2—
Radiochemistry
Gluttony at the Periodic Table
The Laboratory's earliest radiochemistry, apart from radiosodium, concerned nitrogen and oxygen. These elements lent themselves to experiment: they could be obtained very pure and deployed without fear of surface contamination; they have few
[25] Lawrence to A.H. Compton, 14 Mar 1939 (4/10), and to F.C. Blake, 12 Jul 1940 (3/1).
[26] Lawrence, "Report to the National Advisory Cancer Council," 12 Oct 1939 (13/29A); Aebersold to Zirkle, 19 Aug 1939 (1/9).
naturally occurring isotopes to confuse analysis; and they are easily excited by deuterons at 2 or 3 MeV. The experimental setup, which remained standard for gases, is indicated in figure 8.1. The first to work it were Lawrence, Henderson, and McMillan. They attacked the air and detected three groups of alpha particles and two of protons, which they assigned to reactions of nitrogen, but found no radioelements and did no chemistry. The ranges they measured for the alpha particles and protons did not agree with more careful determinations by Cockcroft; once again Lawrence had to remeasure and retract. McMillan persisted, substituted nitrogen for air and Livingston for Lawrence and Henderson, and uncovered a positron activity that lasted about two minutes. A little chemistry showed that the active substance formed water; a little reasoning ascribed the activity to a new radioelement, O15 , half-life 126 seconds, and to the reactions N14 (d,n)O15 , O15® N15 + e+ .[27] In a parallel investigation, Henry Newson, who came from and returned to the Chemistry Department at the University of Chicago, found that F17 (t = 1.16 m)
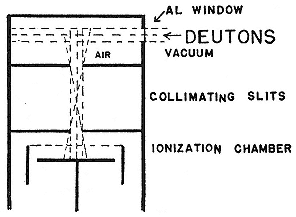
Fig. 8.1
Experimental arrangement for irradiation of gases.
Lawrence, McMillan, and Henderson,
PR, 47 (1935), 276.
[27] Lawrence, McMillan, and Henderson, PR, 47 (1935), 273–7, rec'd 2 Jan; Cockcroft to Lawrence, 28 Sep 1935, and answer, 22 Oct 1935 (4/5); McMillan and Livingston, PR, 47 (1935), 452–7, rec'd 21 Jan, adumbrated in Livingston and McMillan, PR, 46 (Sep 1934), 437–8.
could be made by (d,n) on O16 . He thereby recovered a known activity made by (a ,n) on N14 and raised his reputation in the Laboratory. (Lawrence had judged him to lack the pushiness needed to accomplish anything there.)[28]
Meanwhile McMillan and Lawrence worked on aluminum, which has but one natural isotope, and Henderson attacked magnesium, which has three. They used an apparatus similar to that of figure 8.1 with the target mounted in the beam. Protons, positrons, alpha particles, and neutrons came off aluminum, which could provide them all via the reactions Al27 (d,p)Al28 ® Si28 + e+ , Al27 (d,a )Mg25 , and Al27 (d,n)Si28 . The activity of Al28 (t = 156 sec), the only one studied, was scarcely fresh, having been prepared in France the natural way, by (a ,n) on phosphorus, and in Italy the Italian way, by (n,g ) on aluminum. By placing a series of foils in a line, McMillan and Lawrence measured the "excitation function," the yield of radioaluminum as a function of the energy of the incident deuterons. That brought nothing new either: the excitation function agreed with Gamow's theory.[29]
With a little more energy—3MeV—Lawrence and McMillan, now joined by Thornton, got results that diverged from theory. Oppenheimer became interested, calculated, and concluded that disintegration via (d,p) followed the Oppenheimer-Phillips process at the higher bombarding energies. Meanwhile Henderson was getting two different radioactive products from the heaviest magnesium isotope, Mg26 , that is, Mg27 (t = 10 m) via (d,p) and Lawrence's Na24 via (d,a ). Although both activities were known (Fermi had made Mg27 by neutron capture), Henderson could claim the first case in which two different products resulted from the bombardment of a single isotope by the same charged particle. He determined the excitation functions for both, and consulted Oppenheimer. The sage authorized the conclusion that the Na24 came into existence by Gamow capture and the Mg27 by the mechanism of Oppenheimer and Phillips.[30]
[28] Newson, PR, 48 (1935), 790–6, rec'd 3 Sep; Lawrence to W.D. Harkins, 14 Oct 1935 (13/43).
[29] McMillan and Lawrence, PR, 47 (1935), 343–8, rec'd 12 Jan; Seaborg, Jl., 1 , 282–3 (18–19 Oct 1937).
[30] Lawrence, McMillan, and Thornton, PR, 48 (1935), 493–9, rec'd 1 Jul; Henderson, ibid., 855–61, rec'd 16 Sep.
The Laboratory's appetite at its first sitting at the periodic table appears from the menu of the meeting of the American Physical Society held in Berkeley at the end of December 1935. Cyclotroneers gave twelve talks, only one of which concerned machinery. Otherwise the subjects were elements excited by deuterons: copper, nitrogen, and oxygen, whose excitation functions Newson followed to energies above the nuclear potential barrier, where the Gamow curve no longer holds; phosphorus, argon, nickel, cobalt, zinc, and arsenic, made radioactive by Paxton, Snell, Thornton, and Livingood; nitrogen, fluorine, sodium, aluminum, silicon, phosphorus, chlorine, argon, and potassium, whose beta and gamma emissions gave employment to Kurie, Richardson, Paxton, Cork, and their cloud chamber. For most of this work, deuteron energies ran about 3.5 MeV and the elements studied were no heavier than arsenic (atomic number, Z , = 33). This was a little tame and routine for the boss. Lawrence's name appeared on two papers at the APS meeting. In one, with Cooksey and Kurie, he described improvements in the cyclotron that resulted in 6 MeV deuterons; in the other, with James Cork, he announced the discovery that platinum nuclei (Z = 78!) "resonated" when hit by such rapid particles. This response from the tough platinum nucleus, which he thought he had "transmuted" to gold, was most gratifying. As Lawrence wrote the Macy Foundation in October 1935, six months earlier he would not have thought such alchemy possible with energies attainable in the Laboratory.[31]
During 1936 the Laboratory worked its way forward from iron, using the faster deuterons then available and trusting in the efficiency of the Oppenheimer-Phillips process apparently so potent in aluminum. (In fact, as Bethe later showed in an elaborate, but approximate, calculation, the Oppenheimer-Phillips process would not have been detectable below Z = 30.)[32] At the
[31] Abstracts of papers at APS, 20–21 Dec 1935, in PR, 49 (15 Jan 1936), 203–9; Lawrence to Rutherford, 10 Jul 1935 (ER); to Shenton, 10 Oct 1935 (12/32); to Cockcroft, 2 Oct and 25 Nov 1935 (4/5), and to Chadwick, 27 Nov 1935 (3/34). Newson's definitive results were published after he left Berkeley, in PR, 51 (1937), 620–3.
