The Importance of Riparian Vegetation to Stream Ecosystems[1]
Allen W. Knight and Richard L. Bottorff[2]
Abstract.—Riparian vegetation is very important in determining the structure and function of stream ecosystems. Most aquatic organisms, both invertebrates and fish, are directly or indirectly dependent on inputs of terrestrial detritus to the stream for their food. Natural changes in riparian vegetation and the biotic processing of detritus, as well as other factors, determine the kinds and abundance of aquatic invertebrates living in streams, from headwaters to large rivers. Removal of riparian vegetation will significantly affect stream organisms by: 1) decreasing detrital (food) inputs; 2) increasing the potential for primary production in aquatic plants; 3) increasing summer water temperatures; 4) changing water quality and quantity; and 5) decreasing terrestrial habitat for adult insects.
Introduction
The manner in which riparian systems are managed and protected is commonly related to their value as buffer strips, stream bank stabilizers, and fish and wildlife habitat. These strips of streamside vegetation may be the only habitat remaining for some wildlife species. As riparian vegetation is modified or destroyed by grazing, logging, urbanization, road construction, water development, mining, and recreation, interest in its importance is increasing. Our objective is to briefly review the role of riparian vegetation in the structure and function of stream ecosystems, especially headwater streams. We also explore the possible effects of vegetation modification or destruction in headwater streams. Whenever possible, our review emphasizes conditions found in Sierra Nevada streams.
Headwater Streams
Headwater streams are greatly influenced by riparian vegetation since they function as processors of natural organic matter coming from the watershed (Cummins and Spengler 1978). These small streams are characteristically shaded and kept cool by overhanging riparian vegetation, which also contributes dead organic matter (detritus) to the stream. Shading not only affects water quality but influences the activities of primary producers such as algae and aquatic macrophytes. Riparian vegetation supplies organic matter in the form of dead leaves, needles, twigs, branches, logs, bud scales, fruit, droppings of terrestrial animals (frass), and dissolved organic matter (DOM).
The direct input of organic matter from riparian vegetation is substantial: annual values range from about 100 gm. per m2 to more than 1,000 gm. per m2 (Bray and Gorham 1964; Anderson and Sedell 1979), and values for standing crops can be much higher (Naiman and Sedell 1979). The addition of this organic matter is fundamentally important to the stream biota since this is often its major energy source, which is supplemented by lesser amounts of autochthonous production (Hynes 1963; Cummins 1974). Dead organic matter may contribute as much as 99% of the annual energy input to headwater streams covered by a dense forest canopy (Fisher and Likens 1973). Particulate detritus accounted for 53% of the annual energy input to Bear Brook, New Hampshire, and DOM input accounted for 47%; autochthonous primary production by mosses contributed very little. These streams are termed "heterotrophic", because in effect they consume organic matter produced by adjacent terrestrial systems.
Although allochthonous detrital input to streams continues throughout the year, seasonal pulses do occur. Detritus is added in autumn from deciduous leaf-fall and plant die-off. In winter and spring it is washed in by higher runoff (Minshall 1968; Fisher and Likens 1973;
[1] Paper presented at the California Riparian Systems Conference. [University of California, Davis, September 17–19, 1981].
[2] Allen W. Knight is Professor of Hydrobiology, University of California, Davis. Richard L. Bottorff is Research Assistant, Department of Land, Air, and Water Resources, University of California, Davis.
Hobbie and Likens 1973). Additional pulses may include bud scales in spring and frass in summer. Tree branches broken by wind and snow may drop into streams in winter. Rainstorms periodically wash in DOM exuded from plants or collected on leaves from arboreal animals, while groundwater continuously brings in DOM.
