Seven
Global Climate Change and California's Natural Ecosystems
Daniel B. Botkin, Robert A. Nisbet, Susan Bicknell, Charles Woodhouse, Barbara Bently, and Wayne Ferren
Nationally, there are many reasons why considerable attention should be focused on the impact of climatic change on California: it has great biological diversity, many endangered and threatened species, a number of important national parks and national forests, and its forests and wildlife are a familiar symbol worldwide for biological conservation (see fig. 1 for a representation of present California ecosystems).
If projections of global climate models are correct, the natural ecosystems of California might undergo major changes during the next century. Such changes might include large economic losses in timber, fisheries, and recreation; major changes in our national and state parks and forests and in our nature preserves and conservation areas; increase in extinction of endangered species; loss of large areas of existing habitats; and development of new habitats whose location and areal extent can only be surmised. Many areas currently set aside for the conservation of specific ecosystems might no longer be suitable to them. Yet, in spite of the potential seriousness of these problems, which could dwarf all other environmental changes, California is at present in a poor situation to project what the effects of global change on its natural ecosystems might be.
Most considerations of the ecological impacts of global warming have focused on long-term consequences, but we must be alert to transitional effects that could last decades, during which natural ecosystems would undergo large-scale disruptions. We know the most about and are in the best position to make projections for forest vegetation and coastal oceans and wetlands, but even for these areas projections remain informal and qualitative. For example, while it may be true that one forest type will be replaced over the long run by another, existing forests might decline before warmer- and drier-adapted species are able to immigrate and
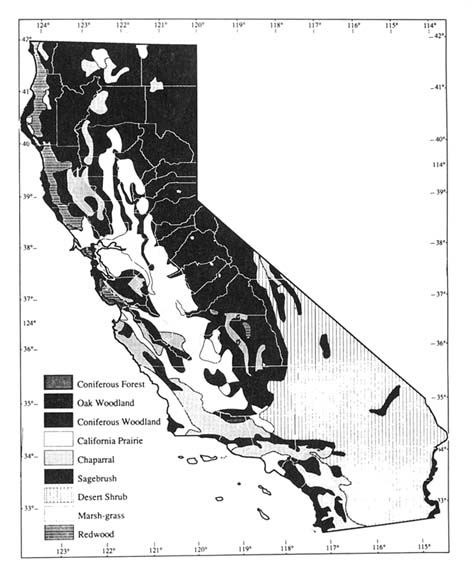
Figure 1. Natural vegetation zones in California. (Map courtesy of Bern Kreissman,
California: An Environmental Atlas and Guide [Davis: Bear Klaw Press, 1991])
become established, and there may well be a period of tree die-offs and a temporary loss of habitat for certain wildlife species (Davis 1989). Species with restricted ranges and specialized environmental requirements, such as the giant sequoia, might be caught in ecological "islands" without sufficient contiguous refuge areas where seeds could migrate and germinate. The very long-lived sequoia and redwood might persist through
this transition, but most woody vegetation would be severely disrupted. Increased temperature and decreased soil moisture might lead to an increase in fire frequency, accelerating vegetation changes and in the long run leading to a decrease in forest biomass. If rapid climate change were to continue past the twenty-first century, forests as we know them might no longer be sustainable, since a tree seed that might germinate in a suitable climate in one decade might reach maturity in a climate no longer suitable to its growth or the germination and survival of its seeds. If such conditions were to occur, only the shortest- and longest-lived tree species might persist. Trees common over much of California's landscape today might be no longer viable.
While it is clear that the natural ecosystems of California will undergo alterations, our ability to make quantitative projections about the impacts of climate change lags behind that for the eastern United States. Projections made for the forests of eastern North America suggest that the anticipated climate changes will lead to dramatic and surprisingly rapid ecological alterations, including large-scale changes in the distribution of major species of vegetation, with some effects observable by the turn of the century or soon after (Botkin and Nisbet 1990; Smith and Tirpak 1989). In part, our inability to make similar predictions with confidence is due to California's multitude of physiographic regions and its complex flora and fauna; but it is also due to the slower pace of ecosystem research and the development of adequate ecological models for California ecosystems. At this time we are lacking in our ability to make projections necessary to the development of wise policy for the management of many natural ecosystems, endangered species, and many commercially important renewable biological resources . The best we can do is to point out likely qualitative responses of natural ecosystems to global warming. The challenge that faces us is thus threefold:
|
Marine Ecosystems
What We Know about Marine Ecosystems
Our general knowledge of oceanic ecosystems can be applied regionally to develop an understanding of the processes that control these systems
and their response to environmental perturbations. The ocean is the world's largest reservoir for carbon. It exists there in the form of dissolved CO2 , carbonate and bicarbonate, CaCO3 in sediments, and as dissolved organic carbon (DOC). The ocean is also a very large heat reservoir. To a very great extent the consequences of adding more CO2 to the atmosphere depends on how the ocean responds to a change in global heat gradients and therefore the wind-driven current systems. These current systems regulate levels of primary productivity by recycling plant nutrients. It is this productivity that results in the vast areas covered by CaCO3 sediment and the enormous pool of DOC.
Ocean Monitoring . The ocean along the California coast has been monitored on a regular basis since 1949 by the California Cooperative Oceanic Fisheries Investigation (CalCOFI). This program has been in place during two major and a number of lesser warming events termed El Niño Southern Oscillation (ENSO). Consequently an extensive database exists, on which marine scientists can draw to formulate educated speculations on the consequences of meteorologic and oceanographic warming trends in California. ENSO events are usually signaled by an increased atmospheric pressure difference in the southern Pacific, leading to an intensification of winds and subsurface temperature along the equator, propagating northward. Warming of water due to global warming may or may not trigger other ENSO-like changes.
Coastal Upwelling System . California's coastal upwelling system is well known, including its variability with respect to intensity or degree of vertical advection with time. Upwelling events occur as pulses, which are presently on the order of three to six days. The pulsing pattern is biologically important to nearshore species such as fish larvae. Upwelling itself is associated with deleterious effects of offshore transport and turbulent mixing which dilutes food concentrations.
