ppm]), nutrients, and other substances than freshwater. Water with high levels (1,000–1,500 mg/l) of dissolved salts or limiting constituents such as boron or heavy metals cannot be used to irrigate most crops. Although many species of riparian trees are salttolerant, water management plans for irrigation must consider salt build-up in root zones, changes in groundwater quality, and other impacts of wastewater reuse.
Reclaimed Water Irrigation Regime
Salts in irrigation water that can harm plants are classified as sodium-containing. Although these salts in wastewater can harm plants and be detrimental to the soil, they can also be removed. To prevent buildup in the root zone, enough water must pass through the soil profile to carry away dissolved minerals. The volume necessary to remove these salts—the leaching fraction, usually 5% to 20% of the applied irrigation—is beyond the quantity needed for plant growth. Although the problems of over-irrigation (loss of nutrients, saturated soils) can be alleviated by the additional nutrients in reclaimed water and the adaptability of most riparian species to water-logged soils, excess irrigation can create downstream impacts, in addition to being wasteful and costly (Dunne and Leopold 1978).
San Diego Case Studies
The San Diego County Department of Public Works has undertaken four limited water re-use projects which, among other objectives, provide reclaimed water to irrigate wild landscapes and woodlands. Although the circumstances of vegetative cover, irrigation volumes, and basin requirements differ among the sites, some general conclusions can be drawn.
Irrigation Uniformity .—More wastewater is generated, and therefore available, during summer months when plant growth and evapotranspiration are greatest. However, the problem of uniformly distributing reclaimed water to plants of varying size and water requirements must be overcome in order to avoid runoff (overwatering) or salt accumulation (underwatering).
Application Efficiency .—In San Diego, discharges of wastewater into moving water are not allowed due to regional health and basin plan requirements (Barry 1978). In some instances maximum use of wastewater is an objective; in every case, the irrigation regime has several goals, i.e., to fill the root zone for plant growth; to provide extra water for leaching salts; and to minimize poor distribution.
|
Salt Management .—Where the protection of groundwater is mandated by health and water quality objectives, irrigation regimes must take into account all sources of infiltration (rainfall, upstream sources, wastewater application) and losses (evaporation, plant transpiration, run-off). The water budget must consider TDS in wastewater, dilution from precipitation, and concentration of salts—in the area of application and downstream—both seasonally and in the long-term (Engineering Science and PRC Tours 1982).
Riparian Woodland Proposal
Project Costs
Expenses associated with the riparian enhancement proposal (table 4) include capital improvements of $82,000 and annual operation and maintenance costs of $18,000. Although conservation projects typically have high operational costs in the short-term, expenses decrease significantly after five years, since plant replacement and major maintenance will have been completed.
|
Woodland Specifications
The overall design and biological parameters of the riparian woodland have been previously discussed. Specifically, the proposed plan recommends that oak and pine species (see table 1) be planted on higher ground in sandy soil, in odd, random groupings of five- and 15-gallon sizes in a ratio of 3:1 respectively. Cottonwoods and sycamores would be planted in both open and dense groupings (at an installation rate of 100 containers per 0.4 ha. [1 ac.]) with a minimum of three-trees width and maximum of 40-trees width in total planting area. Twenty percent of the trees planted should be planted on 1.5-m. (5-ft.) centers to allow crowns to grow into each other (fig. 7). Willows should be installed adjacent to watercourse/surface water. The plan recommends that other shrubs (5-gal. size) be mixed in among edges of tree areas in odd, irregular groupings. Openings in dense plantings would vary from 0.2 to 0.6 ha. (0.5–1.5 ac.).

Figure 7.
Landscape specifications showing irregular groupings; plants
installed to allow crowns to merge, shrubs mixed with trees.
Literature Cited
Barry, Joseph N. 1978. San Diego City/County water reuse study report. 89 p. Department of Public Works, County of San Diego, San Diego, Calif.
California Department of Water Resources. 1979. Water reclamation: State-of-the-Art. Bulletin 189, California Department of Water Resources, Sacramento. 112. p.
Dunne, T., and L. Leopold. 1978. Water in environmental planning. 819 p. W.H. Freeman and Company, San Francisco, Calif.
Engineering Science and PRC Tours. 1981. Orange and Los Angeles Water reuse study. Issue paper on water quality. p. 7–13. In : Water Quality Workshop. [Orange, California, August 7, 1981]. 137 p. Engineering-Science and PRC Tours, Arcadia, Calif.
Sands, Anne (ed). 1977. Riparian forests in California: their ecology and conservation. Institute of Ecology Pub. 15, University of California, Davis. 122 p.
Planting Design Inventory Techniques for Modeling the Restoration of Native Riparian Landscapes[1]
Kerry J. Dawson[2]
Abstract.—Inventory techniques for assessing vegetative distribution patterns in native riparian communities are discussed along with their use and applicability in formulating working drawings for planting design. Such techniques involve a review of historical context and the selection of comparable areas in which to inventory for distribution, community and soil patterns, canopy heights, and elevational transects in relation to streamflow.
Introduction
As native riparian landscapes are increasingly impacted by flood control and water resources development projects, the need has grown for restoration mitigation, not only along existing streams but also on newly created floodways and distribution canals. In the past, governmental reclamation agencies have relied heavily on planting design techniques dependent on exotic plant materials to achieve simplistic goals of erosion control, environmental tolerance (drought tolerance and/or flooding tolerance, soil tolerance, browsing tolerance, etc.), and aesthetic improvement. Today, the use of exotic plant materials is still entrenched in riparian projects. But as more and more managers realize the value and the increasing rarity of ecological diversity that native riparian systems offer, it should be expected that their use will grow.