[32] Bethe, PR, 53 (1938), 42, 47–9, previewed in Bethe, RMP, 9 (1937), 201–4. Oppenheimer's calculation had presupposed a deuteron more weakly bound thanthe best measurements suggested and a Coulomb repulsion effective to vanishingly small distances; both presuppositions favor Oppenheimer-Phillips.
meetings of the spring and early summer of 1936, Van Voorhis introduced a duplicitous copper, which, having imbibed a neutron perhaps in the manner of Oppenheimer-Phillips, decays by either a positron to nickel or an electron to zinc; he thereby found much, but missed more, since the predominant mode of decay of Cu64 is via a process, K-electron capture, then undetected. Livingood reported on the unseparated messes he made with 5 MeV deuterons on several metals and also on his attempt, fleetingly successful, to make the first artificial-natural radioelement (Bi210 , alias RaE) via the Oppenheimer-Phillips process Bi209 (d,p)Bi210 . He thought he glimpsed the faint beta decay of RaE, and also alpha particles of the right range to arise from RaE's descendent Po210 .[33] The man who first identified RaE, Rutherford, was delighted to know that Lawrence could make a, or perhaps any, link of a naturally occurring radioactive series. "[It is] a great triumph for your apparatus."[34]
Another triumph seemed in the offing. Cork had continued with the experiments on platinum. Together he and Lawrence identified four activities, two arising (according to them) from platinum isotopes excited by an unknown process more powerful than Oppenheimer-Phillips and two from irridium isotopes produced by an unlikely (d,a ) reaction. They disclosed further that the excitation function of platinum did not increase monotonically with energy, but showed several bumps or resonances.[35] Oppenheimer developed a new theory to account for it all. At this point, in April 1937, Niels Bohr passed through Berkeley on his way to Japan.
Bohr had just put the finishing touches on his theory likening the nucleus to a liquid drop with modes of excitation incompa-
[33] Van Voorhis, PR, 49 (1 June 1936), 876, and Livingood, ibid., APS meeting of 30 Apr–2 May, in Washington; Livingood, PR, 50 (15 Aug 1936), 385, 391, APS meeting of 17–19 June, in Seattle; Livingood, PR, 50 (1936), 432–4, rec'd 29 June.
[34] Rutherford to Lawrence, 22 Feb 1936 (15/24). Cf. McMillan, PT, 12:10 (1959), and Oliphant, PT, 19:10 (1966), in Weart and Phillips, History , 264, 186–7, resp.
[35] Cork and Lawrence, PR, 49 (15 Jan 1936), 205, and ibid., 788–92, rec'd 7 Apr.
tible with Lawrence's platinum resonances. At a seminar arranged especially to discuss the latest curiosity of Berkeley experiment and theory, Lawrence announced that his measurements conflicted with Bohr's ideas, but agreed perfectly with Oppenheimer's, and Oppenheimer gave what Kamen remembered as "a typically stupefyingly brilliant exposition of its theoretical consequences." Bohr declared that the data had to be wrong. When he left, the resonances became sharper, the experiments more convincing.[36]
Lawrence did not wish to repeat the saga of the disintegrating deuteron. He summoned McMillan, who had once traced an apparent activity of platinum under deuteron bombardment to radioactive nitrogen driven by recoil into the surface of the metal. McMillan realized that he needed chemical advice (the separations on which Lawrence and Cork had relied were hurriedly done by Newson during his last days at the Laboratory). He called on Kamen and a new graduate student in chemistry, Samuel Ruben. It took them over three months of strenuous chemical work—eighteen hours at a time—to separate the activities and to trace the exotic "resonances" to just plain dirt. By rubbing Laboratory grime into platinum foils before bombarding them, Kamen was able to reproduce most of Cork's measurements.[37]
Products
In the summer of 1936, Segrè, then newly appointed professor of physics at the University of Palermo, visited Berkeley, to see the cyclotron, to escape the heat at his main place of sojourn, Columbia, and to survey possibilities of escaping from the heat in Italy should war threaten. He returned to Palermo "still dreaming of the cyclotron" and carrying some bits of copper strip that he had scavenged from the chamber of the 27-inch. He and his associates separated radioisotopes of copper, zinc, and perhaps manganese from the scrap. 'Twas but antipasto. "We would like very
[36] Nahmias to Joliot, 28 Apr 1937 (JP, F25); Kamen, Radiant science , 76–8; Lawrence to Segrè, 5 Apr 1937 (16/14), and to Nishina, 13 Apr 1937 (9/38); McMillan and Livingston, PR, 47 (1935), 454.
[37] Lawrence to Oppenheimer, 26 Apr 1937 (14/9), to Segrè, 28 May 1937 (16/4), and to Bohr, 8 Jul 1937 (3/3).
much to have more copper," Segrè wrote. "I think you can send any substance in a letter."[38] Meanwhile the cyclotron had been opened for repairs. Lawrence salvaged more copper and the molybdenum strip that protected the dee edge at the exit slot. He had it all cut up and sent in several letters. It was an act of headstrong generosity. Lawrence suspected that the molybdenum contained an activity of long life but of too little promise to add its investigation to the rigorous Berkeley routine. "We are all very busy here, but there is nothing very exciting at the time to report."[39] There would have been some excitement had they kept the hot molybdenum.