Onshore Airflow . The cool upwelled surface waters in turn cool and stabilize onshore airflow, which creates a cool summer climate along the coast. The longshore wind which drives coastal upwelling is partly maintained by an atmospheric pressure gradient between the "thermal" low pressure cell that develops over California's heated interior and the higher pressure maintained over the cooler ocean.
Wind Stress . An increase in the equator-ward wind stress over the California Current has been observed since 1946. A similar increase has also been noted for other major upwelling systems. With a warming trend, wind intensity and upwelling intensity would increase. Consequently, one cannot simply expect sea-surface isotherms to increase because of effects of other physical forces such as wind stress. Furthermore, upwelling intensity and degree of turbulence are apparently linked to the recruitment of key food organisms such as pelagic fish (e.g., northern anchovy).
Horizontal Advection . In addition to coastal upwelling, California's marine ecosystems are strongly influenced by horizontal advection (lateral flow) from the north. Both processes bring nutrients to the system, although upwelling is probably more important in contributing nutrients very near the shore and horizontal advection more important in the main body of the California Current. Both sources may be affected by global warming. A decrease in the California Current's strength appears to be characteristic during ENSO episodes (fig. 2), hence global warming might create a similar decrease. ENSO events can serve as one model of the consequences of warming in this current system (Chelton 1981; Chelton, Bernal, and McGowan 1982; Simpson 1984; McGowan 1985; Hickey 1979).
Anoxia . A gradual change in the characteristics of marine ecosystems can have profound effects on productivity in benthic and demersal communities or communities in poorly mixed environments. As water temperature increases owing to global warming, oxygen solubility will decline. This decline in oxygen solubility will facilitate the development of anoxic conditions, particularly below the photic zone, where decomposition of organic detritus can entirely consume the dissolved oxygen. Under these conditions, toxic substances such as ammonia and sulfides are generated.
Possible Impacts of Climate Change
Global warming may result in El Niño–like conditions. CalCOFI has monitored the California Current before, during, and after both the 1958–59 and the 1983–84 ENSOs, and these studies therefore serve as an excellent model for predicting effects of global warming on marine ecosystems (fig. 2). The forty-year CalCOFI biological-physical data set gathered during these ENSOs has to date been only partially analyzed, but from it we can suggest some plausible scenarios (Chelton, Bernal, and McGowan 1982):
|
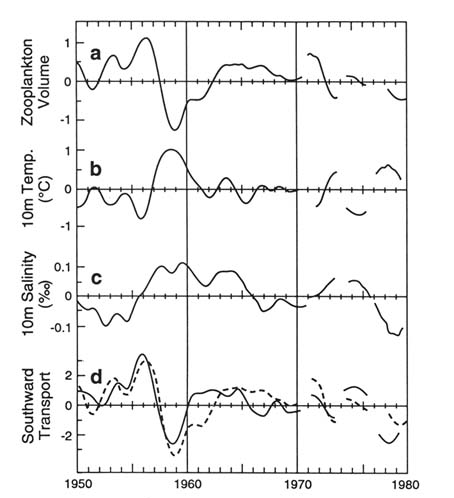
Figure 2. The low-frequency, nonseasonal anomalies from the long-term
means of ecosystem properties in the California Current. The data are
space-averaged over a very large area. The axes are in standardized units vs. time.
The dash line in panel (d) is the same as panel (a) zooplankton. This figure
shows the very close relationship between the large variations in biomass
and the variations in transport of cold, low salinity water from the north.
The period 1958–59 was a strong El Niño period in this current
system. (From Chelton et al. 1982)
There is a possibility of increased coastal upwelling due to the increased land-sea contrast in air temperature. The biological effect of this upwelling is partially dependent on the depth of the thermocline and nutricline. A slow California Current will probably be associated with a deep thermocline, and relatively little nutrient enhancement would be provided by upwelling under these conditions. An intensified California Current may be associated with a shallow thermocline, in
which case coastal upwelling would be colder and nutrient-rich. The scenario of warmer terrestrial conditions in California does not necessarily equate to warmer marine conditions, especially during the spring and summer upwelling period. Furthermore, we can expect ENSO events to continue to be superimposed on the new system, with even warmer maximum ocean temperatures. The time of onset of the upwelling season begins earlier in the south than in the north. If the Pacific high moves northward, we can expect the onset of upwelling to occur earlier in the year.
Response of Fish . The response of various fish species to global climate change may be to change their productivity and migration patterns, but varying considerably among species.
Effects of Anoxia . In southern California, broad areas of undisturbed sediments suggest that areas of extreme anoxia, with oxygen concentrations of less than 0.1 mL/L, are expanding rapidly. If areas of anoxia expand because of increased temperature and declining oxygen solubility, certain economic impacts are to be expected. These include declining fisheries and general oceanic productivity, and declining recreational use of the coastal zone. In addition, an increase in hydrogen sulfide releases in coastal zones is likely.
Coastal-zone fisheries and other marine life will decline either by direct toxic effects of anoxia or by upwelling of toxic waters into the photic zone. The vast majority of the biomass of the ocean lives in the surface wind-mixed layer of the ocean, trophically dependent on photosynthesis. The development of anoxia, or rapid vertical advection of anoxic waters into the surface mixed layer, could create an ecologic crisis for various marine groups through a combination of 1) direct toxicity; 2) reduction or modification of nutrient or food supply; 3) chronic debilitation caused by contact with such deep waters; or 4) increased predation by more adaptive or less affected taxa. Developing anoxia affects a biotic community not only by lack of oxygen but also by changes in temperature, pH, and other constituents present in the water. The presence of chelating agents, antagonistic or synergistic elements, redox state, pH, and temperature modify the actual chemical configuration or activity experienced by the organism.
Along the California coast, the ocean is used as a dump for biological wastes, particularly from the Hyperion sewage plant in Los Angeles. The ability of the ocean to absorb biological wastes may rapidly diminish as the basins off southern California become increasingly anoxic. In extreme cases, hydrogen sulfide generation (such as that reported in a fjord in Oslo, Norway) causes blackening of houses and boats.