Cost is more a factor of influence now than ever before as governmental agencies are increasingly required to adhere strictly to cost: benefit ratios. The greater the benefits and the cheaper the cost, the better chance a project has of proceeding. Native riparian communities are certain to benefit from this phenomenon in that the mitigation of habitat loss for endemic species is a much-needed benefit. In addition, native riparian species can reduce installation costs because of their inherent ecological tolerance, fast-growing character, and regenerative efficiency, lacking in ornamentals. Riparian vegetation has evolved under constraints of erosion and streambed stabilization within community associations which allow all species to attain an optimum locale.
Perhaps, though, the largest influence on riparian design philosophy has been a new attitude among the general populace and increasingly among environmental designers toward the management of public lands. This new attitude places less value on engineered landscapes and more emphasis on the aesthetics of native landscapes. This is especially true in the West where native riparian plant communities provide landscape patterning to otherwise homogeneous rangelands and biological diversity to largely evergreen forestlands.
Traditionally, the engineered or manicured landscape used in riparian development projects has represented design based on garden design styles made popular along highways and in city parks (fig. 1). The use of garden design style reflects a concern that native riparian vegetation is too vigorous and will clog channels, and in the first successional stages it contributes a weedy appearance. However, the realities of the engineered style now recognize that as irrigation water grows scarce, the drought-tolerant ornamentals used more and more frequently can rival any landscape for the weedy look. While riparian landscapes can evolve rapidly to lush, attractive woodland, this is definitely not the case with ornamentals. Additionally, riparian landscapes can be installed and managed to minimize channel clogging, while even the best of the low-maintenance engineered landscapes require some clearance management.
General Discussion
There are two primary factors which presently impair full-scale implementation of vegetative restoration for native riparian landscapes: 1) lack of planting design techniques; and 2) lack of management specifications. With the existing
[1] Paper presented at the California Riparian Systems Conference. [University of California, Davis, September 17–19, 1981].
[2] Kerry J. Dawson is Assistant Professor of Landscape Architecture, University of California, Davis, and Landscape Architect, University of California Agricultural Experiment Station, Berkeley.
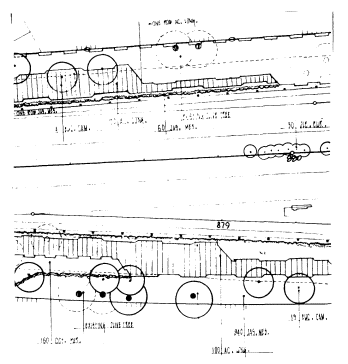
Figure 1.
A classic example of garden design as applied to planting design along
State Highway 30 near Sterling Avenue in San Bernardino, California.
This represents the static rather than the successional approach.
speed at which demands are being made for water resources development and the subsequently astonishing rate at which existing riparian systems are disappearing, the need for rapid growth in the development of restored riparian areas has become crucial for replacement habitat. This has meant that instead of relying primarily on downstream seed dispersal and natural succession, heavily disturbed riparian landscapes must be aided in their development by supplemental seeding or planting to speed succession. In the present construction industry, new types of planting plans and creative specifications in vegetative management to accompany them are called for. Unfortunately, neither concept has been adequately developed.
Planting plans for riparian restoration need to reflect all major components of the ecological system, including some representative water-edge and emergent zones. This means that either the overall engineering context has to be altered for differing circumstances, from small floodways relying heavily on rapid water movement with minimized vegetation in the channel, to larger floodways which temporarily store floodwaters; or the overall concept has to include a pool and channel concept where some areas have completely restored systems while others are partially restored. In either instance, coordinated research and implementation strategies amongst professionals are also necessary for informed, economical decision making.
Of the two factors above, planting design is most limiting in developing riparian restoration because of the uncertainty displayed by landscape architects and other environmental design professionals as to what procedures or guidelines to follow in developing planting design concepts for restoration. With disturbance dynamics as the population ecology norm for riparian systems, the question of what is the proper successional pattern for a severely degraded situation has always been difficult to answer. And, unlike the easily definable standards for ornamental design, standards have not been developed for simplifying riparian planting design criteria.
Historical Context
To begin to develop standards, it seems obvious that the historical contexts which have controlled the parameters of existence for native systems need to be investigated. First, the study site should be categorized in relation to to its physiographic province. Suppositions can then be made as to past plant community relationships and the respective ecological niches for species of special interest. This can be accomplished by studying relic evidence if the site has been historically riparian or, if adequate on-site information does not exist, by studying comparable areas.
In California, there are six basic "ecoregions" for riparian modeling (as adapted from Griffin and Critchfield 1972 and Walters etal . 1980). The first three can be thought of as "subregions" of the western Mediterranean or California physiographic region. They are the California grassland ecoregion, chaparral ecoregion, and the Sierran forest ecoregion. The fourth ecoregion is the northwest ecoregion associated closely with Oregon ash and the northern species. A miscellaneous or mixed community occupies the fifth ecoregion and occurs irregularly in isolated or eastern slope communities dominated by continentals. The sixth ecoregion is restricted to the low desert and is typified by the seasonal wash.
After physiographic classification by eco-region, historic research into development patterns as they relate to the study site is essential. In "Riparian Forests of the Sacramento Valley" (Thompson 1961), a lengthy discussion on the pristine condition of the riparian lands of the Sacramento Valley is found. The vivid accounts of early fur-trappers, explorers, botanists, military expeditions, and surveyors are surprisingly detailed and site specific. For records before European settlement in California, researchers have had to rely heavily on fossil flora. In the "Geologic History of the Riparian Forests of California" (Robichaux 1977), such fossil records are combined to reveal specific information on the existence of riparian forests over the past 20 million years which remained basically unchanged until today.