Although the heterotrophic nature of headwater streams enclosed in forests has been well emphasized by recent research (Fisher and Likens 1973; Cummins 1974), headwater streams in unforested or sparsely-forested regions can be autotrophic, receiving most of their energy from primary production of aquatic macrophytes and algae (Minshall 1978). Autotrophy has been documented in desert streams lacking riparian vegetation and shading (Naiman 1976; Minshall 1978; Busch and Fisher 1981) and has been suggested for high-altitude streams (Cummins and Klug 1979), especially in western montane regions (Wiggins and Mackay 1978). Headwater streams within forests can also change seasonally from heterotrophy to autotrophy, depending upon natural variations in light intensity, nutrients, hydrologic factors, and detrital input (Naiman and Sedell 1980).
Data are currently lacking to classify Sierra Nevada headwater streams as either heterotrophic or autotrophic. The vegetation, climate, and geology of Sierra Nevada mountains vary substantially from location to location. Thus headwater streams may vary widely in their heterotrophy/autotrophy balance. The extensive forests and chaparral on the western slope of the Sierras do suggest that detritus from riparian vegetation is very important to stream energetics. Even above the timberline, dense growths of willow, alder, grasses, and herbs overhang the small stream channels, supplying detritus and shading the water. Only at high elevations when streams flow over granite bedrock is riparian vegetation sparse and the stream unshaded.
Organic Matter Processing
The importance of organic matter contributions from riparian vegetation to stream ecosystems has been fully appreciated for only about 10 years (Cummins 1974). The manner in which aquatic organisms utilize and process organic matter at different seasons and locations along streams is a current research topic (Cummins 1973, 1975; Cummins and Klug 1979; Anderson and Sedell 1979; Hawkins and Sedell 1981). We briefly summarize here the role of aquatic organisms in continually processing and transforming organic matter from the time it enters the stream (fig. 1).
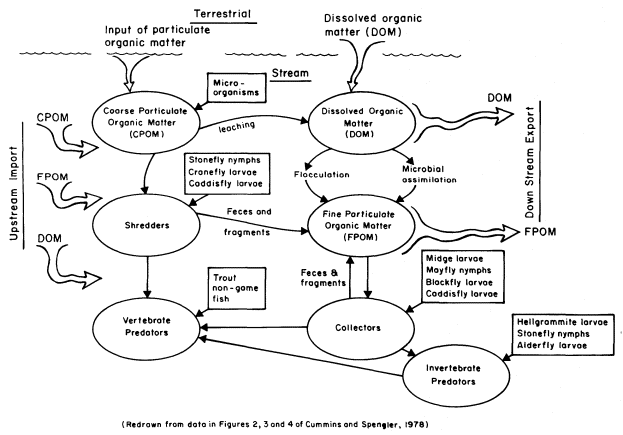
Figure l.
Schematic diagram depicting the processing of dead organic matter in
headwater streams (redrawn from data in Cummins and Spengler 1978).
Coarse particulate organic matter (CPOM: > 1 mm. diameter), such as leaves, starts leaching DOM once it enters the water. Up to 30% of dry weight may be leached in the first day; deciduous leaves leach faster than coniferous needles (Cummins 1974; Hynes etal . 1974). Fungi and bacteria rapidly colonize the leaves undergoing leaching. Although most of these microbes can metabolize cellulose, only some can use lignin (Cummins and Spengler 1978). Certain aquatic insects such as some stonefly nymphs, midge larvae, cranefly larvae, and caddisfly larvae shred or break down leaves (CPOM) during feeding and are called "shredders" (Cummins 1973). The microorganisms that colonize the leaves are an important source of shredder nutrition.
Shredder and microorganism feeding eventually breaks down CPOM into fine particulate organic matter (FPOM: < 1 mm. diameter). However this process is only one source of FPOM. FPOM may result from: 1) shredder and microorganism feeding on CPOM; 2) physical abrasion of CPOM by stream turbulence; 3) fine particles eroded from streambed algae; 4) fine material washed or blown in from the surrounding watershed; and 5) conversion from DOM by chemical and microbial activity (Cummins 1974). Dissolved organic matter leached from CPOM, plus DOM entering from the watershed, aquatic plants, and microbial excretions, can be partially converted into FPOM. This conversion is accomplished by physical flocculation and microbial assimilation, processes dependent on water turbulence, temperature, pH, and various ionic concentrations (Lush and Hynes 1973).
