In the Ether
In October 1940 Alfred Loomis, who had been appointed head of microwave work under Karl Compton's division of NDRC, called members of his committee to meetings at his home and laboratory in Tuxedo Park, New York. Lawrence was among them. They agreed that the technology made possible, and the emergency recommended, the establishment of a central national laboratory to do "anything and everything that was needed to make microwaves work."[78] To confuse the enemy, and in honorific obfuscation, the center, located at MIT, took the name "Radiation Laboratory." It invented or perfected many sorts of radars operating at wavelengths around and below 10 cm. In devising these most important aids to detection and navigation of ships and planes, blind landings, gun laying, and so on, the MIT Radiation Laboratory drew twice over on the experience of cyclotroneers. For one, it acquired appropriately socialized staff with relevant technical knowledge. ("If all the energy which has gone into nuclear physics since 1932 were turned onto this problem [the air menace]," Cockcroft had predicted, "it would be solved.") To head the new Rad Lab, the Microwave Committee chose Lee DuBridge of Rochester, whom Lawrence rated as the most desirable laboratory leader of his generation, an excellent physicist, administrator, and team player, the perfect cyclotroneer. As DuBridge later recalled, with an exaggeration that illustrates the strength of the brotherhood he represented so well, "our whole initial group at the MIT laboratory were the cyclotroneers—all had been associated with Ernest [Lawrence] either remotely or intimately." A meeting on applied nuclear physics in Cambridge around November 1, 1940, arranged by Livingston and attended by 600 physicists from all across the country, provided a perfect recruiting ground.[79]
[78] Loomis to Lawrence, 1 Oct, and reply, 3 Oct 1940 (46/8); MIT, Five years , 12, quote.
[79] Cockcroft to Lawrence, 24 Oct 1938 (4/5); Lawrence to Zeleny, 5 Mar 1940 (18/44); Guerlac, Radar , 260–1; DuBridge, interview by James Culp, Oct 1981, 11, quote; Condon, BAS, 1:11 (15 May 1946), 8–9.
Table 10.1 lists the ten cyclotroneers engaged during the first three months of the MIT Rad Lab's operations. One came and stayed as director; six entered as or quickly became group leaders; five left as division leaders (there were seven R&D divisions in all); two ended on the laboratory's steering committee. Lawrence's devotion to Loomis and conviction of the importance of radar caused him to send his very best men, Alvarez and McMillan, and his expert on radio frequency systems, Salisbury. McMillan, who left MIT soonest, worked on field tests of an interception system against aircraft and on airborne radar for detecting and homing on ships. Salisbury directed efforts in his specialty. Alvarez, who stayed the longest of the Berkeley group, moved furthest. After helping to develop radar for attack planes, he invented a system to guide approaching aircraft from the ground and directed work on other aids to detection, navigation, and identification.[80] Another man from Berkeley, Lauriston Marshall of the Department of Electrical Engineering, had a career at the MIT laboratory similar to a cyclotroneer's: he came to work on magnetrons, rose immediately to group leader, then chairman of the Ship Committee and director of the British Branch of the Radiation Laboratory, and closed the war as head of the Laboratory's Operational Research Section attached to the Headquarters of the U.S. Air Force in the Pacific.
Marshall represents the second way in which accelerator laboratories contributed essentially to radar. In the late 1930s, he helped transform Sloan's latest piece of cyclotronics into a generator of radio waves at a frequency and power suitable to radar. This was a tube designed to remove the obstacle encountered in the early 1930s to the development of the Wideröe linac into a useful tool in nuclear physics. To recall the old difficulty, a Wideröe machine for protons of reasonable size and ambitious energy would require a power oscillator working at a wavelength of two or three meters, about an order of magnitude shorter than commercial tubes could easily handle. As he remembered his
[80] Lawrence to Dunning, 9 Nov 1940 (6/19); MIT, Rad. Lab., "Staff;" Alvarez, Adventures , 86–101. We omit mention of radar work at Bell Labs, not because it lacked importance, but because it lacked cyclotroneers; Fisk, Hagstrum, and Hartman, Bell System techn. jl., 25:2 (1946), 1–189.
