15.3—
Steady States of Growth and Metabolism of Plant Cells in Continuous Culture
Reference was made above to the short period of exponential growth observed in batch cultures. However, even during this phase of the growth cycle, the cells do not achieve a steady state (a state of balanced growth); cell division is uncoupled from increase in cell dry weight and protein content so that the cells are changing in size and composition despite the constancy of the double time of the culture (Fig. 15.2). Such observations raised the question of whether balanced growth could be achieved in plant cell cultures if a constant culture environment could be established by developing open continuous culture
systems (systems in which inflow of fresh medium is balanced by outflow of an equal volume of culture). Wilson, King & Street (1971) have developed such a system providing conditions of aeration and agitation appropriate to plant cell cultures, capable of long-term aseptic operation and functional either as a chemostat or as a turbidostat.
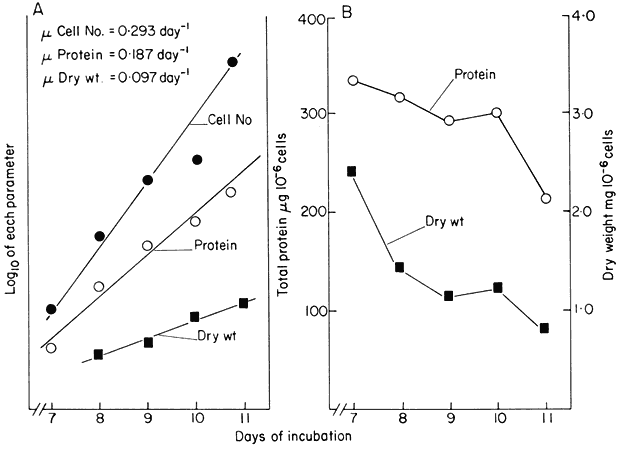
Figure 15.2
Unbalanced growth of sycamore cells (Acer pseudoplatanus L)
during the transient exponential growth phase achieved in batch culture.
(a) Semilogarithmic plots showing rate of change in cell number, total protein
and cell dry weight per unit volume of culture. The slopes of the lines of best
fit (calculated by linear regression analysis, P < 0.01) were used to determine
the specific growth rates (µ) for each parameter.

where x0 = initial value of parameter, x = value after time t(days). When
log10x plotted against t , slope = µ/2.303. (b) Changes in total protein
and cell dry weight per 106 cells with time calculated from data in A.
(From King & Street, 1973.)
15.3.1—
Chemostat Cultures
Subsequent work (King & Street, 1973; King Mansfield & Street, 1973) with this system operated as a chemostat (where equilibrium is established at a fixed rate of imput of a growth-limiting nutrient) has shown that long-term steady states of growth can be achieved with plant cell cultures and that such cultures conform to the chemostat theory developed from work with microorganisms (Monod, 1950; Novick & Sziland, 1950). In a chemostat, the relationship between cell density, x (cells per unit volume of culture), dilution rate, D (volume
of new medium added per unit time expressed as a fraction of the total culture volume), specific growth rate, µ (increase in biomass per unit biomass per unit time) and time (t ) is given by the equation