FPOM is the food for aquatic organisms known as "collectors". These animals obtain FPOM either by gathering it from stream substrate deposits or by filtering it from the flowing water. Deposit feeders include certain midge larvae and mayfly nymphs. Filter feeders have diverse ways of capturing FPOM from the passing water (Wallace and Merritt 1980). Blackfly larvae possess fan-shaped structures on their heads for filtering FPOM and transferring it to their mouths. Some caddisfly larvae construct detailed silk nets capable of sieving out FPOM. The net is often held between small twigs or stones exposed to the current, and the larva hides in a tube just behind. The collected FPOM contains bacteria on its surfaces, which increases the quality of the food for the collector. Particle size is very important to collectors since their mouthparts and sieving devices have specific shapes and openings for obtaining and handling FPOM.
A thin film of algae covers most stream substrates and contributes to instream primary production, especially when light intensity and nutrient concentrations are high. Microscopic diatoms are often the most abundant algal group, but larger filamentous green and blue-green algae are also common. Aquatic organisms known as "scrapers" have well-adapted mouthparts for scraping up and consuming this algal film, which also includes some FPOM and microscopic animals. Scrapers in Sierra streams include many mayfly nymphs, water penny beetles, riffle beetles, and some midge larvae.
Some aquatic invertebrates and vertebrates prey on shredders, collectors, scrapers, and each other; they are known as "predators". Predators in Sierra streams include many stonefly nymphs, dragonfly nymphs, some midge larvae, alderfly larvae, and dobsonfly larvae. Most aquatic insects in streams, even those that are predatory, are potential prey for trout and many nongame fish species.
The amount, kind, and timing of riparian vegetation additions to the stream and the shading provided by streamside plants will determine which feeding groups (shredders, collectors, scrapers, predators) prosper at any site. Thus, the population abundance of stream animals and community composition of the stream ecosystem are dependent on riparian vegetation.
The River Continuum Concept
The structure and function of aquatic communities along a river system have recently been organized into the River Continuum Concept (Cummins 1975; Vannote etal . 1980). This concept involves several stream factors—temperature, substrate, water velocity, stream morphology, and energy inputs from allochthonous and autochthonous sources—which interact to influence the availability of food for stream animals. These factors should vary in a predictable fashion from headwaters to downstream locations, and should produce predictable distributions of the four feeding groups along the continuum (fig. 2).
Since headwater streams (orders 1-3) are often heavily shaded and receive large amounts of organic matter from riparian vegetation, these streams are heterotrophic. Their ratio of gross photosynthesis (P) to respiration (R) will be less than one. Coarse substrates predominate, since stream gradients and erosive power are high. Shredders reach maximum abundance in these upper stream sections because of the abundant CPOM. FPOM and DOM are used and exported downstream. Because many Sierra headwater streams originate within coniferous forests, they may differ from typical headwater streams originating within deciduous forests of the eastern United States in detrital input and lighting conditions.
Organic matter input and shading are less important in medium-sized rivers (orders 4-6) because of the greater widths and more open canopy. Increased primary production shifts these streams from heterotrophy into autotrophy, and a P:R ratio greater than one. Increased algal production allows scrapers to be abundant. Collectors are also common, and a few shredders are still present. FPOM and DOM are again used and exported downstream.