If the long activity arose via (d,n) on molybdenum, it belonged to element 43. Number 43, alias davyum, lucium, nipponium, and masurium, had been nondiscovered several times. No trace of it had turned up in the surveys of x-ray spectra by H.G.J. Moseley and his successors; the only evidence for its existence when Lawrence sent Segrè his second installment of scrap was three faint x-ray lines observed by Walter and Ida Noddack in 1925 in the course of their successful detection of element 75 (rhenium). Segrè and Carlo Perrier, Palermo's professor of mineralogy and an accomplished analytical chemist, took the Laboratory's molybdenum apart. They separated a large amount of radiophosphorus, which they found to contaminate everything from Berkeley, and handed it to colleagues in physiology to administer to rats.[40] They tried to carry the residual activity on molybdenum and the elements immediately below it, niobium and zirconium, but to no avail; its chemistry was closer to that of rhenium, the heavy homologue of "masurium." In April Segrè notified Lawrence: "All the activity is due to some substances which have all chemical characters one would expect to find in the element 43." Perrier and Segrè saw indications of three different radioisotopes of 43, to which the Palermo group soon attached half-lives of 90, 50, and 80 days, in order of relative abundance. As for stable isotopes of "masurium," Perrier and Segrè declared
[38] Segrè to Lawrence, 9 Sep, 2 Nov, 18 Dec 1936 (16/14), and Mezzo secolo , 23–4, 58–9.
[39] Lawrence to Segrè, 6 Jan 1937 (16/14); McMillan, PT, 12:10 (1959), in Weart and Phillips, History , 265; Segrè, Ann. rev. nucl. sci., 31 (1981), 8.
[40] Nature, 139 (1937), 836–7, 1105–6; Kirby, in Gmelins Handbuch , 2–3.
them to be "absent," indeed, nonexistent; no known element could be invoked to act as a carrier for the new activity.[41] Consequently, just after the war, when weighable amounts of element 43 were created in nuclear reactors, Perrier and Segrè annihilated "masurium" and named their element, the first made by man before its discovery in nature, "technetium."[42]
"The cyclotron evidently proves to be a sort of hen laying golden eggs." So Segrè wrote Lawrence at the start of the analysis of the molybdenum strip. He publicly acknowledged his gratitude not only by the usual professional thanks, but also, what Lawrence no doubt preferred, by advertising the cyclotron. Perrier and Segrè concluded their presentation of the radiochemistry of element 43: "We hope also that this research carried on months after the end of the irradiation and thousands of miles from the cyclotron may help to show the tremendous possibilities of this instrument." Segrè asked for more active long-lived material and sent some purified uranium oxide for irradiation by slow neutrons in the hope of making more unnatural elements, perhaps alpha emitters from transurania. "I think that when you are producing neutrons every point near to the cyclotron gets a stronger irradiation than with the most powerful sources available in Europe."[43]
Lawrence at first was more impressed by the salvage and application of P32 than by the news of element 43: "We are all amazed here to to see the amount of good work you have done with such a trivial amount of radiophosphorus." As for claims based on complex radiochemical analysis, he had reason to be wary: "Of course there are difficulties."[44] By the fall of 1937, when sending P32 for Segrè's colleagues and more scrap for Segrè himself, he wrote, with his usual optimism: "We are only too glad to send you
[41] Segrè to Lawrence, 28 Apr 1937 (16/14); Perrier and Segrè, Nature, 140 (1937), 193–4, letter of June 13, and Jl. chem. phys., 5 (1937), 713–4, rec'd 30 June, confirmed ibid., 7 (1939), 155; Cacciapuoti and Segrè, PR, 52 (1937), 1252–3, letter of 17 Nov; Segrè in Nicolini et al., Technetium , 5–6.
[42] Kirby, in Gmelins Handbuch , 7; Perrier and Segrè, Nature, 159 (1947), 24. The first traces of naturally occurring technetium, arising from the spontaneous fission of U , were found in 1961; Alleluia and Keller, in Gmelins Handbuch , 12–3.
[43] Segrè to Lawrence, 7 Feb, 13 June, and 1 Jul 1937 (16/14); Perrier and Segrè, Jl. chem. phys., 5 (1937), 716.
[44] Lawrence to Segrè, 5 Apr and 28 May 1937 (16/14).
material because you have accomplished so much with what little we have furnished." Indeed, although it was not known at the time, Segrè had accomplished precisely what Lawrence had prepared: the creation of new materials interesting in themselves and applicable to medicine. The element has been in clinical use since 1963. One isotope, Tc99 , which gives a useful gamma ray, is an important agent in visualizing tumors and abscesses of the liver, in imaging the living skeleton, and in brain scanning. Its production by accelerators became the basis of a multi-million dollar industry.[45]
As Aristotle said, the road from Athens to Corinth runs also from Corinth to Athens. In the case of technetium, Europeans detected a new element in material made in Berkeley. In the case of the isobars of mass three, the Laboratory made a discovery prepared in Europe. Soon after Rutherford and his collaborators had identified the d-d reactions, the Cavendish and also Fritz Paneth and G.P. Thomson at Imperial College, London, sought to produce enough H3 and He3 in Cockcroft-Walton accelerators to investigate their physical and chemical properties. All failed. Rutherford supposed that the elusive isobars combined with stray protons and neutrons to form alpha particles, of which, however, no trace could be found.[46] Efforts to detect He3 by the spectroscope also failed, except in passing and at Princeton, where physicists saw and then did not see lines in the spectra of the products of d-d reactions ascribable to a light helium isotope.[47] By 1935 the case of the uncollectible isobars was attracting attention on both sides of the Atlantic.
The most promising route appeared to be the isolation of "triterium," as Rutherford called it, from heavy water. This quest rested on the assumption that H3 is stable. Weighing in its favor was a report by Tuve's group of the presence of tritium in the
[45] Lawrence to Segrè, 20 Oct, 23 Nov, 23 and 27 Dec 1937 (16/14); Kirby, in Gmelins Handbuch , 8; A. Seidel, in ibid., 308–9; NRC, Phys. persp., 1 , 138.