|
tinkering to improve their performance, Sloan "just pushed triodes and tetrodes to high power, high frequency, beyond anything the cyclotron needed." By May 1940, he and Marshall had a tube that oscillated at 50 cm and with great power—some 2,500 watts. Cooksey reported this news to Loomis, who had not waited for the NDRC to begin to push work on microwave electronics. Loomis was elated.[81] The cause of elation: the size of radar sets diminished, while the detail of the objects they could see increased, with decline of wavelength down to about 1 cm, where atmospheric absorption begins to make trouble. The Sloan-Marshall tube, or "resnatron," held promise as the fast-paced heart of a powerful, centimetric microwave transmitter for airborne use.[82]
As soon as he became head of the NDRC's microwave work, Loomis asked Lawrence to take responsibility for the further development of the resnatron "in a big way," as "the major war research of the University [of California]." The Research Corporation, which had patented the resnatron, gave $4,500; Loomis provided $1,500 from his deep pocket and a promise of $20,000 of NDRC funds. Lawrence agreed to sail "full speed ahead" with the help, if necessary, of moneys diverted from the 184-inch cyclotron.[83] The good ship resnatron was then not the only centimetric pulser at sea in the Bay Area. Loomis had asked Lawrence to send Sloan and Marshall to San Carlos, California, to join their work with efforts under way there to perfect something called the klystron.[84] Like the resnatron, the klystron resulted from efforts at a university—in its case Stanford—to overcome the frequency limit of commercial oscillator tubes. Stanford wanted a very powerful x-ray tube, no less than 3 MV, but did not want to pay for it. Considering strategies in 1934, William Hansen, an instructor in Stanford's physics department, thought to set up oscillations in a
[81] Sloan, interview by A. Norberg, 10 Dec 1974, 52–3, quote (TBL); Cooksey to Loomis, 14 May 1940, and other correspondence of Loomis, Cooksey, and Lawrence, May 1940 (46/8).
[82] Terman, Elect. radio eng. , 810, 854, 1018–9.
[83] Loomis to Lawrence, 9 Jul and 12 Aug 1940, and Lawrence to Loomis, 26 Jul and 6 and 29 Aug, 28 Sep 1940 (46/8).
[84] Loomis to Lawrence, 26 June, and reply, 1 Jul 1940 (46/8); Poillon to P.R. Bassett, Sperry, 26 June, and to Lawrence, 2 Aug 1940, and Lawrence to Poillon, 6 and 24 Aug 1940 (15/18).
cavity, a transmission line, as it were, with no inner electrode. The thing itself is simple enough in principle and, moreover, of convenient size: a cube 10 cm on a side resonates at a wavelength of 14 cm. It may be driven by a transmission line that creates an appropriate magnetic field within a loop coupled to the cavity. A struck bell is a crude, but serviceable, analogy. In 1937 Hansen had a visionary plan to drive electrons to 100 MeV within his "rhumbatron," as he called his cavity resonator. He sent off a report, in the usual way, to a professional journal; but Stanford's administrators, sensing something big, made him hold it back for a year while they made sure of the commercial possibilities.[85]
The rhumbatron transformed into the klystron when Hansen's former roommate, Russell Varian, discovered a novel way to control electrons. That was in the summer of 1937. Varian and his brother Sigurd, a former commercial pilot, then worked with Hansen as unpaid research associates on the design of a microwave device for navigating and detecting airplanes. This purpose had seized Sigurd Varian, who knew the dangers of commercial flying and could imagine those of enemy action. The klystron (fig. 10.1) consists of two reentrant cavities C1 and C2 , separated by a drift space RS. A beam of electrons from the tubular cathode at the top accelerates under the dc voltage between M and the grid P, whence they drift through the field-free region PQ and into the neck of the "buncher" cavity C1 . Between Q and R they suffer an oscillating field created through the loop F. This experience causes them to collect into bunches in the drift space RS: an electron that crosses just before the oscillating field rises to zero will be slowed; one that crosses when the field goes positive will speed up. As they drift, the retarded early electron, the on-time electron, and the hurrying late electron will congregate. The neck of the "catcher" cavity C2 stands where the density is greatest and the induced field has the proper phase to oppose the motion of the bunches (and so derive energy from them). The congregations pass at the frequency of the buncher, thereby exciting very strong
[85] Science news , in Science, 84 (16 Oct 1936), suppl., 9, reported that Hansen had electrons of 5 MeV; Nahmias to Joliot, 28 Apr 1937 (JP, F25), mentioned the plan for 100 MeV; Hansen, Jl. appl. phys., 9 (1938), 654–63, rec'd 17 Jul 1937; Ginzton, IEEE, Spectrum (Feb 1975), 33.