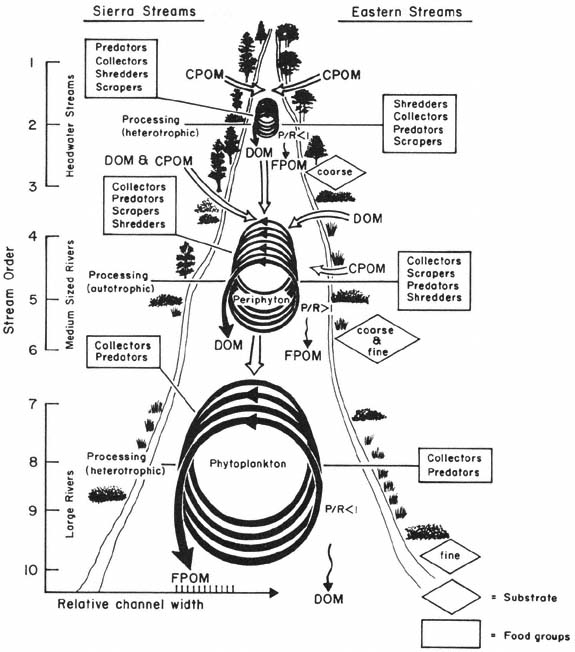
Figure 2.
The relationship between stream size and the progressive shift in structural and functional
components of streams. Box graphs of feeding groups are provided to compare Sierra streams
with eastern streams. The relative number of organisms in each feeding group is indicated
by rank-ordered lists, from large to small (from Cummins 1975; Vannote et al . 1980).
Riparian vegetation has little direct influence on large rivers (orders > 6) since the wide channels are open to sunlight, and the input of terrestrial detritus relative to water volume is small. However, FPOM from upstream sources is very important, and for this reason collectors are the predominant aquatic organisms of large rivers. Although these rivers are open to sunlight, increased turbidity restricts both light penetration and primary production by algae on the fine river substrates. Instead, phytoplankton may be important primary producers in the upper water layers, although turbidity may restrict the depth of their production. Therefore, large rivers are thought to be heterotrophic and have a P:R ratio less than one.
Shredders and scrapers are essentially absent because their food resource and coarse substrate are lacking.
Streams on the western slope of the Sierra Nevada typically pass through several plant communities—subalpine forests (conifers), red fir forests, mixed conifer forests, oak woodlands, chaparral, and grasslands—each of which contributes different organic matter inputs and shading effects. In addition, alpine tundra, montane meadows, and montane chaparral may be locally important. It is not known if all aspects of the river continuum concept apply to Sierra streams.
It is possible to summarize predictions of the river continuum concept (Vannote etal . 1980), especially as they are thought to be true for many streams in forested regions. Exceptions are known to occur for desert streams (Minshall 1978), and possibly for western montane streams (Wiggins and Mackay 1978). Some of these predictions have recently been tested in four Oregon streams, and shown to support the river continuum concept (Naiman and Sedell 1980; Hawkins and Sedell 1981).
Width, depth, and discharge increase as stream order increases. Substrate size changes from coarse to fine going from headwaters to large rivers. Diel changes in water temperature increase to a maximum in medium stream orders (3–5), then decrease downstream.
CPOM and riparian vegetation shading decrease in importance downstream, and FPOM increases in importance. This causes the CPOM:FPOM ratio to decrease as stream order increases. The particle size of detritus decreases downstream.
DOM diversity decreases downstream as labile components are used by microorganisms, causing refractory components to accumulate.
P:R ratio < 1 for stream orders 1–3—heterotropic condition.
P:R ratio > 1 for stream orders 4–6—autotrophic condition.
P:R ratio < 1 for stream orders > 6—heterotrophic condition.
Shredders decrease downstream as CPOM becomes less abundant.
Collectors increase downstream as FPOM becomes more important.
Scrapers increase to a maximum abundance in medium-sized rivers (orders 4–6) as the canopy opens and admits light to the substrate, but then decrease in larger rivers (orders > 6) because turbid water shades algae on the stream substrate.
Predators maintain approximately constant abundance along the continuum.
Biotic diversity is low in the headwaters, increases to a maximum in medium stream orders (3–5), and decreases in larger rivers.
Effects of Riparian Vegetation Removal
Some of the major inputs of riparian vegetation to instream systems are shown in figure 3. Effects on stream invertebrates of disruptions to five of these inputs will be discussed: 1) decrease of detrital inputs; 2) loss of shade as it affects primary production; 3) loss of shade as it affects stream temperature; 4) water quality and quantity alterations; and 5) loss of terrestrial habitat. The intensity of these effects is related to the degree of modification of the vegetation.