Effects on San Francisco Bay . Global warming would affect San Francisco Bay principally by causing sea-level rise. Higher sea level would flood present marshes and other tidal wetlands as well as push the
entrapment zone upstream. Present levees would be breached, producing many shoal-water areas. Runoff from storm drains and from fields would lead to enhanced productivity in the expanded bay. At the same time, warming of surface waters would reduce oxygen content. Influx of waters from streams would decline as the upland soils became drier. Reduced water flow would tend to concentrate nutrients and organisms in the expanded bay. Reduced oxygen content and expanded shallow waters, rich in organisms, could lead to hypoxic conditions such as those experienced at present in similar environments in the Baltic and Adriatic. Global warming could lead to large areas of oxygen-deficient waters in areas now occupied by delta water. Indeed, global warming could result in reversion to conditions of the 1940s and early 1950s when bay tidelands were characterized by obnoxious odors and lack of marine life.
Wetland Ecosystems
"Wetlands are lands transitional between terrestrial and aquatic systems where the water table is usually at or near the surface or the land is covered by shallow water" (U.S. Fish and Wildlife Service [Cowardin et al. 1979]). This classification requires the presence of at least one of three attributes: 1) hydrophytes; 2) hydric soil; or 3) nonsoil saturated by water or covered by shallow water at least periodically. Wetlands of the United States are classified (Cowardin et al. 1979) in five major systems (marine, estuarine, riverine, lacustrine, palustrine), all of which occur in California.
Marine Wetlands
Marine wetlands include intertidal wetlands and subtidal deepwater habitats of the open ocean, where they overlie the continental shelf and extend landward to the limit of tidal inundation (Cowardin et al. 1979). Along the coast of California, marine wetlands consist of wave-cut bedrock outcrops, sand and pebble beaches, and cobble deltas that generally are dominated by a rich association of algae, marine angiosperms, and invertebrates. Impacts to marine wetlands as a result of global warming will come largely from an anticipated rise in sea level. Accentuating the effect of sea-level rise is the simultaneous subsidence of some areas.
Potential impacts from sea-level rise will include:
|
|
Artificial barriers may exist or may be built to protect coastal real estate and could further limit the landward migration of marine wetlands. A rise in sea level of one-third meter could erode beaches from 67 to 133 meters in California (Titus 1989), but because many are not this wide, substantial loss of marine intertidal zones, wetland habitat, and recreational sites is likely in urban areas with seawalls. If the rise in sea level is one-half meter, Titus estimates one-third of coastal wetlands in the United States would be inundated.
Estuarine Systems
Estuarine systems include deepwater subtidal habitats and intertidal wetlands that generally are semienclosed by land but open at least sporadically to the ocean and receive at least occasional dilution from freshwater runoff (Cowardin et al. 1979). The upstream limit extends to where ocean-derived salts are diluted to 0.5 percent during the period of average annual low flow (Cowardin et al. 1979). In general, estuarine wetlands occur between the highest tide of the year and mean sea level. Prominent estuarine habitats are fairly well documented in terms of floral composition and distribution. Estuaries serve as habitats for many rare and protected species.
At least 75 percent of the estuarine wetlands of southern California are estimated to have been destroyed in the last century (Zedler 1982), and most of the remaining ones are either degraded, fragmented, or isolated remnants of historically larger wetland systems. Landward migration of wetlands as a result of sea-level rise will be constrained by abrupt topography of the coastline and artificial barriers constructed to protect agricultural lands and urban areas.
Possible Impacts of Climate Change
The EPA has estimated that from 40 to 73 percent of the wetlands in the United States could be lost by the year 2100 but that the potential formation of new wetlands might reduce this loss to from 22 to 56 percent (Armentano, Park, and Cloonan 1988). Other estimates include a 30 to 70 percent loss with a one-meter rise and 33 to 80 percent loss with a two-meter rise, 90 percent of which would be in the southeastern United States (Titus 1989). In California, 35 to 100 percent of the EPA study wetlands were projected to be lost during the same period. This loss could be reduced to 1 to 18 percent if developed or protected areas
were abandoned to allow landward migration of wetlands (Armentano, Park, and Cloonan 1988; Titus 1989).
General possible impacts of global warming on estuaries include:
|
In California, estuaries can be grouped into several major types: 1) structural basins (e.g., Carpinteria Salt Marsh and Goleta Slough) that are often hypersaline, have steep watersheds, and receive much sediment; 2) mouths of canyons that support small lagoons (e.g., Devereaux Slough) with great seasonal fluctuations in salinity and water regimes; 3) mouths of rivers (e.g., the Sacramento/San Joaquin Delta) with brackish lagoons and contiguous riparian corridors; and 4) large bays with extensive salt and brackish marshes (e.g., San Francisco Bay) and contiguous tidal riverine and palustrine habitats. Each of these estuaries may be affected differently by a rise in sea level, depending on natural and artificial barriers that would obstruct the landward migration of wetlands and saline water.
Riverine Systems
The riverine systems include wetlands and deepwater habitats contained within channels, except those supporting woody or persistent emergent vegetation (= palustrine) or those with water containing ocean-derived salts in excess of 0.5 percent (= estuarine) (Cowardin et al. 1979). The distribution of some species (particularly fish) is sensitive to water salinity. Areas such as the Sacramento River are important for spawning of anadromous fish species.
Possible Impacts of Climate Change
The global warming scenario for California could have profound effects, which may, however, be subtle or difficult to interpret. Potential effects include: 1) increased winter runoff and decreased spring and summer runoff from mountains; 2) increase in salinity and inundation from sea-level rise; 3) increase in evaporation as a result of increased temperatures; and 4) movement of saline water landward in riverine systems.
Runoff . Warmer temperatures will melt snow earlier and cause more of the precipitation to occur as rain, which could cause a reduction in snowpack, an increase in winter runoff, and a reduction in spring and summer flows (Gleick 1988; Smith and Tirpak 1989; Smith 1989). Because the current reservoir system in the Central Valley does not have the capacity to store more winter runoff and also provide adequate flood control, the additional winter flow would have to be released, causing increased winter erosion and decreased spring and summer inundation of riverine wetlands.