When equilibrium is reached and a steady state established dx/dt = 0, µ = D and x has a value characteristic of the dilution rate. Further, the nutritive environment remains constant, each nutrient achieving an equilibrium concentration which is related to its imput concentration and the rate of its consumption by the culture. As defined above, the equilibrium achieved in a chemostat culture results from one particular nutrient (depending upon the composition of the culture medium) becoming the limiting nutrient and determining the specific growth rate (µ) of the cells.
The nature of these steady states can be illustrated from work involving sycamore cell cultures growing in a synthetic medium (Stuart & Street, 1969) in which the supply of nitrogen is the limiting factor. Data for one such steady state (D = 0.194 day–1 ) is presented in Fig. 15.3. This shows that the cells are in a balanced state of growth and metabolism (as illustrated by the values for cell number, packed cell volume, cell dry weight, protein, DNA and RNA, and oxygen demand) and that the nutrient medium within the culture vessel is constant in composition (as illustrated by constancy of culture pH and the levels of glucose, phosphate and nitrate). Such steady state cells also display constant levels of metabolites (e.g. amino acids; Street, Gould & King, 1975) and constant levels of activity of individual enzymes (e.g. enzymes concerned with carbohydrate respiration; Fowler & Clifton, 1974; and with nitrogen assimilation; Young, 1973).
Such chemostat cultures can be operated from very low growth rates (i.e. low dilution rates) to growth rates approaching the maximum growth rate (µmax ) for the culture medium chosen, provided dilution rate is such that the cells can still achieve a matching growth rate. Of course if dilution rate is further increased, cell density does not stabilize and the culture suffers wash-out. Cells in balanced growth but highly contrasted in growth rate (and hence in cytology and metabolism) can therefore be obtained by chemostat culture. The range of change in certain cell parameters in sycamore cell cultures at different dilution rates (and hence specific growth rates) over the range D = 0.06–0.236 day–1 (corresponding to double times over the range 280–70 hr) is illustrated in Fig. 15.4. What this means is that it is possible to stabilize at will, by fixing dilution rate at an appropriate level, the individual physiological states which have only a transient existence in batch culture.
Work with chemostat cultures has shown that the same cell population can be taken through a series of steady states and then if the dilution rate is returned to that of an earlier steady state the cells again achieve not only the new predictable growth rate but also the physiological activities earlier recorded as
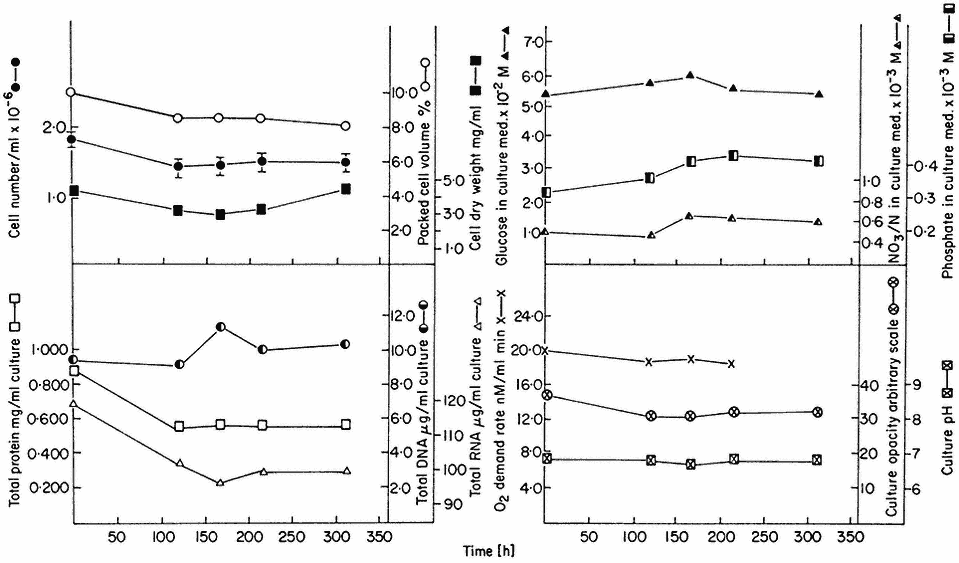
Figure 15.3
A steady state established in a 4-litre chemostat culture or A. pseudoplatanus cells. The
culture was diluted for 400 hrs. at a rate of 0.194 culture volumes per day. Samples were withdrawn
at intervals for biomass measurements, determinations of nutrient levels in the culture medium and for
respiration rate measurements. Culture opacity and pH were monitored continuously in the culture vessel.
(From King & Street, 1973.)
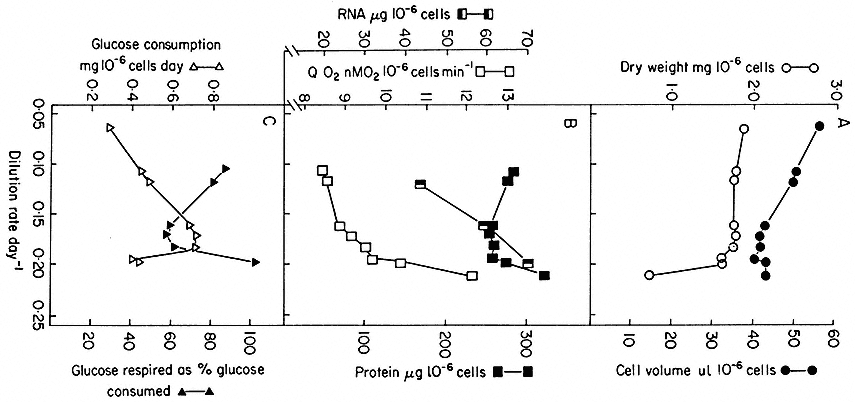
Figure 15.4
Steady state values for parameters of cell composition and physiological activities recorded
over a range of growth rates (as expressed by dilution rates) established in chemostat cultures
of sycamore cells (A. pseudoplatanus ) with nitrate/N as the limiting nutrient. The relationship
between dilution rate, D (fraction of culture volume displaced per unit time), specific growth rate
(m ) and doubling time (td) of the cell population in a steady state is given by

(From King & Street, 1973.)
characteristic. Such experiments demonstate the full reversibility of the cytological and physiological changes invoked. Interest will now focus on detailed studies of the kinetics of the transition between steady states in terms of enzyme activities and metabolite levels. Preliminary studies along these lines have already shown that during these transitions there are pronounced oscillations in enzyme activity levels (characteristic for each enzyme monitored) which gradually decline in amplitude as the new steady state is established. How far this behaviour is to be explained in terms of changes in rates of enzyme synthesis and degradation has yet to be determined. Clearly study of these transitions will yield entirely new data on metabolic regulation in higher plant cells; whether it will yield the key to expose the changes underlying cytodifferentiation is less certain.
15.3.2—
Turbidostat Cultures
The constancy of culture opacity in the steady state described by Fig. 15.3 has formed the basis for a second form of continuous culture—the turbidostat system (a continuous system in which inflow of new medium occurs in response to an increase in the opacity-decrease in the light transmission of the culture). The turbidostat culture system developed for work with sycamore cell cultures is shown in Fig. 15.5 (Wilson, King & Street, 1971). Here, each time the population density exceeds a pre-selected value as determined by the optical density continuously monitored by the photocell, an electronically operated valve opens to admit a pulse of new medium. This reduces the optical density of the culture and the valve closes. These imputs of new medium are balanced by periodic harvesting of small volumes of culture in response to an electronically controlled level detector which controls the output valve. The effect is to produce a culture of constant volume and constant population density growing at a constant rate. Whereas in the chemostat growth rate must always be below µmax (the culture growth being limited by the supply of a chosen nutrient) here in the turbidostat growth can safely proceed under non-limiting nutrient conditions and one can study the effect of physical factors (e.g. temperature, light regime, CO2 tension) and growth regulators, in particular plant growth hormones, on growth rate (King, 1976), and by appropriate techniques (Gould, Baylis & Street, 1974) on the duration of the different phases of the cell cycle. This is the system where one can attempt to achieve conditions under which biomass increase expresses the maximum genetic potential for cell growth. Such a system offers an entirely new approach to studies on the molecular basis of the hormonal control of cell growth.