Decrease of Detrital Inputs
Riparian vegetation often supplies large amounts of organic matter (energy) to the stream, forming a dependable food base for stream invertebrates year after year. Many of these animals have complex structures, behaviors, and life cycle events which are specially adapted for using different kinds and sizes of detritus as food. Decrease of detritus will cause decreased populations of these species, although instream production may still maintain some at lower densities.
Loss of Shade
Effect on Primary Production
Riparian vegetation is a major control on light intensities reaching algae and macrophytes in headwater streams, and therefore on the level of primary production that can occur. Shade removal has been demonstrated to increase primary production and cause algal mats in small streams, both in the field (Brown and Krygier 1970; Likens etal . 1970; Granoth 1979), and in the laboratory (McIntire and Phinney 1965; Brocksen etal . 1968). For example, vegetation removal along a small stream in Kansas changed it from heterotrophy to autotrophy (Gelroth and Marzolf 1978). Also, in laboratory streams exposed to two different light levels, the stream receiving twice as much light had twice the gross plant production (Brocksen etal . 1968). If nutrients or other factors are not limiting, increased illumination due to shade removal will increase primary production and the food resources used by scrapers.
Loss of Shade
Effect on Stream Temperature
Shade from riparian vegetation moderates stream temperatures, often preventing excessive summer temperatures that may be lethal to invertebrates or fish. Field studies have demonstrated significant increases in summer water temperatures and decreases in winter temperatures when shade is removed from small streams (table 1).
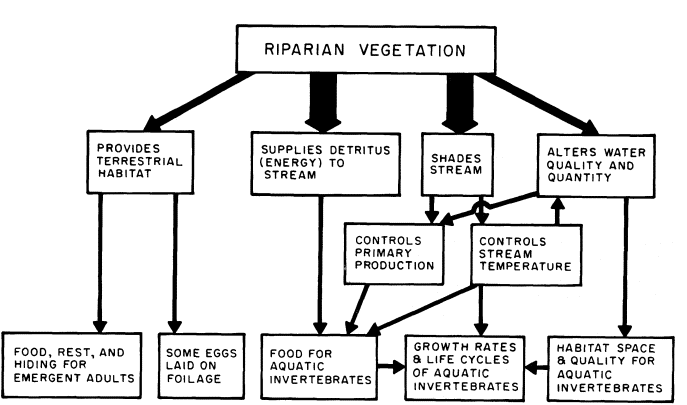
Figure 3.
Relationships between riparian vegetation and stream components.
|
Studies on clear-cut watersheds show that when riparian buffer strips are used, stream temperatures remain essentially the same as in undisturbed watersheds (Brown and Krygier 1970; Swift and Messer 1971; Graynoth 1979), and stream macroinvertebrate diversities remain high (Erman etal . 1977).
Water temperature affects numerous important stream functions, such as processing rates of organic matter, chemical reactions and concentrations, metabolic rates of stream invertebrates, and cues for life cycle events. Because of these complex interactions it is very difficult to assess the ultimate effects of shade removal and water temperature changes on stream animals. Stream invertebrates have different tolerances for water temperature variations, but most species in headwater streams are narrowly adapted for cool temperatures and may use dormant strategies to survive natural warm periods (Hynes 1970). Some stoneflies in Sierra streams survive summer temperatures lethal to mature nymphs by being in the egg stage or by diapausing as young nymphs. Although some survival strategies can be used whenever conditions become unfavorable, other strategies are closely timed to natural stream temperature cycles and might not be useable during abnormal temperature patterns.