Increased Water Demand . A temperature increase of 4° C with no increase in rainfall is estimated to produce an average reduction of 10 percent in annual runoff in northern California due to higher evaporation rates (Gleick 1988; California Energy Commission 1989) and as much as a 62 percent decrease in summer runoff. An increase in temperature also will increase demand for water resources for agriculture and urban needs (California Energy Commission 1989), which will further stress riverine systems, particularly if the timing and quantity of runoff change. All of these stresses could affect the species composition, productivity, fishery, and general environmental quality of riverine wetlands and adjacent deepwater habitats in California.
Increased Salinity . Stress in wetland vegetation as a result of increased salinity from a rise in sea level may alter species distributions and successional patterns of plant communities (Pezeshki, DeLaune, and Patrick 1987). Reduced spring and summer flows and a potential decrease in precipitation would increase the potential for movement of salt water into the riverine wetlands.
Competition between human uses of water resources and requirements to maintain natural ecosystems could result in serious conflicts. To preserve the freshwater nature of riverine and adjacent palustrine wetlands, water may have to be released from reservoirs to augment reduced spring and summer flows of rivers that empty into estuaries, thereby forcing salt and brackish water back into bays (Titus 1989). However, potentially increased human demands on water resources, partly from the anticipated higher temperatures, may make this alternative impossible. Construction of new reservoirs to provide additional holding capacity to capture potential increased winter runoff would cause the destruction of other riverine habitats where the reservoirs would be built, and could further reduce sediment input to areas such as the Sacramento/San Joaquin Delta, limiting the potential for estuarine wetlands to keep pace with a rise in sea level.
Landward Movement of Saline Waters . A rise in sea level by one meter will cause significant movement of salt water up rivers and change tidal riverine wetlands and deepwater habitats into estuarine wetlands and deepwater habitats. This process would be harmful to native plants and
animals generally restricted to the freshwater habitats and could seriously threaten human uses of fresh water derived from these sources (Titus 1989). Increased salinity could also prevent spawning of anadromous fish species.
Lacustrine Systems
Lacustrine systems include wetlands and deepwater habitats situated in basins or dammed river channels. They lack woody or persistent emergent vegetation, generally exceed eight hectares, often have wave-formed or bedrock shorelines, in their deepest portions may exceed two meters at low water, and never exceed 0.5 percent salinity from ocean-derived salts (Cowardin et al. 1979). A lacustrine system may be bounded by the estuarine, riverine, or palustrine systems and may include a limnetic subsystem (deepwater habitats) and a littoral subsystem (wetland habitats), which extend from the shore to the maximum depth of growth of nonpersistent emergent plants (Cowardin et al. 1979). Wetlands associated with desert lakes and their lacustrine wetlands are important habitats for many endangered species and for migratory birds.
Possible Impacts of Climate Change
The effects of global warming on lacustrine systems could include 1) increase in temperatures; 2) change in water input and outflow amounts and patterns; and 3) changes in air quality that affect water chemistry.
Temperature . Increased temperatures and decreased rainfall could reduce lake levels and change shoreline vegetation. Where lake levels are low, pollution is diluted less, and water quality could decline, affecting entire lacustrine systems. Higher temperatures in lakes could increase the growth of aquatic plants and algae, change circulation patterns, and reduce levels of dissolved oxygen (Smith 1989; California Energy Commission 1989).
Runoff and Evaporation . Changes in the amount of runoff and evaporation (as described for riverine wetlands) could also affect lacustrine wetlands. Desert lakes of California (e.g., Mono and Owens lakes), already stressed by excessive water diversion and high rates of evaporation, could be further stressed by increased rates of evaporation and decreases in precipitation. Wetlands on the margins of these lakes might desiccate earlier each year and could be reduced in size, composition, and zonation. However, potential increases in winter runoff from nearby mountains could provide beneficial inflows for systems such as Mono Lake.
Palustrine Systems
The palustrine system includes: 1) all wetlands dominated by trees, shrubs, persistent emergent plants, emergent mosses and lichens; 2) all such wetlands as occur in tidal areas where salinity due to ocean-derived salts is less than 0.5 percent; and 3) all nonvegetated wetlands less than eight hectares without wave-formed shorelines and where salinity due to ocean-derived salts is less than 0.5 percent (Cowardin et al. 1979).
In California, palustrine wetlands include, for example, small bodies of open water (e.g., dune swale ponds), nonpersistent emergent wetlands (e.g., vernal pools), persistent emergent wetlands (e.g., freshwater and alkali marshes and seeps), scrub/shrub wetlands (e.g., riparian and alkali scrub), and forested wetlands (e.g., riparian woodlands and forests). These wetlands support many endemic and rare and endangered plants and animals, are important for migratory birds, often have enormous food chain support values, and are highly regarded for their scientific, educational, recreational, and aesthetic values.
Possible Impacts of Climate Change
Palustrine wetlands could be affected by all aspects of global warming, including increases in temperature, changes in precipitation, a rise in seal level, and changes in air quality. Both negative and beneficial impacts might occur. For example, in discussing potential benefits of global warming, the California Energy Commission (1989) has suggested that an increase in rainfall in mountainous and foothill areas could lead to increased soil-moisture levels and an increase in the rangeland yield of meadows, which are generally forms of palustrine emergent wetland. They emphasize, however, that droughtlike conditions may become more frequent in some areas and "desertification" might affect others. Such changes could result in the loss of palustrine wetlands, an increase in alkaline soil conditions, a displacement of wetland communities, and regional extirpation of sensitive species. Specific impacts are best described in reference to specific examples of palustrine wetlands.
Desert Marshes and Alkali Flats—Fish Slough . Fish Slough in Owens Valley supports extensive flooded marshes, perennial springs, sensitive alkali flats, endemic and/or endangered species of fish, plants, and a snail, and is valued highly for its aesthetics and biological and cultural resources (Bureau of Land Management 1985; Forbes, Ferren, and Haller 1988). Changes in precipitation, snowmelt, flow from springs, and evapotranspiration rates due to global warming could seriously alter the sensitive balance of this ecosystem.
