Physiology of Sleep-Associated Apnea
There are four primary areas to consider when discussing the physiology of sleep apnea in seals: respiratory, cardiac, circulatory, and metabolic alterations. The electrophysiology of sleep in pinnipeds is also of great interest but will only be referred to here when sleep staging is important to better understand the four areas noted above. In each of these areas, data from diving apnea will be compared and contrasted. The goal of this section is to ask how sleep and dive apnea may be related, keeping in mind that while at sea, seals may sleep while at depth and thus combine the two events. Most of this information comes from studies on elephant seal pups, with some comparative work on Weddell seals. This is all very recent work and is still in the process of being analyzed. Thus, a great deal of this discussion is based on personal observations, but references to work already published will be noted as available.
Respiratory Pattern
In elephant seal pups, the typical respiratory pattern while sleeping is to link together several periods of apnea and eupnea into one "bout" of sleep. Thus, the seal may sleep for 30 to 40 minutes and during that time go through a 10-minute period of apnea, 2 to 3 minutes of eupnea, another apnea, another short eupnea, and then a final apnea before waking. Figure
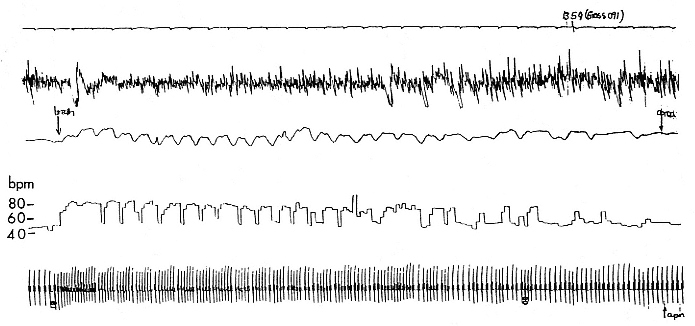
Fig. 19.1
Polygraph recording of a sleeping, 3-month-old northern elephant seal pup. The top line is a time trace with 5-second tick marks.
Line 2 is the electroencephalogram (EEG) showing large-voltage, slow-frequency recordings typical of slow wave sleep (SWS).
Line 3 is a respiratory trace showing the end of a 12-minute apnea, about 2.6 minutes of eupnea, and then the beginning of
another apnea. Line 4 is the instantaneous heart rate showing the low heart rate during apnea, the postapnea tachycardia,
the appearance of the normal sinus arrhythmia, and then the low heart rate of the next apnea. The heart rate calibration is on
the left. Line 5 is the electrocardiogram (EKG), which varies depending on the respiratory cycle.
19.1 shows a short (2.5-min.) breathing interval between two longer apnea periods. There are many variations to this pattern, but it is critical to note that apnea is not a prerequisite for sleep (i.e., a seal can be ventilating and sleeping) and that sleep is not a prerequisite for apnea (an awake seal can breath hold). However, the longest apneas appear only during sleep, and awake apnea seems to have a limit of about 5 minutes. The longest period of apnea that has been reported on a 4-month-old elephant seal pup is just over 11 minutes (Castellini, Costa, and Huntley 1986), but recent recordings have exceeded 14 minutes. Average apnea duration ranges from about 6 to 8 minutes for a 3½- to 4-month-old pup. It is interesting to note that the pattern of long apnea relative to short eupnea is similar to the repetitive diving habits of both elephant seals (Le Boeuf et al. 1986) and Weddell seals (Castellini, Davis, and Kooyman 1988). This pattern implies that oxygen loading and carbon dioxide dumping are accomplished quickly during the respiratory period and that there is no metabolic processing of hypoxic end products. In fact, as will be discussed in detail later, there is no apparent change in plasma lactate or glucose during or after sleep apnea in elephant seals. Neither we nor Hubbard (1968) were able to find a clear correlation between the length of the eupnea and the length of the preceding apnea, although Huntley (1984) saw such a relationship in his study, which was conducted using different methods on restrained pups. Similarly, there is no relationship between the surface interval duration and preceding dive duration interval in elephant seals (Le Boeuf et al. 1988). In Weddell seals, there is no relationship between dive time and surface time during aerobic diving, but there is a longer surface recovery time correlated to increasing postdive lactate loads after anaerobic diving (Kooyman et al. 1980). Thus, both sleep apnea and diving apnea have the similar appearance of short eupnea periods between longer apnea periods, occur in bouts, and appear to be mostly aerobic.