Water Quality and Quantity Alterations
Riparian vegetation affects water quality not only by moderating water temperature and influencing chemical reactions, but also by contributing DOM and nutrients to the stream. Riparian vegetation also protects streambanks from excessive erosion, minimizing the input of fine sediments which can fill the numerous cracks, crevices, narrow channels, and openings that ramify through the upper substrate layers that form the invertebrate habitat of normal headwater streams. Removal of riparian vegetation may increase the annual amount of stream runoff, increase peak discharges after rainstorms, and change the timing of peak flows. Change in runoff quantity will cause the stream channel to readjust its velocity patterns, channel dimensions, frequency of pools and riffles, and substrate composition, all of which are important for the amount and quality of invertebrate habitat. Since invertebrate species vary in their habitat requirements, some species may benefit while others are harmed. Nonetheless, disturbances that add fine sediment to streams decrease the species diversity.
Loss of Terrestrial Habitat
Most aquatic insects, including those in Sierra streams, emerge into terrestrial ecosystems as adults with wings for dispersing and searching for mates. Riparian vegetation is an important habitat used by these adult insects for feeding, resting, and hiding. Use of this foliage is heavy in spring and somewhat less in summer and autumn. Some use occurs even in winter. Without this vegetation, predation by birds, terrestrial insects, and mammals would undoubtedly be much greater. Some insect adults lay eggs on riparian vegetation overhanging the stream so that upon hatching the young larvae will drop back into the stream for the aquatic life stages. This method of egg laying is used by the alderfly Sialis in Sierra streams. Stoneflies signal and find mates by drumming with their abdomens on streamside vegetation.
Literature Cited
Anderson, N.H., and J.R. Sedell. 1979. Detritus processing by macroinvertebrates in stream ecosystems. Ann. Rev. Entomol. 24:351–377.
Aubertin, G.M., and J.H. Patric. 1974. Water quality after clearcutting a small watershed in Western Virginia. J. Environ. Qual. 3:243–249.
Bray, J.R., and E. Gorham. 1964. Litter production in forests of the world. p. 101–157. In : J.B. Cragg (ed.). Advances in ecological research, Vol. 2. 264 p. Academic Press, New York, N.Y.
Brocksen, R.W., G.E. Davis, and C.E. Warren. 1968. Competition, food consumption, and production of sculpins and trout in laboratory stream communities. J. Wildl. Mgmt. 32:51–75.
Brown, G.W., and J.T. Krygier. 1970. Effects of clear-cutting on stream temperature. Water Resources Res. 6:1133–1139.
Busch, D.E., and S.G. Fisher. 1981. Metabolism of a desert stream. Freshwat. Biol. 11:301–307.
Cummins, K.W. 1973. Trophic relations of aquatic insects. Ann Rev. Entomol. 18:183–206.
Cummins, K.W. 1974. Structure and function of stream ecosystems. Bioscience 24:631–641.
Cummins, K.W. 1975. The ecology of running waters: theory and practice. p. 277–293. In : Proceedings of Sandusky River Basin symposium. International Joint Commission of the Great Lakes. Heidelberg College, Tiffin Ohio.
Cummins, K.W., and G.L. Spengler. 1978. Stream ecosystems. Water Spectrum 10:1–9.
Cummins, K.W., and M.J. Klug. 1979. Feeding ecology of stream invertebrates. Ann. Rev. Ecol. Syst. 10:147–172.
Erman, D.C., J.D. Newbold, and K.B. Roby. 1977. Evaluation of streamside bufferstrips for protection aquatic organisms. Water Resources Center Contract No. 165. 48 p. University of California, Davis.
Fisher, S.G., and G.E. Likens. 1973. Energy flow in Bear Brook, New Hampshire: an integrative approach to stream ecosystem metabolism. Ecol. Monog. 43:421–439.
Gelroth, J.V., and G.R. Marzolf. 1978. Primary production and leaf-litter decomposition in natural and channelized portions of a Kansas stream. Am. Midl. Nat. 99:238–243.
Graynoth, E. 1979. Effects of logging on stream environments and faunas in Nelson. New Zealand J. Mar. Freshwat. Res. 13:79–109.
Greene, G.E. 1950. Land use and trout streams. J. Soil Wat. Cons. 5:125–126.