Central Valley Freshwater Marshes . Extensive natural and artificial marshes occur in the Central Valley (e.g., Gray Lodge Wildlife Management Area in the Sacramento Valley) and are major habitat areas for
migratory birds. Henderson (1989) suggests that increased temperatures and seasonal changes in rainfall of inland areas could reduce such wetlands in North America and seriously affect the reproduction of breeding waterfowl. Gleick (1988) suggests that changes in wildlife refuges of the Central Valley could affect the last remaining stopovers for migratory waterfowl in the Pacific Flyway. Increased runoff from the Sierra Nevada, however, could be managed to offset regional changes in climate and could result in the preservation or increase in such wetlands, unless conflicts develop over the use of water resources for human needs. Such a conflict is likely because 85 percent of the state's water supplies already are used for irrigation (Smith 1989).
Vernal Pools . Vernal pools, among California's most rare and sensitive wetland habitats, are seasonal wetlands in depressions that support many endemic and endangered organisms with winter and spring rains, followed by summer desiccation. Because of their occurrence on relatively flat terrain, vernal pools in general have received frequent, widespread, and often devastating perturbations due to the spread of agriculture and urban development (Cheatham 1976; Zedler 1987). Increased temperatures and reduced rainfall might result in a loss of shallow pools, decrease in size of deeper pools, loss of species that are growing at the limits of their ranges, and the endangerment of rare, narrowly restricted species.
Coastal Palustrine Marshes . Various types of palustrine marshes adjacent to or landward of estuaries and/or impounded by levees would be threatened throughout California by a rise in sea level because of increases in inundation and salinity. Particularly vulnerable are those marshes in the Delta and South Bay regions of San Francisco Bay (Williams 1985; Armentano, Park, and Cloonan 1988; California Energy Commission 1989; Josselyn and Callaway 1988). Emergent wetlands dominated by tules and cattails, seasonal alkali wetlands on deltaic deposits, salt ponds, and nontidal brackish marshes in historic estuarine areas could be permanently inundated with a one-meter rise in sea level by the year 2030, especially if the levee system in the Delta region is not maintained.
Riparian Corridors . California is rich in examples of stream and riverbank vegetation dominated by various trees and shrubs correlated with latitude, elevation, hydrology, or proximity to the coast. Depending on the severity of temperature and precipitation changes or the intensity of air pollution, the species composition of some riparian scrubland, woodlands, or forests could change significantly. Major climatic zones could shift as much as 30–60 km northward (Knox 1989). Because the shift in climate zones might take place in decades rather than thousands of years, many riparian tree species in North America might not be able to migrate northward in latitude or upward in elevation at a rate necessary
for germination, establishment, and growth (Roberts 1989; Smith and Tirpak 1989), resulting in some treeless riparian areas.
Interior Ecosystems
Although much information is available concerning the terrestrial vegetation and climate of California (Barbour and Major 1988), the only comprehensive relationship of vegetation and climate in relation to climate change is presented by Westman and Malanson (1990), from whom our figure 3 is taken. This figure maps the distribution of vegetation types in two-dimensional space defined by mean temperature in the warmest month and annual precipitation. For example, a 4°C warming on a site
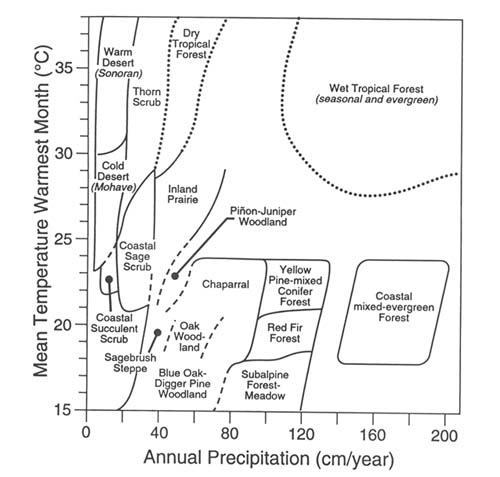
Figure 3. Classification of California vegetation types according to temperature and
precipitation. (From Westman and Malanson 1990)
currently anywhere within subalpine forest/meadow space results in mean temperatures that occur nowhere within subalpine forest/meadow currently. This condition suggests that subalpine forest/meadows will be eventually replaced by red fir forest.
In the following sections, six different dominant California interior vegetation types or ecosystems are considered: forests, lakes and streams, shrublands, inland prairies, deserts, and alpine ecosystems of the Sierra Nevada.
Forests
What We Know about Forests
Physiology . Physiological processes in trees are greatly influenced by environmental variables. These relationships are well known in general. For example, rates of photosynthesis are related to light levels, moisture stress, temperature, availability of nutrients and CO2 , and other plant stresses (such as air pollution) (Kramer and Kozlowski 1979; Smith 1981). Laboratory studies have provided evidence that drought stress is offset in some species, at least temporarily, by elevated levels of CO2 (e.g., Kramer and Sionit 1987). However, there is little information available regarding the response of forest-grown trees to the combined effects of elevated temperatures, changed moisture regimes, and enhanced CO2 .
Populations . Population-level studies of forest trees emphasize reproduction and life cycles (e.g., Harper 1977). Trees have different tolerance levels for temperature, light, moisture stress, and nutrients at different stages in their life cycles. For example, seedlings may be girdled and killed by soil-surface temperatures that do not influence mature trees adversely. These tolerance levels may influence tree growth and viability at many stages of the life cycle. Climate change could affect the reproductive cycle, seedling survival, and mortality at many ages from combined stresses and reduction in productivity, chilling requirements for bud break, and hardening off at the end of the growing season (Fowells 1965).
Communities . The relationships between communities and climate are well documented, including those for forest cover types in the United States. Palynological investigations document the relationship between long-term climate change and vegetation change (Faegri and Iversen 1975). The study of past climate and vegetation change in California has lagged behind similar research in other parts of North America. Only a handful of Quaternary scientists have worked in California, and they have been hampered by the large floristic and topographic diversity of California (Adam 1985). Adam has inventoried the sites with fossil pollen records from California. Over one hundred continuous records exist
for California, but the striking conclusion from Adam's survey is that many of the best data are not published.