[46] Oliphant, Harteck, and Rutherford, PRS, A144 (1934), in Rutherford, CP, 3 , 395; Rutherford, Nature, 140 (1937), in CP, 3 , 427–8 (1937); Paneth and Thomson, Nature, 136 (1935), 334.
[47] Harnwell, Smyth, and Urey, PR, 46 (1934), 437; Smyth et al., PR, 47 (1935), 800–1. Seaborg also planned a try; Jl., 1 , 46 (29 Jan 1935). For a time Lewis thought he had H ; Lawrence to Stern, 20 Nov 1933 (16/49).
heavy water made by Urey and F.G. Brickwedde and the apparent slight excess in mass of lightest helium over heaviest hydrogen.[48] But Tuve's result had not been duplicated and the argument from the masses was far from secure. Although the Cavendish's values of the energies and masses entering into the reaction H2 (d,p)H3 were regarded as so reliable that the mass of H3 determined from them stood as one of the most certain of nuclear constants, the mass of He3 , deduced from the reaction H2 (d,n)He3 , stumbled over the difficulty of measuring the kinetic energy of the liberated neutron. Rutherford's group made He3 just slightly heavier than H3 ; analysis by the meticulous T.W. Bonner and W.M. Brubaker, who also used the reaction Li6 (d,a )He3 , made the masses the same within experimental error; and Hans Bethe and R.F. Bacher, scrutinizing it all in the spring of 1936, awarded He3 the tenuous excess of about two ten-thousandths of a mass unit.[49] Excess in the atom, as in the human, may be a sign of instability. Already in 1934, the Cavendish physicists conjectured that He3 might transmute into stable H3 by emission of a positron, in the manner then just made fashionable by the studies of Joliot and Curie.[50]
The first in the field were Walker Bleakney and his associates at Princeton, who had electrolyzed 75 tons of ordinary water down to its heaviest cubic centimeter before learning of the Cavendish evidence for the existence of H3 . They set their precious material free in Bleakney's mass-spectrometer, found traces of particles of mass five that they declared to be molecules of H2 H3 , and inferred that H3 constitutes about one part in a billion of ordinary water. Their colleagues at Princeton, G.P. Harnwell and H.D. Smyth, corroborated their finding by running the product of a gas discharge in deuterium into the spectrograph; and, in a further dividend, the Princeton mass-spectroscopists identified He3 in the d-d product as well. A year later Tuve's group again reported
[48] Tuve, Hafstad, and Dahl, PR, 45 (APS, Apr 1934), 840–1.
[49] Oliphant, Kempton, and Rutherford, PRS, A149 (1935), in Rutherford, CP, 3 , 401–3; Bonner to Bethe, 10 Dec 1935 (HAB/3); Bonner and Brubaker, PR, 49 (1936), 19–21; Bethe and Bacher, RMP, 8 (Apr 1936), 87, 147, 197 (the 0.0002 given on 197 disagrees with the 0.0004 on the preceding pages).
[50] Cockcroft to Lawrence, 14 Nov 1934 (5/4).
"stable hydrogen atoms of mass 3 in numerous electrolytic deuterium samples" put through their magnetic analyzer.[51]
The British were unable to duplicate the feats at Princeton and Washington. From Norsk Hydro, which by the mid 1930s had become the world's largest producer of deuterium, the Cavendish received 11 grams of the heaviest and most expensive remains of the electrolysis of 13,000 tons of water. (In 1935 Norsk Hydro-Elektrisk Kvaelstofaktieselskab sold almost pure heavy water for $1.25/g in quantities over 50 grams; in 1938, the price had declined to 75 cents/g for lots of 25 grams.)[52] Aston could not find a drop of heaviest water in the Norwegian stock. Meanwhile, Mark Oliphant and Fritz Paneth and G.P. Thomson had failed to confirm Harnwell and Smyth, and guessed that the Princetonians, who had begun to doubt themselves, had been misled by the release of helium that had been dissolved into the glass walls of their discharge tube.[53] By mid 1937 a consensus of sorts had been reached. "The claims of the Americans . . . were ill-founded," Thomson wrote Rutherford after visiting Princeton. "I am glad that you are coming to a similar conclusion." The next move appeared to Thomson to be to persuade Lawrence to devote a large amount of cyclotron time to irradiating a bucket of heavy water with deuterons in order to search for the spectrum of He3 .[54] Nothing seems to have come of his proposal. The Laboratory had a more certain manufacture than the elusive isobars of mass three.
In July 1939 owing to the idleness of the 60-inch cyclotron as it awaited adequate shielding, the matter was reopened in Berkeley. Luis Alvarez thought to fuse deuterons in the 37-inch and feed the product into the 60-inch, which he would use as a giant mass-
[51] Lozier, Smith, and Bleakney, PR, 45 (1934), 655; Harnwell, Smyth, et al., ibid., 655–6 (both letters dated 21 Apr), and PR, 46 (1934), 437; Bleakney et al., PR, 46 (1934), 81–2; Tuve, Hafstad, and Dahl, PR, 48 (15 Aug 1935), 337; Hafstad and Tuve, PR, 47 (1935), 506.
[52] Lawrence to Urey, 21 Mar 1935 (17/40), and to J.E. Henderson, 27 Aug 1935 (9/5); Cooksey to Snell, 21 Nov 1938 (16/33); Joliot to Norsk Hydro, 11 Mar 1938 (JP, F25).
[53] Paneth and Thomson, Nature, 136 (1935), 334; Smyth et al., PR, 47 (1935), 800.