Hawkins, C.P., and J.R. Sedell. 1981. Longitudinal and seasonal changes in functional organization of macroinvertebrate communities in four Oregon streams. Ecology 62:387–397.
Hobbie, J.E., and G.E. Likens. 1973. Output of phosphorus, dissolved organic carbon, and fine particulate carbon from Hubbard Brook watersheds. Limnol. Oceanogr. 18:734–742.
Hynes, H.B.N. 1963. Imported organic matter and secondary productivity in streams. Proc. XVI Int. Congr. Zool. 4:324–329.
Hynes, H.B.N. 1970. The ecology of running waters. 555 p. University of Toronto Press, Toronto, Canada.
Hynes, H.B.N., N.K. Kaushik, M.A. Lock, D.L. Lush, Z.S.J. Stocker, R.R. Wallace, and D.D. Williams. 1974. Benthos and allochthonous organic matter in streams. J. Fish. Res. Bd. Can. 31:545–553.
Lee, R., and D.E. Samuel. 1976. Some thermal and biologial effects of forest cutting in West Virginia. J. Environ. Qual. 5:362–366.
Levno, A., and J. Rothacher. 1967. Increases in maximum stream temperatures after logging in old-growth douglas fir watersheds. USDA Forest Service Research Note PNW-65. 12 p. Pacific Northwest Forest and Range Experiment Station.
Levno, A., and J. Rothacher. 1969. Increases in maximum stream temperatures after slash burning in a small experimental watershed. USDA Forest Service Research Note PNW-110. 7 p. Pacific Northwest Forest and Range Experiment Station.
Likens, G.E., F.H. Bormann, N.M. Johnson, D.W. Fisher, and R.S. Pierce. 1970. Effects of forest cutting and herbicide treatment on nutrient budgets in the Hubbard Brook Watershed-Ecosystem. Ecol. Monog. 40:23–47.
Lush, D.L., and H.B.N. Hynes. 1973. The formation of particles in freshwater leachates of dead leaves. Limnol. Oceanogr. 18:968–977.
McIntire, C.D., and H.K. Phinney. 1965. Laboratory studies of periphyton production and community metabolism in lotic environments. Ecol. Monog. 35:237–258.
Meehan, W.R., W.A. Farr, D.M. Bishop, and J.H. Patric. 1969. Some effects of clearcutting on salmon habitat of two southeast Alaska streams. USDA Forest Service Research Paper PNW-82. 45 p. Pacific Northwest Forest and Range Experiment Station.
Minshall, G.W. 1968. Community dynamics of the benthic fauna in a woodland springbrook. Hydrobiol. 32:305–339.
Minshall, G.W. 1978. Autotrophy in stream ecosystems. Bioscience 28:767–771.
Naiman, R.J. 1976. Primary production, standing stock, and export of organic matter in a Mohave Desert thermal stream. Limnol. Oceanogr. 21:60–73.
Naiman, R.J., and J.R. Sedell. 1979. Benthic organic matter as a function of stream order in Oregon. Arch. Hydrobiol. 87:404–422.
Naiman, R.J., and J.R. Sedell. 1980. Relationships between metabolic parameters and stream order in Oregon. Can. J. Fish. Aquat. Sci. 37: 834–847.
Swift, L.W., and J.B. Messer. 1971. Forest cuttings raise temperatures of small streams in the southern Appalachians. J. Soil Wat. Cons. 26:111–116.
Vannote, R.L., G.W. Minshall, K.W. Cummins, J.R. Sedell, and C.E. Cushing. 1980. The river continuum concept. Can. J. Fish. Aquat. Sci. 37:130–137.
Wallace, J.B., and R.W. Merritt. 1980. Filter-feeding ecology of aquatic insects. Ann. Rev. Entomol. 25:103–132.
Wiggins, G.B., and R.J. Mackay. 1978. Some relationships between systematics and trophic ecology in Nearctic aquatic insects, with special reference to Trichoptera. Ecology 59:1211–1220.