During this century, the best historical records of land-use change in riparian communities have come with aerial photography and remote sensing. Accurate inventories of in-place resources can be made, and change through time can be assessed. A good example of application of this method is provided by the studies by McGill (1975, 1979) on land-use changes along the Sacramento River riparian zone from Redding to Colusa during the period 1952 through 1979.
On-Site and Comparable Area Inventories
In degraded situations where historical information is insufficient to formulate a design format, the use of comparable areas may be necessary to guide the planting plan. When relatively natural conditions exist upstream or downstream of a study site, these areas will prove valuable for comparison. This was the practice utilized by the Santa Clara Valley Water District in riparian revegetation completed on previously excavated earth channels where natural succession was encouraged. Comparative information for study sites came almost completely from upstream or downstream areas.
The best comparable areas are those that have been least disturbed and managed as natural areas. Such areas often have previous research which can save time and survey expenses. Examples of these areas include Caswell Memorial State Park on the Stanislaus River[3] and The Nature Conservancy's recently purchased Kern River Preserve. In comparable areas where previous research on community distribution is not available, studies to determine community and soil patterns, canopy heights, and elevational transects are necessary. These studies relate to the common formats of working drawings in landscape architecture by emphasizing the base (horizontal) plan and transect (vertical) elevation.
Base Plan Development
Base plan development first involves selecting a sample plot within the study site or a comparable area where homogeneous distribution of characteristic species occurs. Next, the designer must determine whether to utilize random or non-random species sampling for the distribution studies. Random sampling is usually used where the site is large[4] and/or statistical analysis is desirable. The random technique most commonly employed is the gridding of quadrants over the site with random sampling combinations used to ascertain sub-plots (fig. 2).
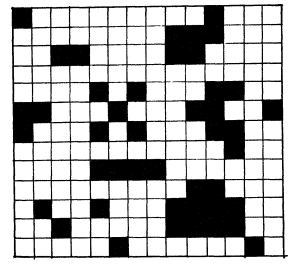
Figure 2.
The grid above is an example of random pairing of quadrants
for the sampling and statistical analysis of spatial patterns.
Frequency of species occurrence in the quadrants is the
basis for the analysis (adapted from Goodall 1974).
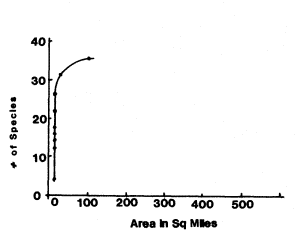
Figure 3.
When the curve in the graph begins to flatten, enlarging
the study site area will result in fewer additional species.
In the instance of riparian systems, so few species hold
fidelity on the high terraces that sample plot expansion
should be streamside (adapted from Kershaw 1964).
Non-random sampling implies the inventory of the whole plot and is used when ecological accuracy is the foremost consideration. If cost and time are major factors, statistical analysis difficult, and the plot size greatly enlarged due to limited species lists, carefully selected (but non-random) subplots might suffice.
Vegetative association is the primary classification arrangement for determining base-plan design patterns. The characteristics of an asso-
[3] Great Valley Museum. Undated. Flora and fauna of Caswell State Park, Ripon, Calif. Regional Biota Series, No. 1. Modesto, Calif.
[4] The site size is usually determined by enlarging the plot until the species list gets progressively shorter (fig. 3).
ciation rely primarily on the totality of homogeneous releves (lists) of species which relate floristically to one another in the plot. Associations should, however, also be characterized by geography, dynamics, and ecological niche (Kershaw 1964) (fig. 3).
The indicators for riparian associations depend primarily on one or two high-fidelity species. High fidelity can be thought of as faithfulness to a relatively narrow ecological niche. Other species may have wide ecological tolerance and occur in several associations, while still others may have such a limited tolerance that true consistency of association is not possible (plants on the limit of their range for example). Degree of faithfulness to a relatively narrow ecological niche is the key.
Patterns are drawn by placing an enclosing two-dimensional shape on the base map; this shape outlines individual species in primary association (fig. 4).[5] The actual location of the enclosing line is determined using either a multi-dimensional or hierarchical clustering method for correlation. The multi-dimensional method is controlled by positive associations detected between pairs of species, where distance is directly related to degree of association between them (ibid .). On the other hand, the hierarchical method relies on species clustering by descending order of importance (dominance rather than distance). Hierarchical clustering is generally considered an oversimplification (Webb 1954); while multi-dimensional clustering is considered the most applicable to patterning for design.
Soil types and topography can be mapped separately or as overlays to vegetative associations. Information usually comes from existing soil surveys or from field data collection. As with vegetative association, soil bores are also clustered by similarity and location.
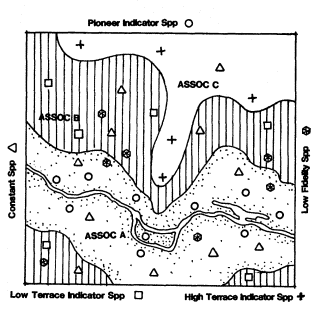
Figure 4.
After location symbols have been placed on the base map
for all individual species locations, the enclosing line for an
association should form a continuous and flowing line based
on inclusion of only those individuals and species in close
proximity to the key indicator species. Some species will
occur constantly throughout all associations so judgments
based on fidelity to ecological niche are essential.