[54] Thomson to Rutherford, 27 May 1937, and Mann to Thomson, 7 June 1937 (GPT); Rutherford, Nature, 140 (21 Aug 1937), 303–5, in Rutherford, CP, 3 , 424–8.
spectrograph. He apparently accepted Bethe's revaluation of the mass data in 1938, which corrected in the wrong direction made He3 definitely heavier than H3 and allotted light helium a period of 5,000 years. "This would mean that He3 cannot be found in nature."[55] This was to ignore the recomputation made by Bethe and Livingston in 1937, using revised values of the relevant parameters, which lowered the mass difference by a factor of ten; and also the reconsiderations of Bonner, who found that neutrons carried off more energy in H2 (d,n)He3 than he had thought, and lowered the mass of He3 below that of H3 .[56] Rutherford thought it safest to assume the stability of both isobars. Holding with Bethe, Alvarez was alert to any anomaly that indicated that He3 does not have a period of five millennia.[57]
When Alvarez and a graduate student, Robert Cornog, began their search, the magnetic field of the 60-inch cyclotron was set to accelerate alpha particles. As a preliminary check on the background radiation through the machine, Alvarez watched an oscilloscope that monitored the current to the target while the operating crew decreased the field. The current through the cyclotron fell to zero, as expected, when the field no longer held the alpha particles in phase with the radio frequency potential on the dees. At the end of the test, the crew turned off the field after readjusting it for accelerating alpha particles. As the rapidly changing field passed through the setting for mass three, a sudden burst of particles registered on the oscilloscope. The general phenomenon—a momentary spike in a cyclotron beam as the magnet current sweeps rapidly through the resonance point—had been noticed by M.C. Henderson and Milton White, who explained that the changing magnetic flux set up eddy currents in the pole faces that acted like shims.
Alvarez did not expect to see a spike. It made his day ("one of the finest moments of my scientific life"). It indicated particles of mass three in the cyclotron's source. The source was natural
[55] Bethe and Bacher, RMP, 8 (Apr 1936), 197; Bethe, PR, 53 (1938), 313–4.
[56] Bonner, PR, 53 (1938), 711–3, challenged by Rumbaugh, Roberts, and Hafstad, PR, 54 (1938), 675–80, on the basis of Bethe's new numbers.
[57] Livingston and Bethe, RMP, 9 (Jul 1937), 324, 331, 373, 378, 379; Rutherford, Nature, 140 (1937), in CP, 3 , 424; cf. Alvarez, PT, 35:1 (1982), 26.
helium from a deep well in Texas, where it had lain for geologic ages. Evidently He3 had a half-life greater than 5,000 years. In fact, as Alvarez proclaimed, it is stable.[58] He and Cornog shimmed the 60-inch to give a He3 beam and estimated the relative abundance of the light and heavy isotopes as 10–7 for atmospheric, and 10–8 for well helium. (These numbers are low by a factor of ten; the higher percentage in the atmosphere arises from creation of He3 by cosmic rays.) That solved half the old problem. They then looked for radioactive H3 in the product of d-d reactions passed into an ionization chamber. A new long activity with chemical properties of hydrogen rewarded their search.[59]
The most obvious next step was to determine the half-life of the radioactive hydrogen. A first estimate, made by Thanksgiving day, 1939, was 230 days, later diminished to 150 days, and then raised to perhaps ten years. The lower numbers may stand as a warning to unwary experimenters: as Cornog discovered to his chagrin, they measured the rate, not of decay, but of the leak of hydrogen through a rubber tube used in the apparatus.[60] This last number came from McMillan, who had measured the half-life of H3 without discovering it. He had guessed that an activity he had noticed in 1936 and reckoned at ten years belonged to a suppositious Be10 made by (d,p) along with the B10 made by (d,n) in the usual cyclotron irradiation of beryllium. After the disclosure of the activity of H3 , physicists at the University of Chicago got a nice radioactive gas on dissolving a specimen similar to McMillan's. They supposed they dealt with the product of the reaction Be9 (d,H3 )Be8 and that McMillan's half-life characterized tritium.[61] The period is, in fact, 12.6 years. Tritium is constantly
[58] Alvarez and Cornog, PR, 56 (1939), 379, letter of 31 July; Henderson and White, RSI, 9 (Jan 1938), 29–30; Cooksey to Allen, 2 Aug 1939 (1/14); Alvarez, "Adventures," 16–21, PT, 35:1 (1982), 27–8, and Adventures , 68–71, quote.
[59] Alvarez and Cornog, PR, 56 (1939), 613, letter of 29 Aug.
[60] Alvarez and Cornog, abstract of paper for APS meeting, 22 Nov 1939 (2/2), PR, 57 (1940), 249, and PR, 58 (1940), 197; Cornog in Trower, Discovering Alvarez , 26–8.
[61] McMillan, PR, 49 (1936), 875; O'Neal and Goldhaber, PR, 57 (1940), 1086–7. McMillan's sample was lost before it could be tested for H ; Ruben and Kamen, PR, 59 (1941), 349.
made in the atmosphere by cosmic rays. The heavy Norwegian rainwater examined by the Cavendish consequently contained radioactive hydrogen, which lived long enough to reveal itself to a Geiger counter after the war.[62]
Lawrence was delighted with the identification of the isobars of mass three. He gave it pride of place in his report to the Research Corporation on the Laboratory's work for 1939. When the report was submitted early in 1940, negotiations with the Rockefeller Foundation over the 184-inch cyclotron had reached their critical phase. Lawrence gave the result a gloss that he doubtless expected Poillon to pass on to the Foundation. "Radioactively labelled hydrogen opens up a tremendously wide and fruitful field of investigation in all biology and chemistry."[63]
Processes
Until the late spring of 1937, experimentalists knew only one way for artificial radioelements to decay: by the emission from their nuclei of a positive or a negative electron. Theorists had observed, however, that a nucleus liable to produce a positron might also transmute by capturing one of the two atomic electrons—the so-called K electrons—closest to it. The heavier the nucleus, the stronger the pull on the K electrons and the greater the likelihood of capturing one of them. The possibility was first aired by that inventive interpreter of Fermi's theories, Gian Carlo Wick.[64] A means of detecting the process, should it occur, lay close to hand. An atom containing the stable nucleus created by K-electron capture would lack an electron in its innermost shell. An electron from the next shell is likely to fall into the hole and to emit an x ray, called a Ka ray, in the process. K-electron capture by an unstable nucleus of charge Z betrays itself by a Ka ray characteristic of element Z – 1. The higher the Z and the longer the life of a positron emitter, the more likely the competing K process is to occur. None of the naturally occurring radioelements, all of which have high Z , decay by releasing positrons. Hence the K-prospector looked as though it were among the longest-lived
[62] Johnston, Wolfgang, and Libby, Science, 113 (1951), 1–2.