Ecosystems . Natural, undisturbed ecosystems, their processes and dynamic equilibria, vary with vegetation, soil, and climate. Ecosystem processes (e.g., decomposition) occur at different rates in different ecosystems as a result of the varying influences of vegetation (and other organisms), soil, and climate. Stressors that change the rate of ecosystem processes will result in changes in the community and changes in the equilibria of the ecosystem. Forest ecosystem processes are generally well understood owing to the intensive studies conducted at several experimental forests in the U.S. Notably, no similar long-term research sites now exist in California. Furthermore, there have been no experiments conducted anywhere which address forest productivity (as distinguished from seedling productivity) or forest ecosystem carbon balance (as distinguished from seedling or very small tree carbon balance). Modeling efforts (e.g., Botkin and Nisbet 1990) currently addressing this issue are hampered by a lack of information about the interrelationships between climate change, CO2 fertilization, resource limitation, stresses, and tree physiology.
Possible Impacts of Climate Change
Specific impacts of global warming on California forests include:
|
Lakes And Streams
What We Know about Lakes and Streams
Aquatic ecosystems integrate coupled atmospheric and terrestrial watershed effects in their physical, chemical, and biological characteristics. Aquatic ecosystems, particularly in mountain environments, are quite
sensitive to environmental disruptions. At high elevations (alpine and subalpine) snowmelt quantity and chemistry have immediate effects on stream and lake dynamics (Melack et al. 1989). Many lakes are flushed annually during spring snowmelt. They are naturally very oligotrophic and of low alkalinity. As a consequence, they respond quickly to snowmelt acidity (Melack et al. 1989) and nutrient dilution or enrichment from the watershed or directly from the atmosphere (Goldman, Jassby, and Powell 1989). Interannual variation in the heat budgets (Strub, Powell, and Goldman 1985) and primary productivity (Goldman, Jassby, and Powell 1989) of subalpine lakes are strongly linked to climatic variation. Mountain streams are often almost completely dependent on the surrounding forest for their source of reduced carbon, as aquatic productivity is often light- and nutrient-limited (Meyer, Likens, and Sloane 1981). Any disturbance of a watershed can have both immediate and long-term effects on the nutrient and sediment load of the stream (Vitousek and Melillo 1979).
Possible Impacts of Climate Change
Examples of likely impacts include:
|
Shrublands
What We Know about Shrublands
The only information available on the direct effect of elevated carbon dioxide on chaparral species derives from measurements of photosynthetic response to elevated carbon dioxide and temperature in leaf chambers placed on field plants (Wright 1974). This study indicates that some chaparral species are able to use additional CO2 to stimulate photosynthetic rates. The long-term effects of elevated CO2 on growth of the species alone, or in combination with climatic changes, are not
known. There have been no long-term chamber or field-exposure studies done with elevated CO2 on any of the California shrubland species.
Possible Impacts of Climate Change
Specific impacts on shrublands may include:
|
Inland Prairies
What We Know about Inland Prairies
Before its agricultural development, the Central Valley of California was occupied by a mixture of grassland, dry shrubland, and riparian woodland. Increased fire frequency induced by Native Americans, combined with domesticated grazing introduced by settlers in the nineteenth century, encouraged a relative abundance of grasses, especially annual grasses, in this vegetative mix. The dry shrubland was probably characterized by such semiarid species as Artemisia tridentata and Haplopappus linearifolius . Riparian woodland would have been dominated by such species as cottonwood, Populus trichocarpa .
Possible Impacts of Climate Change
Specific likely impacts on shrubland ecosystems include:
|
Deserts
What We Know about Deserts
One-third of California is desert, including all of the area inland of the Transverse and Peninsular ranges in southern California, the valleys
east of the Sierra and Cascade ranges, and part of the southern San Joaquin Valley. Except for the San Joaquin Valley, the deserts of California are extensions of larger desert regions that cover much of the southwestern United States and northwestern Mexico. These deserts have different climates and support different plant and animal communities. The Sonoran Desert extends into southeastern California at elevations below 500 m. The California component of the Sonoran Desert is extremely arid, with a total annual rainfall of only 50 to 150 mm. Consequently, most of the region is characterized by a sparse vegetation of creosote bush, bursage, and occasionally abundant ephemerals (Burk 1977). Only in washes and on rocky slopes of desert mountains are found large cacti and arborescent species characteristic of the wetter parts of the Sonoran Desert in Arizona and Sonora, Mexico. To the north of the Sonoran Desert elevation increases, and above about 500 m (approximately along the Riverside—San Bernardino county line) there is a gradation into the Mojave Desert. The principal climatic features of the Mojave Desert are an extreme deficit of summer rainfall, more winter rainfall than the Sonoran Desert, and nightly frost in midwinter (January mean monthly minimum temperature below 0° C). Creosote bush and bursage remain the dominant plant species in the Mojave (Vasek and Barbour 1977), but the associated arborescent species differ from those of the Sonoran Desert. Many of the Sonoran Desert species are frost-sensitive and apparently cannot survive in the Mojave Desert (Turner and Brown 1982).
To the north of the Mojave Desert, lying in the vast rain shadow of the Sierra and Cascade mountain ranges, is the extreme western edge of the Great Basin Desert. Its high elevation with mean minimum temperatures below 0° C during the winter make this "cold" desert botanically distinct from the "hot" deserts (MacMahon 1979). Big sagebrush and shadscale are principal dominant shrubs with bunchgrasses in more moist habitats (Young et al. 1977). Moisture comes mostly during the coolest half of the year; summers are hot and dry. Thus, periods of suitable moisture for plant growth are substantially out of phase with periods of suitable temperature, greatly limiting plant growth.
Survival in the desert depends on an organism's ability (adaptation) to utilize specific "pulses" or "windows" of resource availability (Noy-Meir 1973). Winter annual plants, for example, are adapted to germinating, growing, and completing their life cycle within the winter season, while summer annual species are adapted to the short summer season. Desert granivores are adapted to utilizing seeds of a select group of species. To the extent that climate change brings about a restriction of availability of a resource, say summer moisture or seeds, then species adapted to utilizing that resource will incur direct impacts, with potential decline in vigor and abundance, or even elimination.