Transect Elevation Development
Transect elevations can be placed randomly or non-randomly within the study site or comparable area. They profile the plot in representative locations for topography, soil depth, canopy height, and vegetative association. As with the base plan, the random selection of transects would apply mostly to a large plot where statistical analysis is desirable. Non-random sampling, on the other hand, would be most acceptable on smaller plots and where the quality of the base map has been sufficiently detailed.
Two basic forms of delineation are possible for transect elevations. The first is the histogram of occurrence for individual species; it compares percentage of cover to horizontal distribution along the transect (fig. 5). This inventory technique is very valuable for quantifying plants along the transect.
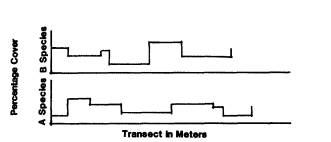
Figure 5.
An example of a histogram of occurrence (as adapted from Kershaw 1964).
The second transect form is the profile diagram. The profile diagram identifies individual plants along the transect, shows stratification of canopy, which adds a third dimension to the base map, and delineates soil depths and topographical relationships (fig. 6). In addition, if wildlife habitat loss mitigation and ecological
[5] An important point here is that the survey to locate individual species is the foundation for clustering. This information typically is accumulated through field work although remote sensing, especially with infra-red photography, is growing in popularity because of the time and expense involved in field work.
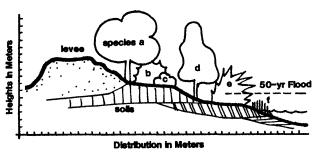
Figure 6.
Profile diagrams indicate a variety of design factors including
canopy height, species niche, vegetative structure, and elevational
relationships (topography, water levels, and soil depths).
stability are the foremost considerations for the project, crown density, basal area, vigor, and stand structure (age) should be added as inventory components (Davis 1977).
Interpreting Planting Design
Design programs for developing a planting plan in riparian restoration usually include a number of structural decisions which are the true test of the designer. Included in these decisions are such program items as fire lane access, levee arrangements, channel alterations, and recreational facility locations. All of these program items should make use of planting as options in design. Unfortunately, this often does not often happen. As an example, gabions can often be combined with willow cuttings but are not, and willow mats will easily substitute for jute mats and nets. Normally, with either of these treatments (gabions and nets), vegetation has difficulty colonizing engineered channels because bare earth is not available. Most structural design decisions have to be made in relation to planting design. What erosion control is necessary, and what structures are appropriate (cribbing, bermed willow rolls, gabions, etc.)? Will recreation be non-structural or are facilities required, and if so, for what activities? The list of program needs can go on, and all will have some relationship to planting.
The basis for the planting plan must be successional pathways. Although highly statistical pathways can be established for most landscapes (Cattelino etal . 1979), riparian design can be simplified to three major successional stages. These are: a) the pioneer stage, usually associated with surface water and freshly exposed sand- and gravelbars dominated by willows; b) the low terrace, usually the moderately wet bottomland dominated by cottonwood; and c) the high terrace, the somewhat drier, infrequently flooded floodplain component, dominated by oak and other more drought-tolerant mesic species.
With succession as a primary design determinant, another determinant should be vegetative structure. Modelled vegetative structure designed into plans is crucial for habitat restoration. With western riparian systems serving as the principal corridors of biological diversity in an increasingly man-altered landscape, they offer the only habitat for both native wildlife and native plants (Dawson 1981).
As shown in figure 7, designers have been thinking about the evolving landscape since the English country gardens of the 1800s. What is new is the commitment to ecological integrity and the hopeful development of accurate native landscape survey methods to model design decisions. With a new commitment and tools available for
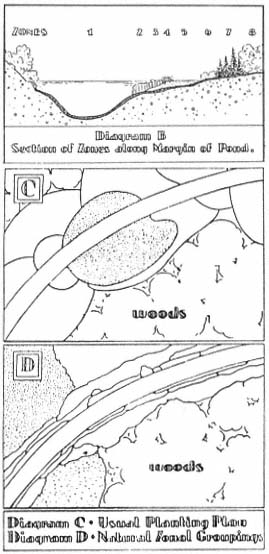
Figure 7.
These sketches represent early 20th century work in
interpreting natural succession for design (Waugh
1931). Diagram B (upper drawing)—section of zones
along margin of pond. Diagram C—usual planting
plan. Diagram D—natural zonal grouping.
implementation, surely the minimum levels of mitigation measures necessary to satisfy CEQA are within our grasp.
Recently, methods outlined in this paper were used to complete planting design working drawings for riparian forest restoration along a two mile stretch of the Sacramento River near Interstate 5. In addition to vegetative modelling, endemic avian species were surveyed for preferred vegetative structure as a habitat restoration success indicator. The project will go to bid for installation in 1984. Copies of research methods will be available through the author during 1984.
Acknowledgments
Special thanks to Landscape Architecture Magazine Archives for the use of the Waugh sketches.
Literature Cited
Cattelino, Peter J., J.R. Noble, R.O. Slatyer, and S.R. Kessell. 1979. Predicting the multiple pathways of plant succession. Environmental Management 3(1):41–50. Springer-Verlag, New York, N.Y.
Davis, Gary A. 1977. Management alternatives for the riparian habitat in the Southwest. p. 59–67. In : R.R. Johnson and D.A. Jones (tech. coord.). Importance, preservation and management of riparian habitat: a symposium. [Tucson, Ariz., 9 July 1977.) USDA Forest Service GTR-RM-43. Rocky Mountain Forest and Range Experiment Station, Fort Collins, Colo. 217 p.
Dawson, Kerry J. 1982. Bioregional landscapes as frontier. p. 208–210. 1982–83 yearbook of the International Federation of Landscape Architects. IFLA Secretariat, Versailles, France.