Cardiac Pattern
The bradycardia associated with natural diving seals is well documented and has been shown to be related to the length of the dive (Kooyman and Campbell 1973) and to ascent and descent patterns (Hill et al. 1987) and therefore, presumably, to both effort and the need to stay underwater as long as possible. Similarly, Bartholomew (1954) noted that there was bradycardia in sleeping elephant seals with a reduction in heart rate from about 65 beats per minute (BPM) to 54 BPM in adults. G. L. Kooyman (1968) recorded the heartbeats of sleeping Weddell seals and also observed a decline in heart rate of about 30 to 40% in adults. S. H. Ridgway, R. J. Harrison, and P. L. Joyce (1975) demonstrated a slowing of heart rate in sleeping gray seals, and Huntley (1984) recorded the electrocardiogram (EKG) of restrained, sleeping elephant seal pups and showed that the aver-
age sleeping heart rate declined during apnea to about 70% of average, awake, breathing values. This pattern of bradycardia during the sleep apnea in seal pups appeared straightforward until we recently found two patterns that suggest the heart rate changes may be more complicated. First, the "bradycardia" in sleeping northern elephant seal pups seems to be age related in that 2-month-old pups neither drop their heart rate as low as 4-month-old pups nor seem to hold the heart rate steady during apnea. This same pattern has been seen in Weddell seal pups (Kooyman 1968). Second, there may not actually be a true bradycardia in the sense of the dramatic instantaneous decline seen in diving animals. By analyzing the EKG with an instantaneous beat-to-beat heart rate analyzer, we found that during eupnea, the older pups show a normal sinus arrhythmia. That is, as they inhale, heart rate increases, and as they exhale, heart rate declines. During eupnea, heart rate varies from about 80 BPM high to about 50 BPM low, for an "average" heart rate of about 65 BPM. As the pups enter into apnea with the last exhalation of their breathing period, the heart rate declines just as it had during the exhalation in eupnea and then stays at the 50 BPM rate during the long apnea. This pattern is clearly shown in figure 19.1. Thus, it seems that the "bradycardia" associated with sleep apnea is actually the low heart rate of a normal respiratory cycle and that the apnea is just a very long breath pause, at least in terms of cardiac control. The only time that the heart rate becomes very low during sleep is when the pup moves from slow wave sleep (SWS), which is the predominant type of sleep state, into rapid eye movement (REM) sleep. At this point the heart rate can get very low (around 20–25 BPM) and become quite variable.
The heart rate of freely diving elephant seals is being studied at this time (R. Andrews, pers. comm.). Preliminary evidence suggests that the most common heart rate of freely diving elephant seals may, in fact, be closer to the rate seen during sleep apnea than to low rates more often associated with forced diving conditions. However, very low heart rates have been observed and reinforces the point that the heart rate in these animals is not a reflex and is probably under higher-level control. Therefore, what at one time seemed to be clear sleep and dive apnea bradycardia may not remain as clear as more information is collected.
Circulatory Alterations
In 1980, Kooyman et al. found that the hemoglobin (Hb) levels in the blood of freely diving seals varied before and after dives. When the seals returned from a dive, the Hb levels would be high but declining. Later, J. Qvist et al. (1986) showed that Hb and hematocrit (Hct) in Weddell seals began to increase as soon as the dive began, leveled out at high values during the dive, and then declined afterward. M. A. Castellini, R. W. Davis, and G. L. Kooyman (1988) showed that the Hct in Weddell seals tended to
stay high during an entire diving bout and only returned to resting levels during very long breaks in diving. This variation in Hct has been proposed to be caused by the sequestering and release of red blood cells (RBC) by the spleen during diving events (Zapol 1987). The maximization of Hct in the interdive surface interval would certainly facilitate the rapid loading of oxygen and also maximize the amount of oxygen that could be carried by the blood. Increased Hct is also known to occur in racing horses and dogs during sprint events (Harris et al. 1986). This is a perfect example of the difficulty of trying to separate diving into its exercise and apnea components. Does the change in Hct in diving seals come about because of exercise, or is it related to breath holding? As it turns out, sleeping seals may provide the answer.
In 1986, M. A. Castellini, D. P. Costa, and A. C. Huntley found that the Hct in sleeping elephant seal pups began to increase as soon as the apnea started and then declined as soon as breathing began. It has recently been found that when several apnea-eupnea-apnea cycles are linked together, the Hct stays elevated during the entire cycle and then drops to resting values when the pup is awake and breathing for a long period. Therefore, it seems reasonable to conclude that the change in Hct that occurs in diving seals most likely arises from the apnea response and not from exercise. If the spleen is the modulating organ for this phenomenon, then it must begin to contract on the initial apnea and sequester RBC during eupnea. However, if the next apnea follows soon after the short eupnea, there would not be enough time for all the cells to be gathered, and thus the Hct will stay somewhat elevated during the breathing period.
The striking similarity between the diving and sleeping apnea alterations in Hct suggests that the neurological mechanisms involved in initiating and maintaining the apnea are the same for both diving and sleeping. This is a critical point because sleep apnea in seals appears to be centrally controlled. That is, it is a neurologically influenced event and not obstructive apnea. In obstructive apnea, which is very common in humans, the upper airway becomes blocked during sleep as the tissues around the trachea relax and the patient begins, essentially, to suffocate. In obstructive apnea, the patient tries to breathe but cannot and must awaken to break the pattern (Strohl, Cherniak, and Gothe 1986). In seals, there is no attempt to breathe during the sleep apnea event (fig. 19.1). The apnea is controlled from higher central nervous system centers, and the same neural inputs that initiate the breath hold must also contract the spleen. The advantage of a high Hct, in both sleeping and diving seals, is that they can load oxygen quickly during the short eupnea period following the apnea.