Possible Impacts of Climate Change
Specific likely impacts in California deserts include:
|
Alpine Ecosystems Of The Sierra Nevada
What We Know about Sierra Alpine Ecosystems
Most of California's alpine ecosystems are found in the Sierra Nevada above about 2,800 meters. These systems may be generally defined as small lake and stream watersheds having dilute surface waters, acidic soils, exposed bedrock, and sparse vegetation. The hydrology of these watersheds is dominated by snowfall, which can account for more than 90 percent of the wet deposition falling above the forest line. Most of our understanding of ecological processes in these watersheds and surface waters is based on studies to investigate the effects of acid deposition and other anthropogenic disturbance (e.g., Cooper, Jenkins, and Soiseth 1988).
Possible Impacts of Climate Change
The following changes in alpine ecosystems may be expected:
|
|
Recommendations For Research
It is obvious that global climate change of the magnitude suggested in foregoing scenarios will result in very serious impacts on California natural ecosystems. It is also obvious that we lack sufficient information both to estimate the impacts with certainty and to know how to respond effectively. California needs an aggressive ecosystem research program to 1) compile existing sources of information; 2) determine accurate models for predicting regional changes; 3) begin monitoring of current conditions to provide a baseline for future comparisons; and 4) conduct experiments under field conditions and in the laboratory to better understand biological feedback mechanisms.
The best approach to understanding potential problems and setting policy regarding the impacts of global warming on the California environment is to develop an integrated statewide program of ecological study. We suggest that the University of California in conjunction with state agencies develop a California Ecosystems Program to foster collaboration on natural systems research. The state at present has only a few small long-term research sites in terrestrial ecosystems (e.g., Deep Canyon, administered by UC Riverside), and it has no large laboratory research facility for manipulation of plant communities at the level required. A California Ecosystems Program could serve as a clearinghouse and storage bank for data, coordinate specific research projects and activities on key habitats, and provide integrative communication mechanisms such as sponsorship of symposia and regular publications.
The mission of a California Ecosystems Program should include these general areas of activity:
|
|
|
References
Adam, D. P. 1985. Quaternary pollen records from California. In Pollen records of late-Quaternary North American sediments , ed. V. M. Bryant, Jr., and R. G. Holloway, 125–140. Dallas: American Association of Stratigraphic Palynologists Foundation.
Armentano, T. V., R. A. Park, and C. L. Cloonan. 1988. Impacts on coastal wetlands throughout the United States. In Greenhouse effect, sea level rise, and coastal wetlands , ed. J. G. Titus. EPA 230-05-86-013. Washington, D.C.: U.S. Environmental Protection Agency.
Barbour, M. G., and J. Major, eds. 1988. Terrestrial vegetation of California . New expanded ed. California Native Plant Society, Special Publication no. 9. Davis, Calif.: California Native Plant Society.
Botkin, D. B., and R. A. Nisbet. 1990. Projecting the effects of climate change on biological diversity in forests. In Consequences of the greenhouse effect for biodiversity , ed. R. Peters. New Haven: Yale University Press.
Bureau of Land Management. 1985. Management plan for Fish Slough: Area of critical environmental concern, a cooperative management program . Department of the Interior, Bishop Resource Area.
Burk, J. 1977. Sonoran Desert. In Terrestrial vegetation of California , ed. M. G. Barbour and J. Major, 869–889. New York: Wiley.
Byron, E. R., A. Jassby, and C. R. Goldman. 1988. Water quality of subalpine lakes. In The potential effects of global climate change on the United States , ed. J. B. Smith and D. A. Tirpak. EPA-230-05-050. Washington, D.C.: U.S. Environmental Protection Agency.
California Energy Commission. 1989. The impacts of global warming on California: Interim report . P500-89-044. Sacramento: California Energy Commission.
Cheatham, N. D. 1976. Conservation of vernal pools. In Vernal pools: Their ecology and conservation , ed. S. Jain. Institute of Ecology Publication no. 9. Davis, Calif.: Institute of Ecology, University of California, Davis.
Chelton, D. B. 1981. Interannual variability of the California Current: Physical factors. CalCOFI Report 22:34–48.
Chelton, D. B., P. A. Bernal, and J. A. McGowan. 1982. Large-scale physical and biological interaction in the California Current. J. Mar. Res. 40:1095–1125.
Cooper, S. D., T. M. Jenkins, and C. Soiseth, 1988. Integrated watershed study: An investigation of fish and amphibian populations in the vicinity of the Emerald Lake Basin, Sequoia National Park: Final report . Sacramento: California Air Resources Board.
Cowardin, L. M., V. Carter, F. C. Golet, and E. T. LaRoe. 1979. Classification of
wetlands and deepwater habitats of the United States . FWS/OBS-79/31. Washington, D.C.: Office of Biological Services, U.S. Fish and Wildlife Service.
Davis, M. B. 1989. Lags in vegetation response to global climate change. Climate Change 15:75–82.
Faegri, K., and J. Iversen. 1975. Textbook of pollen analysis . New York: Hafner Press.
Forbes, H. C., W. R. Ferren, Jr., and J. R. Haller. 1988. The vegetation and flora of Fish Slough and vicinity, Inyo and Mono counties, California. In Plant biology of eastern California , ed. C. A. Hall and V. Doyle-Jones. Natural History of the White-Inyo Range Symposium, vol. 2. Los Angeles: University of California White Mountain Research Station.
Fowells, H. A. 1965. Silvics of forest trees of the United States . Agriculture Handbook no. 271. Washington, D.C.: U.S. Department of Agriculture, Forest Service.
Gleick, P. H. 1988. Impacts on natural resources: Climate change and its impact on water resources. Testimony prepared for Subcommittee on Water and Power Resources, Fort Mason Conference Center, October 17, 1988.
Goldman, C. R., A. Jassby, and T. Powell. 1989. Interannual fluctuations in primary production: Meteorological forcing to two subalpine lakes. Limnol. Oceanogr . 34:310–323.
Harper, J. L. 1977. Population biology of plants . New York: Academic Press.
Henderson, S. 1989. How it could be: Species. EPA Journal 15, no. 1:21–22.
Hickey, B. M. 1979. The California Current system—hypotheses and facts. Prog. Oceanog . 8:191–279.
Josselyn, M., and J. Callaway. 1988. Ecological effects of global climate change: Wetland resources of San Francisco Bay . Prepared for the Environmental Protection Agency, Environmental Research Laboratory, Corvallis, Oregon.