Goodall, David W. 1974. A new method for the analysis of spatial pattern by random pairing of quadrats. Vegetatio 29(2): 135–146.
Griffin, James R., and William B. Critchfield. 1972. The distribution of forest trees in California. USDA Forest Service Research Paper PSW-82. (Reprinted with Supplement, 1976.) Pacific Southwest Forest and Range Experiment Station, Berkeley, Calif. 118 p.
Hackett, Brian. 1979. Planting design. McGraw-Hill Book Company, New York, N.Y. 174 p.
Kershaw, Kenneth A. 1964. Quantitative and dynamic ecology. Edward Arnold (Publishers) Ltd., London, England. 183 p.
McGill, Robert R., Jr. 1975. Land-use changes in the Sacramento River riparian zone, Redding to Colusa. 23 p. California Department of Water Resources, Northern District Report, Sacramento, Calif.
McGill, Robert R., Jr. 1979. Land-use change in the Sacramento River riparian zone, Redding to Colusa. An update—1972 to 1977. 34 p. California Department of Water Resources, Northern District, Sacramento, Calif.
Robichaux, Robert. 1977. Geologic history of the riparian forests of California. p. 21–34. In : A. Sands (ed.). Riparian forests in California: their ecology and conservation. Institute of Ecology Pub. 15, University of California, Davis. 122 p.
Thompson, Kenneth. 1961. Riparian forest of the Sacramento Valley, California. Ann. Assoc. Amer. Geogr. 51(3):294–315.
Walters, Alice M., R.O. Teskey, and T.M. Hinckley. 1980. Impact of water level changes on woody riparian and wetland communities. Vol. 3, FWS/OBS-78/93. USDI Fish and Wildlife Service, Washington, D.C. 55 p.
Waugh, Frank A. 1931. Natural plant groups. Landscape Architecture 21(3): 169–180. Landscape Architecture Publishing Co., Boston, Mass.
Webb, D.A. 1954. Is the classification of plant communities either possible or desirable? Saer. Dot. Tidssk. 51:362–370.
Irrigation Systems for Riparian Zone Revegetation[1]
John Disano, Bertin W. Anderson, and Robert D. Ohmart[2]
Abstract.—Revegetation of aridland riparian zones with native riparian species is feasible, but generally requires initial irrigation to maintain the plants while root systems are established. For most desert riparian revegetation work, the irrigation pump should be gasoline- or diesel-powered. Main irrigation lines should be PVC (polyvinyl chloride) buried about 31 cm. (12 in.). Laterals should be polyethylene tubing because this can safely lie on or near the surface. The water should be delivered to each tree through pressure-compensating emitters.
Introduction
We have been reintroducing native riparian species of trees and shrubs on an experimental basis along the lower Colorado River riparian zone since 1977. In this region, irrigation is a necessary prerequisite to any successful revegetation effort. Irrigation is critical in getting vegetation root systems established; it is also a major expense associated with revegetation efforts. In our work we have used two different systems. One was established in 1978 on a barren dredge-spoil area of about 30 ha. (75 ac.); the other was established on a 20-ha. (50-ac.) plot from which salt cedar (Tamarixchinensis ) had been cleared. Both systems delivered water to 2,500 plantings and included about 19 km. (12 mi.) of pipe. In this report, we describe and evaluate the major systems with which we have had experience. Advantages and disadvantages of each system are noted and recommendations are made for designing irrigation systems for revegetation. English units of measurement are used in order to be consistent with units currently in use in the irrigation industry.
Dredge-Spoil System
The irrigation system on the dredge-spoil revegetation site was assembled from polyvinyl chloride (PVC) irrigation pipe. A well was drilled that reached water at 3 m. (10 ft.); water from this well contained approximately 1100–1400 ppm total dissolved solids. A Worthington Model 8M-28 electric pump developing 20 hp at 1760 rpm was installed on the well (fig. 1). Pump bowls were set at 50 ft. The pump had a 5-in. suction pipe and a 6-in. discharge pipe. Water was delivered from the pump through an Olson-Filtomat Model FLT-3000 vacuum cleaning filter with a capacity of 250 gpm (fig. 1). This filter is an automatic backwash filter with a hydraulic controller which detects pressure differential between the intake and outlet valves of the filter. From the filter, water entered a pressure-regulating valve (fig. 1) which was set to maintain 35 psi in the irrigation system. Before entering the main line, water passed two pressure-sensitive electrical cutoff switches (fig. 1). One switch was set at a high pressure of 40 psi, the other was set at a low pressure of 30 psi. Any time the pump was running and the irrigation system control panel was set on automatic, an increase or decrease in pressure to the above settings automatically broke the electrical

Figure 1.
Dredge-spoil pump station.
[1] Paper presented at the California Riparian Systems Conference. [University of California, Davis, September 17–19, 1981].
[2] John Disano is Research Biologist, Bertin W. Anderson is Faculty Research Associate, and Robert D. Ohmart is Associate Director; all are with the Center for Environmental Studies, Arizona State University, Tempe, Arizona.
circuit and turned off the pump. These automatic cutoff switches protected the integrity of the irrigation system in the event of a failure of the pressure-regulating valve or a major break within the irrigation system.
The main body of the irrigation system consisted of a 750-ft. long, 6-in. PVC main line running from the pump station to the Colorado River (fig. 2). At the river a butterfly valve was installed along with a bypass pressure relief valve. The entire capacity of pumped water could be discharged into the Colorado River with the butterfly valve open; there was no discharge if the valve was closed. The bypass pressure relief valve was a final safeguard to protect the irrigation system. If all other safeguards failed, the value would open at 50 psi and bleed water into the Colorado River, thereby relieving pressure on the system.