[63] Lawrence, "Report [for 1939]," n.d. (15/18).
[64] Wick, Acc. naz. lincei, Atti, 19 (1934), 319–24 (4 Mar 1934).
emitters of positive electrons he could make as far up the periodic table as his means allowed.
Fermi's theory modelled beta decay and the competing capture process in analogy to the theory of electromagnetic radiation: just as an atomic electron can release or absorb a photon in leaving or reaching an excited state, so a proton can give rise to a positron or capture an electron while turning into a neutron. The analogy breaks down in that the energy of the beta particle created in the process is not equal to Emax , the difference in energy of the nucleus before and after the creation; rather, the energy may take any value up to Emax , as indicated in figure 8.2. To save the principle of energy conservation, physicists had supposed that a second particle is created in the beta decay, a "neutrino," whose lack of charge and vanishingly small mass (necessary to produce the asymmetry in figure 8.2) protected it from observation. With a neutrino mass of zero, the shape of the theoretical beta curve is determined chiefly by the value of Emax . Fermi's calculation, which took the strength of the interaction between electron and neutrino to be proportional to the amplitude of their fields, did not give quite the degree of asymmetry observed. Theorists at the University of Michigan, E.J. Konopinski and his professor, G.E. Uhlenbeck, came closer in the cases of P32 and Al28 by making the interaction proportional to the product of the amplitude of the electron field and the gradient of the neutrino field.[65]
In 1936 Christian Møller in Bohr's institute compared the predictions of Fermi and of Konopinski and Uhlenbeck (K-U) for the total probabilities per second of positron emission (l+ ) and K-electron capture (lK ) in a hypothetical susceptible nucleus of high Z . (l is the inverse of the period of the activity.) His result: on both theories lK is much larger than l + , the disparity being the greater the smaller Emax . Then he specialized to the curious results of Cork and Lawrence, whose "platinum" decayed with a period of 49 minutes by emission of positrons of Emax = 2.1 MeV. Neither Fermi nor K-U could give enough positrons to fit these data. Møller supposed that K-electron capture must have occur-
[65] Konopinski and Uhlenbeck, PR, 48 (1935), 7–12. Cf. Rasetti, Elements , 193–200, and (for Al ) Cork, Richardson, and Kurie, PR, 49 (15 Jan 1936), 208.
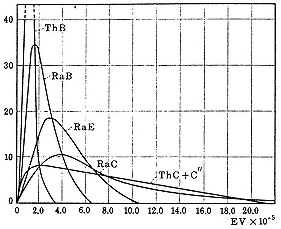
Fig. 8.2
The beta-ray spectra of some naturally occurring
radioisotopes. The ordinate indicates the percentage
of the total activity at the energy of the abscissa to
which it corresponds. Rasetti, Elements , 146.
red in the Berkeley experiments 9 times or 47 times as often as positron emission depending on whether events followed Fermi or K-U. He advised looking for x rays from the "iridium" formed from the "platinum" decay. Lawrence could not find the x rays and, as usual, thought he had caught out the theorists: "It looks to be a serious difficulty for the Fermi theory." Since Bohr's institute had no machine for making radioplatinum, experimentalists followed up Møller's lead by seeking Ka from the decay product of the heaviest available positron emitter. This was Sc43 (Z = 21, t = 4 hours), made by alpha particles from radon on (ancestor) calcium via (a ,p). A search for Ka from (descendent) calcium by J.C. Jacobsen failed. Calculations indicated that for Sc43 , lK /l+ = 5 according to K-U and 0.1 according to Fermi. Calculation and measurement in Copenhagen therefore favored Fermi.[66]
Berkeley had by then plumped for K-U on the basis of its apparently better fit to measurements of beta decay. Here the primary instrument of research was Kurie's cloud chamber and the
[66] Møller, PR, 51 (1937), 84–5, rec'd 9 Nov 1936; Cork and Lawrence, PR, 49 (1936), 788–92; Jacobsen, Nature, 139 (1937), 879–80, letter of 6 Apr; Lawrence to Segrè, 5 Apr 1937 (16/14).
primary researchers himself, J.R. Richardson, and Hugh Paxton. By using hydrogen, in which slow particles have a better chance to show their presence than in oxygen, then the usual medium in the chamber, they obtained close agreement with K-U for N13 , F17 , Na24 , and P32 . The cloud chamber men concluded that "[the K-U theory] completely describes the process of emission of a beta particle."[67]
As they drifted further along the periodic table, however, their conviction dissipated. Active chlorine, argon, and potassium could not be fitted to K-U unless each contained two unresolved activities. And nothing fit unless Emax were put higher than the limit to which, as judged by the eye, the experimental curve tended.[68] (They could not measure all the way to the maximum because they could not register enough of the very few fastest particles.) Ernest Lyman, another graduate student associated with the Kurie group, confirmed the disconfirming of K-U in the cases of P32 and RaE. As he observed, however, P32 and RaE have unusually long periods (14 and 5 days respectively), and might die in ways not dreamed of in the competing theories.[69]
Fermi himself had pointed out that substances like RaE might escape his theory. He called attention to their position on the so-called Sargent curves, a plot of logEmax against logl for the naturally radioactive substances. Its author, B.W. Sargent, who took up the project while at the Cavendish, divided the empirical points into two classes, each of which fell roughly along a straight line (fig. 8.3). For a given Emax , an element of class II, which included RaE, has a much longer life than an element of class I. Their decay apparently required an inhibiting change of nuclear spin. At a meeting of the American Physical Society in Seattle in June
[67] Kurie, Richardson, and Paxton, PR, 48 (1935), 167, and PR, 49 (1936), 368–81, rec'd 7 Jan 1936, 372, quote. Konopinski had worried about N and the difficulty of putting the theories to the test; Konopinski to Bethe, 10 May 1935 (HAB, 3).
[68] Kurie, Richardson, and Paxton, PR, 49 (15 June 1936), 203 (APS meeting, 20–1 Dec 1935); Newson, PR, 51 (1937), 624–7, rec'd 16 Feb.