There are additional changes that occur in the circulation of sleeping seals that strike parallels with the diving condition. It is well known that there are marked circulatory perfusion shifts that occur in diving seals. The
classic dive response involves shunting blood flow away from the peripheral tissues and conserving the oxygen-rich blood for the more aerobic central organs, such as the brain and heart. This shunting has been visualized in a variety of methods, but one that is relevant here is a procedure that examines how plasma radioisotope tracers can show such shifts. When a radioactive metabolic tracer is injected into the circulation of a diving seal, the tracer slowly equilibrates into the blood pool and is only slowly utilized until the dive ends. At that point, the tagged tracer is metabolized at the normal resting rate. At the point of inflection, the specific activity of the tracer in the plasma falls dramatically and provides a qualitative method to visualize the transition. This process appears to occur in both laboratory dives (Castellini et al. 1985) and natural dives (Guppy et al. 1986). Similarly, such transition points have been observed at the apnea/eupnea transition at the end of sleep apnea in northern elephant seal pups (Castellini 1986). When a tracer is injected into a sleeping seal, it follows a distinct pattern during the apnea and is then altered as soon as breathing occurs. These data imply that the same type of circulatory shifts that have been so well documented in diving seals may also occur in the sleeping seal.
On the basis of these two different indications of circulatory modifications that occur in sleeping seals, it is tempting to suggest that many of the same control mechanisms that regulate circulation during diving also occur in the sleep apnea event.
Metabolic Changes
While diving, there are a variety of blood chemistry changes that can occur. The first and most obvious is that blood oxygen decreases as the dive progresses, and the animal becomes hypoxic. Carbon dioxide partial pressure increases, and there is a respiratory acidosis induced by the high CO2 (Kooyman et al. 1980; Qvist et al. 1986). During long dives, beyond the aerobic diving limit (ADL), lactate accumulates in the periphery and is flushed into the circulation when the peripheral tissues are reperfused after the dive ends (Guppy et al. 1986; Kooyman et al. 1980). Despite the low oxygen levels that are reached in all dives, the majority of dives, at least in Weddell seals, are known to be aerobic and do not show the characteristic increase in lactate after the dive. Similarly, the concentration of plasma glucose, the ultimate substrate for the lactate, drops during anaerobic diving but does not change during aerobic diving (Castellini, Davis, and Kooyman 1988; Guppy et al. 1986; Kooyman et al. 1980). Finally, during bouts of dives, there appears to be very little change in glucose or lactate over hours of diving unless a long dive occurs (Castellini, Davis, and Kooyman 1988).
During sleep apnea, blood oxygen declines to very low levels, CO2 increases, and there is a respiratory acidosis (Kooyman et al. 1980). However, plasma lactate and glucose remain stable and do not change before,
during, or after any single apnea or bout of apnea (Castellini, Costa, and Huntley 1986; Castellini and Castellini 1989). Thus, sleep apnea would appear to be mostly aerobic. This makes sense, since the seal sleeping on the beach can simply breathe when it becomes necessary. However, this does raise an interesting problem for seals that may be sleeping underwater at sea. If a sleeping seal has dropped its blood oxygen and raised its carbon dioxide to the point where is it necessary to breathe, it cannot ventilate if it is at 500 m depth. It would seem to be necessary for the seal to either awaken and swim to the surface or to somehow stay asleep and get to the surface. We know that sleeping elephant seal pups can come to the surface from about 0.5 m in a tank and ventilate without having to awaken. But floating to the top of a 0.5 m tank is considerably different from swimming to the surface from 500 m. We are left with trying to construct a control mechanism that signals to the seal when it will be necessary to breathe and get the animal to the surface while it is sleeping. Perhaps, however, elephant seals do not sleep while at sea, although this is unlikely given that they are pelagic for months at a time.
There is one last area of metabolic alteration that is of importance, and this concerns the metabolic cost of diving or sleeping. In Weddell seals, it has been shown that diving is not very costly and only elevates metabolism by 1.5 to 2 times over resting (Kooyman et al. 1973). We have recently found that for dive events and sleep apnea events of the same duration, diving only costs about 1.5 times the cost of sleep in Weddell seals (Castellini, Kooyman, and Ponganis 1992). If this is an energy demand that is typical of phocids, then we might be able to predict the metabolic cost of diving in elephant seals from the oxygen requirements of sleeping. For elephant seal pups, this would not be a difficult calculation, because there is a considerable amount of data available on the oxygen consumption patterns of pups during both apnea and eupnea periods. For adult elephant seals, however, this would involve finding a way to measure the oxygen consumption rate of a large and intractable animal. However, measuring the oxygen consumption rate of a sleeping elephant seal on land is infinitely easier than obtaining the same information on one that is diving at sea.