Figure 2.
Map of the irrigation system, dredgespoil site.
Seventeen laterals, each 2,000 ft. long, were installed north of the main line; 17 laterals, each 1,500 ft. long were installed south of the main line. The main line and all lateral lines were buried 15–20 in. Risers for irrigation were placed every 20 ft. along laterals (fig. 3).
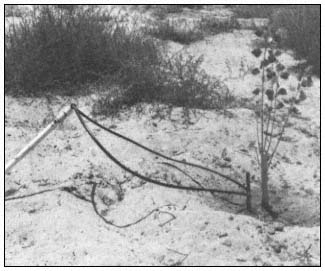
Figure 3.
Riser with Subterrain Dual Flapper emitter and tubing.
The emitters used to deliver water to the trees were Subterrain Dual Flappers. These emitters are pressure-compensating and delivered 4 gal. per hour with a system pressure between 15–50 psi. Tubing was attached at each end of an emitter to deliver a point source of water to the base of a tree (fig. 3).
With the dredge-spoil irrigation system in full operation, the pump delivered 167 gal. per minute at a system pressure of 35 psi. This pressure was chosen because the last emitter along a 2,000-ft. lateral still delivered a pressure of 18–20 psi, while the first emitter near the main line had a pressure of 35 psi. Therefore, both emitters were within the recommended 15–50 psi, and each delivered 4 gal. per hour to its respective tree.
Another pertinent point concerning the irrigation system is that the main line could be opened or closed by a butterfly valve at the well or the river, thus permitting irrigation water to be supplied from the well or from the Colorado River by an auxiliary, trailer-mounted pump. Any one or more laterals could be shut off at the
main line by turning a shutoff valve. Also, any one or more of the 2,500 emitters could be capped temporarily or permanently to prevent further irrigation of trees.
A major disadvantage of this system is that it is expensive to purchase and to install. Purchase and installation costs amounted to $34 per tree.
Refuge Site
The irrigation system on the refuge site received water from the lower Colorado River. This water contained 600–800 ppm total dissolved solids. The water was delivered to the revegetation site by the Cibola National Wildlife Refuge concrete-lined canal (fig. 4). A jack gate was installed (fig. 4) to divert water into two intake ports (fig. 5) of a holding pond (fig. 6). In addition to providing a seven day supply of water for irrigation, the pond also served as a settling basin. This was important because a drip irrigation system using microtubing or emitters with small orifices may be blocked or damaged by dirt or sand.
Water was pumped from the pond using an 8-hp., two-cycle Wisconsin-Robbins engine with a centrifugal pump (fig. 7). This pump delivered 90 gal. per minute at 20 psi to the main line.
A prescreen filter, made from a 3-ft. section of 6-in. PVC pipe with vertical slits and with the open ends covered with fine mesh wire, was fastened to the intake hose and suspended from a barrel float in the pond (fig. 8). The purpose of the prescreen filter was to prevent leaves, sticks, and other debris from being drawn into the pump. A Yardney Model-8 Free Flow manually cleaned filter was installed between the pump and main line (fig. 9). This filter had a capacity of 125 gal. per minute.
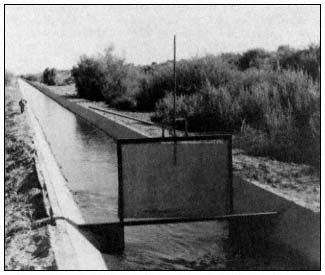
Figure 4.
Cibola National Wildlife Refuge, concrete-
lined irrigation canal and jack gate.

Figure 5.
Intake ports in canal.

Figure 6.
Holding pond at low-water level to show intake ports.

Figure 7.
Engine and pump.
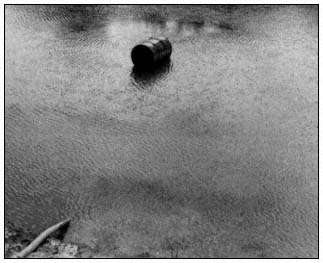
Figure 8.
Barrel float in pond.
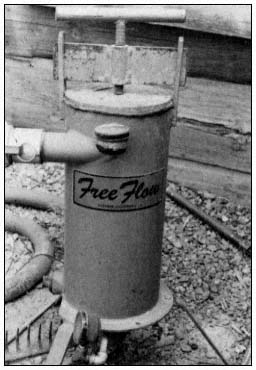
Figure 9.
Free Flow filter.
The irrigation system on the refuge was assembled using regular 3-in. diameter aluminum sprinkler irrigation pipe for the main line transport of water. The laterals were flexible black polyethylene tubing with an inside diameter of 0.58 in. The maximum length of any lateral was 750 ft; none of the pipe was buried.
The site was divided into four 750- x 530- ft. sections (fig. 10). Water to each section was supplied by 17 lateral lines; each lateral supplied 38 trees. The main line of 3-in. diameter aluminum sprinkler pipe was 1,500 ft. long, with the pump located at the center. The north and south submains were both 530 ft. long.
The installation of two 3-in. gate valves (fig. 11) in the main line near the pump permitted the watering of either the north or south section separately or simultaneously. Further flexibility in design was incorporated by installing a ball valve (fig. 12) on each lateral so that any one or more laterals could be shut off during irrigation.
Each lateral valve assembly contained one Subterrain pressure regulator (fig. 12), which prevented main-line water from entering the lateral at more than 20 psi. This was to prevent bursting of laterals and blowing out of microtubes due to a pressure surge; it also assured equal distribution of water to all 68 laterals. The pressure at the beginning of a lateral was 20 psi; pressure at the end was 8–10 psi.