[69] Lyman, PR, 50 (1936), 385, and PR, 51 (1 Jan 1937), 1–7, rec'd 27 Oct 1936. By 1939, experiment favored a mixture of Fermi and K-U (Walke, Rep. prog. phys., 6 (1939), 20–1), and theory gave little support to either (Breit, RSI, 9 (1938), 64).
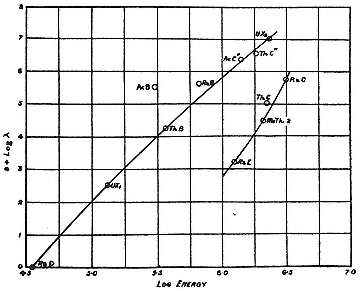
Fig. 8.3
The Sargent curves relating the maximum energy of the electrons
emitted by natural radioelements to the decay periods. Sargent,
PRS, A139 (1933), 671.
1936, Lawrence introduced Laslett to talk about a singularly long-lived positron emitter, Na22 (t = 3 years), first made by Otto Frisch by (g ,n) on F19 , then in comparative plenty by Laslett by (d,a ) on Mg24 . Its exceeding longevity interested Willis Lamb, who was brought forth at the same meeting by his mentor, Oppenheimer. Lamb reported that his calculations showed that if Na22 did require a change in nuclear spin, it would be twice as likely to decay by K-electron capture as by positron emission according to Fermi's theory, and thirty times as likely according to K-U. He proposed as a test not looking for the Ka line of neon but counting the relative numbers of alpha particles (one for each atom of Na22 created) and positrons (one for each positron decay) in the process. K-electron capture was not, and has not been, observed in Na22 .[70]
[70] Sargent, PRS, A139 (1933), 671, and in Shea, Otto Hahn , 227–9; Frisch, Nature, 136 (1935), 220; Lamb, PR, 50 (1936), 388–9; Laslett, ibid., 388, and PR, 52 (1937), 529–30, rec'd 25 June, and Laslett to Lawrence, 26 June 1936 (10/32).
While Laslett wrote up his results, Alvarez entered the game and picked up the chips. He noticed that Sc43 lies on the first Sargent curve and looked around for a neighboring element with a radioisotope on the second. The cyclotroneers had been exploring the region. By the end of 1935 they had reached zinc and had studied at least one element, argon, carefully; but they did not stop to examine the transition elements below zinc closely enough to find the eligible positron emitters they had activated in them.[71] Nor did Livingood's surveys of 1936 or a direct search among the activities of copper pick them up.[72]
Harold Walke, who was so unsure of himself that he had to be careful, then looked closely at the activities of the first few elements in the fourth period. He found a strong positron activity induced on titanium; chemical separation pointed to an isotope of vanadium (later identified as V48 ) produced by (d,n). The period of decay, 16 days, placed the new activity on the second Sargent curve. That was the combination desired: a Z high enough, a life long enough. Alvarez attacked radiovanadium with McMillan close behind, opening loopholes, "following the job." Alvarez did not attend the Laboratory picnic on Sunday, June 20. That day he detected rays from the decaying vanadium with a penetrating power appropriate to the K rays of titanium. K-electron capture, long expected in theory, thus materialized in the laboratory. Alvarez made the ratio lK /l+ about 1, closer to Fermi's theory than to K-U. Lawrence praised the work as "especially significant."[73]
[71] Livingood, PR, 49 (15 Jan 1936), 206 (Zn); Thornton, ibid., 207 (As, Ni, Co); Snell, ibid., 207, and PR, 49 (15 Apr 1936), 555–60 (A).
[72] Livingood, PR, 50 (1 Sep 1936), 425–32; Lawrence to Segrè, 5 Apr 1937 (16/14). Alvarez, PR, 54 (1 Oct 1938), 486, miscredits Yukawa and Sakata, Phys.-Math. Soc. Japan, Proc., 17 (1935), 467–79, and 18 (1936), 128–30, rather than Wick with the first suggestion that K-electron capture might compete with positron emission. Cf. Rasetti, Elements (1936), 202–3, and Segrè in Trower, Discovering Alvarez , 11–2.
[73] Walke, PR, 51 (1937), 1011, talk at APS, Washington, ca. 1 May; Alvarez, PR, 52 (1937), 134–5, letter of 21 June; Walke, PR, 51 (1937), 439, Hurst and Walke, ibid., 1033, and Walke, PR, 52 (1937), 663, letter of 6 Aug (K, Ca); Allen and Alvarez, RSI, 6 (1935), 329; Cooksey to Lawrence, 17 and 21 June 1937, and Lawrence to Cooksey, 25 June 1937 (4/21).
Once the process had been seen everyone saw it. Livingood, now disciplined in collaboration with Seaborg and another chemist, Fred Fairbrother, a Leverhulme Fellow from the University of Manchester, found a positron activity in manganese. Later Livingood and Seaborg showed that this isotope (or rather isotopes: Mn52 and Mn54 ) decays by K-electron capture and that Zn65 , which Livingood had examined earlier, does so too. Then Otto Oldenburg, on sabbatical from Harvard, found a K process without positron competition in tantalum excited by neutrons (Ta180 , t = 8.2 hours).[74] In all this there was a difficulty, however, which McMillan pressed on Alvarez. Perhaps the K electron does not jump into the nucleus but out into the world, driven by a gamma ray originating from an excited state of the stable final nucleus? If the probability for "internal conversion" (the release of an atomic electron that absorbs the gamma ray) were sufficiently high, only the x rays and the converted electrons would appear in the radiations. To decide the question, the experimenter must determine the element from which the K ray emerges: if from element Z (Z being the atomic number of the radioelement), then internal conversion; if from element Z – 1, then K-electron capture.