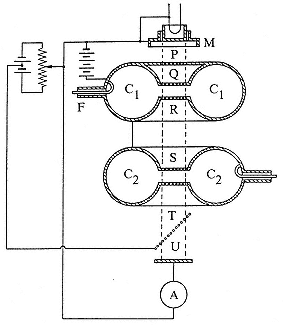
Fig. 10.1
The klystron as first described by the Varian
brothers. R. Varian and S. Varian, J1 appl. phys. ,
10 (1939), 324.
oscillations in the tuned catcher. The deceleration of the bunches in the catcher prevents them from overcoming the opposing voltage between T and U, a fact recorded by the meter at A. The whole business rests in a vacuum. By August 1937 a cardboard version coated with copper foil was working at l = 13 cm. The Sperry Corporation undertook to develop it for aircraft detection.[86] This was the line of research to which Loomis wished to couple development of the Sloan-Marshall tube. As it happened, the Varians and Hansen went to Sperry's research laboratory in New York, where the klystron developed into a versatile circuit element. Hansen spent much of his time in the East lecturing about microwaves at the MIT Radiation Library.
[86] Ginzton, IEEE, Spectrum (Feb 1975), 34–9; Varian and Varian, Jl. appl. phys., 10 (1939), 321–7; Webster, ibid., 501–8, 864–72. The business can be followed in detail in the Hansen Papers, Stanford Univ. Library.
Neither Berkeley's nor Stanford's entry into what had become a worldwide race for a transmitter of centimetric radar could generate the necessary power in 1940. The device on which the MIT Rad Lab was raised later in the year had much in common with them, however; it came from a university laboratory much concerned with particle accelerators and exploited the bunching principle introduced by the Varian brothers. This "cavity magnetron" came from Oliphant's institute at the University of Birmingham. Its inventors, J.T. Randall and H.A.H. Boot, arranged an anode consisting of a thick ring scalloped by cavities around a central cylindrical cathode (fig. 10.2). Electrons move toward the anode under a dc potential and a magnetic field strong enough to bend them all back to the cathode. When the cavities resonate under an external impulse, their radio frequency fields, leaking into the anode space, slow some electrons enough that the magnetic force on them (which is proportional to their velocity) no longer suffices to return them to the cathode. Electrons so affected bunch together and add energy to the resonant cavities as they pass en route to the anode. In effect, the cavity magnetron is to the klystron what the cyclotron is to the linac.[87]
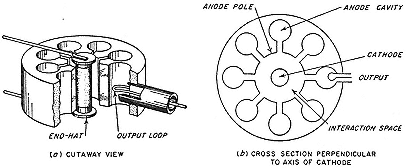
Fig. 10.2
The cavity magnetron. Modulation of the radial electrostatic field between
the cylindrical cathode and the concentric anode-block by a high-frequency
field leaking from the cavities causes some of the electrons to bunch.
Terman, Elec. rad. eng. , 689.
[87] Terman, Elect. radio eng. , 689—95; Hagstrum, IRE, Proc., 35 (June 1947), 548–64.
The cavity magnetron was the centerpiece of many ingenious devices that a British technical mission under Henry Tizard, which included Cockcroft and Fowler, showed American military and scientific men in September 1940 in the hope of returns in kind. The magnetron made an impression. The Naval Research Laboratory then had a klystron transmitter operating at 10 watts. The British cavity magnetron gave a thousand times as much power at the same wavelengths. Cockcroft and E.J. Bowen explained its operation in detail at a gathering at Loomis's estate at the end of September. Two weeks later, on October 12–13, the Microwave Committee met with Bowen and Cockcroft, again at Tuxedo Park. They decided to copy Britain in entrusting development of radar to interdisciplinary teams of academic scientists and engineers. Lawrence ran to Loomis's telephone. "During the next few weeks [as Bowen recalled] he was to telephone every physicist of consequence in the United States." A month or so later the MIT Rad Lab, "a central laboratory built on the British lines, was in operation."[88]
The magnetron did not end the war for the resnatron. After Marshall threw in his lot with the MIT group and Sloan at last won his Ph.D. in Berkeley, the new doctor took his tube for treatment at Westinghouse's Research Laboratory in Pittsburgh. There it waxed exceedingly robust. Westinghouse built forty-two of the final design, each of which weighed 500 pounds. These fat resnatrons knocked out everything on the air. They were of first importance in jamming German radar on D-Day.[89]