Each tree was watered by two microtubes (fig. 13), each delivering water at a rate of 1 gal. per hour. Any individual tree could be cut off from watering by removing the microtubing and installing "goof" plugs. In order to provide 1 gal. per hour delivery from each microtube, microtube length was varied as shown in figure 10. Appropriate microtube lengths were determined by a computer using the flow characteristics of the system.
Purchase of the system and installation costs associated with this system were much less than that for the dredge-spoil system, amounting to $3 per tree. However, maintenance costs ($16 per tree) were higher than for the dredge-spoil system ($11 per tree).
Evaluation of Irrigation Systems
In comparing the dredge-spoil irrigation system with the refuge irrigation system, there are several factors to consider. One important factor is the availability of electricity. In most areas along the lower Colorado River, electricity is not available. If a system based on electrical pumping is necessary, then revegetation efforts would have to be limited to areas where electricity is available. In most of these areas there is likely tobe competition for land from farming or some form of urbanization. Cost of pumping with electricity is comparable to that for gasoline-powered pumping, but the cost of an electrical pump is about 20 times greater than for a gasoline pump. Locations for a gasoline or diesel-powered pump are limited only by the availability of free-flowing or well water.
A second important consideration is that flowing water is preferable for irrigation if total dissolved solids are low enough to permit plant growth. Water from a well has anaerobic bacteria; the combination of their chemical reactions with those of aerobic bacteria in irriga-

Figure 10.
Refuge site, map of irrigation system. Note: for
0.035 I.D. microtube, use various lengths as shown.
North side of the field is the same as the south side.
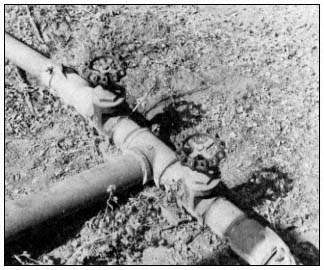
Figure 11.
Gate valves.
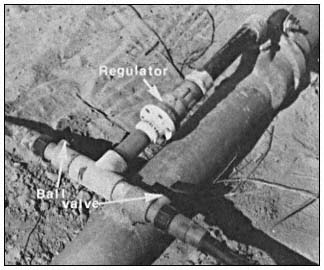
Figure 12.
Lateral valve assembly.
tion systems produces an orange or rust-colored precipitate that must be continuously flushed from lines and/or emitters. This problem usually does not occur when free-flowing river water is used for irrigation.
PVC pipe must be buried because of algae development and deterioration of the pipe when exposed to sunlight. Burying pipe is both labor-
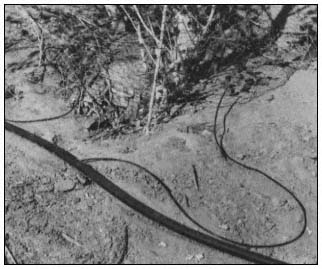
Figure 13.
Microtubing at tree.
intensive and expensive (Anderson and Ohmart 1981[3]) . Black polyethylene tubing accumulates very little algae and can be laid on the surface; in addition, it can be picked up and moved to another area relatively quickly. Also, if polyethylene tubing is damaged it can be repaired easily and cheaply on the surface. PVC pipe is about 10 times more costly than polyethylene tubing.
The decision of whether to use the very inexpensive microtubing or pressure-compensating emitters is more difficult. Although emitters would initially cost six to eight times more than microtubing, they last longer and provide more uniform water distribution over natural, sloping terrain. Also, the cost of labor for installation of emitters is about one-half that of microtubing.
Recommendations
For irrigating most desert riparian revegetation areas, the pump should be gasoline- or diesel-powered and should pump free-flowing water. For flexibility and durability, PVC pipe should be used in main irrigation lines; it must be buried in the soil about 12 in. Lateral lines should be polyethylene tubing with pressure-compensating emitters.
Acknowledgments
We wish to thank Susan M. Cook, Jane R. Durham, Dr. Julie K. Meents, and Cindy D. Zisner for editorial assistance. Marcelett Ector and Cindy D. Zisner typed the numerous drafts of the manuscript. We are grateful to Dr. F. Aljibury, Les Ede, and Jule Meyer, University of California Agricultural Extension Service, Riverside, for their advice and cooperation. James Moore and Judy Huff prepared the figures. Our work was supported by USDI Bureau of Reclamation and the USDI Fish and Wildlife Service Contract No. 7-07-30-V0009.
[3] Anderson, B. W., and R. D. Ohmart. 1981. Chapter 5. In : Revegetation efforts along the lower Colorado River. Final report, in preparation, to USDI Bureau of Reclamation, Boulder City, Nevada.
High Mountain Meadow Restoration[1]
David H. Clay[2]
Abstract—A technique using a rock sill and vegetation to stabilize streams in high mountain meadows which will enable restoration of these riparian systems is discussed.
Introduction
The USDA Soil Conservation Service (SCS) believes strongly in the use of riparian vegetation for erosion control, wildlife habitat development, and improving the aesthetics of artificial and natural waterways.
From an engineering standpoint, the use of vegetation for an erosion control system has both advantages and disadvantages. Vegetation is not as predictable as concrete and other materials which engineers normally consider when they are designing waterways and erosion control systems. Since water velocities must be kept at lower levels when vegetation is used, greater channel cross-sectional areas are generally needed. This may be very costly where land values are high. Maintenance of the vegetation must be provided on a regular basis if channel capacities are to be maintained, and damaged sections must be cared for on an annual basis.