Knox, J. B. 1989. Climate scenarios selected for the first UC/DOE Workshop. Backgrounder Contribution no. 18.
Kramer, P. J., and T. T. Kozlowski. 1979. Physiology of woody plants . New York: Academic Press.
Kramer, P. J., and N. Sionit. 1987. Effects of increasing carbon dioxide concentration on the physiology and growth of forest trees. In The greenhouse effect, climate change, and U.S. forests , ed. W. E. Shands and J. S. Hoffman, 219–246. Washington, D.C.: The Conservation Foundation.
Leonard, R. L., L. A. Kaplan, J. F. Elder, R. N. Coats, and C. R. Goldman. 1979. Nutrient transport in surface runoff from a subalpine watershed, Lake Tahoe basin. California Ecol. Monogr . 49:281–310.
Leverenz, J. W., and D. J. Lev. 1987. Effects of carbon dioxide-induced climate changes on the natural ranges of six major commercial tree species in the western United States. In The greenhouse effect, climate change, and U.S. forests , ed. W. E. Shands and J. S. Hoffman, 123–188. Washington, D.C.: The Conservation Foundation.
Lewis, W. M., and M. C. Grant. 1980. Relationships between snow cover and winter losses of dissolved substances from a mountain watershed. Arctic and Alpine Research 12:11–17.
MacMahon, J. A. 1979. North American deserts: Their floral and faunal components. In Aridland ecosystems: Structure, functioning and management , ed. D. W. Goodall and R. A. Perry, 1:21–82. London: Cambridge University Press.
McGowan, J. A. 1985. El Niño in 1983 in the southern California Bight. In El Niño north , ed. W. W. Wooster and D. L. Fluharty. Seattle: Washington Sea Grant Program, University of Washington.
Melack, J. M., S. D. Cooper, T. Jenkins, L. Barmuta, S. Hamilton, K. Kratz, J. Sickman, and C. Soiseth. 1989. Chemical and biological characteristics of Emerald Lake and streams in its watershed, and the responses of the lake and streams to acidic deposition: Final report . Prepared for the California Air Resources Board. Santa Barbara, Calif.: Marine Science Institute and Dept. of Biological Sciences, University of California.
Meyer, J. L., G. E. Likens, and J. Sloane. 1981. Phosphorus, nitrogen, and organic carbon flux in headwater streams. Arch. Hydrobiol . 91:28–44.
Noy-Meir, I. 1973. Desert ecosystems: Environment and producers. Ann. Rev. Ecol. Sys. 4:25–51.
Pezeshki, S. R., R. D. DeLaune, and W. H. Patrick, Jr. 1987. Effect of salt water intrusion on physiological process in wetland plant communities. In Wetland and riparian ecosystems of the American West , ed. K. M. Mutz and L. C. Lee. Denver: Planning Information Corp.
Roberts, L. 1989. Research news: How fast can trees migrate? Science 243:735–737.
Roos, M. 1989. Possible climate change and its impact on water supply in California. In Oceans '89: An international conference …, September 18–21, 1989, Seattle, Washington, USA . Piscataway, N.J.: IEEE Service Center.
SFBCDC (San Francisco Bay Conservation and Development Commission). 1987. Future sea level rise: Predictions and implications for San Francisco Bay . Prepared by Moffatt and Nichol, Engineers; Wetland Research Associates, Inc. San Francisco: San Francisco Bay Conservation and Development Commission.
Schulze, E. D., and F. S. Chapin, III. 1987. Plant specialization to environments of different resource availability. In Potentials and limitations of ecosystem analysis , ed. E. D. Schulze and H. Zwolfer, 120–148. Ecological Studies, 61. New York: Springer-Verlag.
Simpson, J. J. 1984. El Niño-induced onshore transport in the California Current during 1982–83. Geophys. Res. Let . 11:232–236.
Smith, J. B., and D. A. Tirpak, eds. 1989. Potential impacts of climate warming on California. In The potential effects of global climate change on the United States , vol. 1, Regional studies , chap. 4. EPA-230-05-89-050. Washington, D.C.: U.S. Environmental Protection Agency.
Smith, J. E. 1989. How it might be: Water resources. EPA Journal 15, no. 1:19–20.
Smith, W. H. 1981. Air pollution and forests: Interactions between air contaminants and forest ecosystems . New York: Springer-Verlag.
Strub, P. T., T. Powell, and C. R. Goldman. 1985. Climatic forcing: Effects of El Niño on a small, temperate lake. Science 227:55–57.
Titus, J. G. 1989. How it could be: Sea levels. EPA Journal 15, no. 1:14–16.
Turner, R. M., and D. E. Brown. 1982. Sonoran desert scrub. Desert Plants 4:181–221.
Vasek, F. C., and M. G. Barbour. 1977. Mojave desert scrub vegetation. In Terrestrial
vegetation of California, ed. M. G. Barbour and J. Major, 835–867. New York: Wiley.
Vitousek, P. M., and J. M. Melillo. 1979. Nitrate losses from disturbed forests: Patterns and mechanism. Forest Sci. 25:605–619.
Westman, W. E., and G. P. Malanson. 1990. Effects of climate change of Mediterranean-type ecosystems in California and Baja California. In Consequences of the greenhouse effect on biodiversity , ed. R. Peters. New Haven: Yale University Press.
Williams, P. B. 1985. An overview of the impact of accelerated sea level rise on San Francisco Bay . Philip Williams and Associates Project no. 256. San Francisco: Philip Williams and Associates.
Wright, R. D. 1974. Rising atmospheric CO2 and photosynthesis of San Bernardino mountain plants. Amer. Midl. Nat. 91:360–370.
Young, J. A., R. A. Evans, and J. Major. 1977. Sagebrush steppe. In Terrestrial vegetation of California , ed. M. G. Barbour and J. Major, 763–796. New York: Wiley.
Zedler, J. B. 1982. The ecology of southern California coastal salt marshes: A community profile . FWS/OBS-81/54. Washington, D.C.: U.S. Fish and Wildlife Service, Biological Services Program.
Zedler, P. H. 1987. The ecology of southern California vernal pools: A community profile . Biological Report 85. Washington, D.C.: U.S. Fish and Wildlife Service.