On the positive side, vegetation can provide channel stability which is aesthetically pleasing, provides wildlife habitat, recreational benefits, low initial cost, and maintenance which can be done by private landowners. The determination as to whether vegetation can be successfully used is dependent on the situation at the time of design and what is expected in the future. Some of the factors which should be considered are listed below.
1. Is there sufficient area available at a reasonable cost to provide adequate capacity at an acceptable level of maintenance?
2. Are climatic conditions suitable to establish and maintain the desired vegetation?
3. Are structural measures needed to provide an adequate level of protection and maintain the vegetation?
4. Has sufficient maintenance been provided for?
Soil Conservation Service Role
The SCS works primarily with individuals and groups of landowners on their own lands. Cooperation between SCS and the landowners is on an entirely voluntary basis. In order for SCS to be effective, it must provide the landowner with a workable and economically feasible solution to his problems. In the case of developing stable waterways, the work proposed must be economically feasible and within the landowner's maintenance capability. Riparian vegetation often meets these requirements.
An example of this is demonstrated by the use of rock grade-control structures to establish vegetation along eroding natural channels in the arid areas of northeastern California and northwestern Nevada. Although the work is structural in nature when it is built, the long-term solution will be accomplished by vegetation. Recognition of this philosophy usually leads to success of the project.
Wet Meadow Restoration Procedures
Historically, wet meadows have been drained, as a result of stream erosion (usually brought about by livestock grazing) forming gullies 1–2 m. (3–5 ft.) deep. These have eroded in an upstream direction through the meadows. Lowering of the water table changes the moisture regime so that native grasses and other meadow plants can no longer survive. With loss of vegetative cover, erosion is accelerated, and eventually the entire area will be converted to bare ground or brush.
The normal configuration of eroded gullies in the area is one with vertical sides and a relatively flat bottom. During spring runoff, the vertical banks are undercut, causing large
[1] Paper presented at the California Riparian Systems Conference. [University of California, Davis, September 17–19, 1981.]
[2] David H. Clay is Resource Conservation and Development Project Coordinator, Soil Conservation Service, Alturas, Calif.
portions of the bank to fall into the stream and wash away. The channel continues to deepen and widen until the meadow is entirely destroyed.
In order to bring this condition under control, the procedure described below has been very successfully employed.
1. Slots are cut across the channel using a bulldozer or backhoe. These slots extend into the bank approximately 3.3 m. (10 ft.) and into the bottom about 1 m. (3 ft.). Location of the slots is determined by channel gradient and configuration.
2. Large rock is dumped into the slots and then shaped so that it has a level crest and sides with slopes no steeper than 2:1 horizontal to vertical. This forms a sill across the channel. The rock should extend at least 0.3 m. (1 ft.) above the natural ground on each side of the channel.
3. Soil excavated from the trench is placed on the upstream side of the sill to prevent water from passing through the rock.
4. Low earth fills are placed a short distance out from each side of the rock sill to prevent water which exceeds the channel capacity from flowing overland immediately against the rock shoulder. Spillways are left at the end of each fill at ground level.
5. All disturbed areas are planted with suitable vegetation. It is desirable to plant willows or other woody shrubs into the rock on each abutment of the sills.
6. The entire area is fenced when necessary to prevent indiscriminate use by livestock. Such fencing should be designed to provide access to water by livestock and have a smooth bottom wire in areas where there are antelope. It is sometimes desirable to use controlled grazing of livestock inside the fenced areas to spread and cover seed when re-establishing sod along and in the waterway.
Generally two to three years after this work is completed deposition has filled the channel upstream from each sill to its crest, raising the channel bottom 0.7–1 m. (2–3 ft.). This raises the water table in the meadow, sometimes to original elevations. The sides of the channel develop a slope due to the action of the water. This slope is sufficiently flattened to let grass and shrubs become established. Sod forms on the low earth fills to a degree that prevents damage from the spring overflow. The sills themselves are almost unnoticeable.
Figures 1 through 4 illustrate this process as it occurred on Willow Creek near Adin, Modoc County, California.
Costs for installation of a typical loose rock grade-stabilization structure are $700 to $1,000.
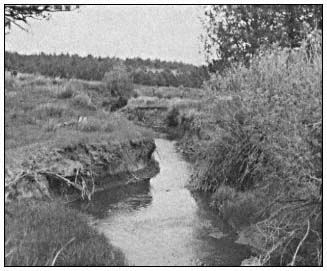
Figure 1.
Willow Creek near Adin, Modoc County, before
installation of rock sill. Note recent and continuing
bank erosion.
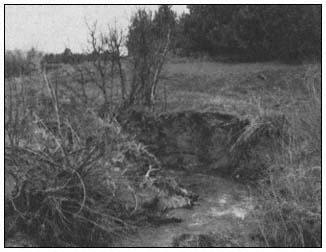
Figure 2.
Willow Creek, May 1980, before
erosion control device installed.
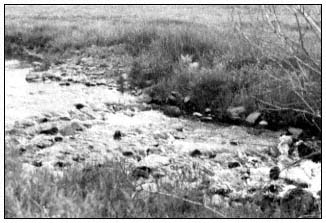
Figure 3.
Willow Creek one year after rock sills were installed.
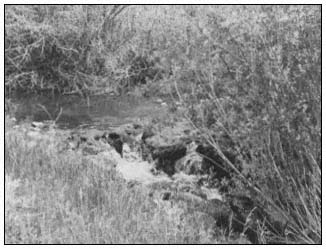
Figure 4.
Willow Creek one year after rock sills were installed.
Sediment has now filled eroded channels.
At this point the success of the program is dependent upon management. Under proper grazing management, the fencing can be removed and the meadow can be maintained in a stable condition for an indefinite period.