Alvarez took up this problem with the positron emitter Ga67 , which Wilfred Mann had made by (d,n) on zinc. The radiation from Ga67 consists of electrons, gamma rays, and x rays characteristic of zinc. Ernest Lyman and another graduate student showed that all the electrons had about the same energy. Alvarez explained: a nucleus of Ga67 swallows a K electron and ends in an excited state of Zn67 , which emits a monoenergetic gamma ray that has a moderate possibility of internal conversion; homogeneous electrons demonstrate the conversion and the zinc x rays the filling of the holes in the zinc atom's electronic structure. Walke found a better demonstration with long-lived V49 (t = 600 days), which decays only by K-electron capture and only into the ground state of titanium. No gamma rays or ionizing radiations
[74] Livingood, Fairbrother, and Seaborg, PR, 52 (1937), 135, letter of 30 June; Livingood and Seaborg, PR, 54 (1938), 239, 391; Seaborg, Jl., 1 , 355–6 (23 June 1938); Oldenburg, PR, 53 (1938), 35–9, rec'd 22 Oct 1937.
complicate the picture. Like the V48 in which Alvarez had made his discovery, V49 appeared to die out more closely to Fermi's than to K-U's specifications. By 1939 K-electron capture had been recognized in some twenty isotopes, including two of element 43.[75] K-electron capture proved to be as common as theorists expected. Among other consequences of its ubiquity, it ruled out the possibility that platinum could be the source of the positrons seen by Cork and Lawrence.[76]
Some pieces of the platinum puzzle fit well with the study of another nuclear process, in which, like the detection of K-electron capture, the Laboratory pioneered. This was the behavior of isomers, forms of the same unstable nucleus differing in internal energy. A pair of isomers can decay in several ways: each might emit a beta particle, or the more energetic isomer may relax into the lower by throwing off a gamma ray, or both processes might occur together. Isomerism first came to light in 1921, when Otto Hahn deduced that the third member of the radioactive chain descending from uranium UX2 (Pa234 ) consists of two beta emitters, both of which, he thought, arose directly from UX1 (Th234 ). No other instance was found. It took theorists some time to devise an explanation. In 1934 the inventive Gamow thought to trace the difference between Hahn's isomers UX2 and UZ to the presence in one of them of a hypothetical antiproton-proton pair in place of two neutrons. Another idea, put forward by a student of Heisenberg's, C.F. von Weizsäcker, in 1936, preserved the upper isomer long enough to emit a beta ray by supposing that a big difference in spin discouraged it from dropping immediately to the lower. Still, the matter was neither clear nor persuasive; Hahn's partner Lise Meitner expressed skepticism about isomerism and Bethe, though accepting the phenomenon, hedged over whether the pair UX2 and UZ was an example of it. The Cavendish's Norman Feather and Egon Bretscher cleared the matter up early
[75] Oldenburg, PR, 53 (1938), 35–9, rec'd 22 Oct 1937; Alvarez, PR, 53 (1938), 606, letter of 15 Mar, and PR, 54 (1 Oct 1938), 486–97, followed up by Helmholz, PR, 57 (1940), 248; Walke, Williams, and Evans, PRS, A171 (1939), 360; Walke, Rep. prog. phys., 6 (1939), 22–3. The vanadium isotopes were misidentified as V and V .
[76] Lawrence recognized the problem; Lawrence to Bohr, 8 Jul 1937 (3/3).
in 1938. They made UX2 the excited metastable state and the only direct descendent of UX1 , and UZ the rare result of UX2 nuclei that could not restrain their gamma radiation. By an appropriate attribution of the complex beta rays, they showed that UZ belonged on the first, and UX2 on the second Sargent curve.[77]
Feather and Bretscher had the encouragement of the first unequivocal example of isomerism among artificially active elements. That was the work of Arthur Snell, the most complete and exact bit of radiochemistry accomplished at the Laboratory to that time (August 1937). He was inspired by the apparent existence of too many active bromines. Fermi's group had found two, with periods of 18 minutes and 4.5 hours, which they supposed to arise by slow neutron capture in the two stable bromine isotopes, Br79 and Br81 . Then a Soviet physicist, I.V. Kurchatov, found a third activity (t = 36 hr) in bromine hit by neutrons from a Rn-Be source, for which there was no obvious available antecedent in natural bromine. Kurchatov proposed that whereas Fermi reactions were of the ordinary type (n,g ), his occurred via the then still unestablished route (n,2n), giving rise to a suppositious active Br78 .[78]
Snell checked these results by trying to make the Italian radioisotopes Br80 and Br82 and the Soviet Br78 in other ways than by neutron bombardment of natural bromine. He examined no fewer than twenty-eight different reactions involving As, Se, Br, Kr, and Rb activated by deuterons, alpha particles, and neutrons. Among his most significant results: Br78 does exist—he made it by (a ,n) on the single arsenic isotope As75 and by (d,n) on Se77 —but its period (6 min) and its decay mode (positron emission) exculpated it from responsibility for Kurchatov's activity; Br83 , hitherto
[77] Gamow, PR, 45 (1934), 728–9; von Weizsäcker, Nwn, 24 (1936), 813–4; Meitner, in Bretscher, Kernphysik , 41; Bethe, RMP, 9 (1937), 225–6; Feather and Bretscher, PRS, A165 (1932), 530–1, 545–50. Cf. Flammersfeld in Frisch, Trends , 71–6.
[78] Amaldi et al., PRS, A149 (1935), 522–58, and Amaldi, Phys. rep., 111 (1984), 128ff.; Kurchatov et al., CR, 200 (1935), 1201–3; Livingston and Bethe, RMP, 9 (1937), 348–50. According to Golovin, Kurchatov , 25–6, Kurchatov proposed the existence of bromine isomers to the Soviet Academy of Sciences in March 1936.
entirely unknown, also exists (t = 2.5 hr); and the three previously known activities had to be shared among Br80 and Br82 . From his inventory of twenty-eight reactions, Snell could show that both Fermi activities belong to Br80 . And, to complete his happiness, he created Kurchatov's activity (Br82 ) by deuterons on selenium. With the advice of Bohr—this result dates back to April 1937 or earlier—Snell pinned the reaction on Se82 , since Se81 , which could have given rise to Br82 by the familiar (d,n) reaction, does not exist naturally. On this explanation, Snell gave the first example of a (d,2n) reaction.[79] The excitement of the discoverers of such arcana may be hard for outsiders to share. But in the breast of the nuclear physicist, they inspired "great joy."[80]
The joy came also to McMillan, Kamen, and Ruben, who were still laboring on Lawrence's dirty platinum when Snell completed his work. Platinum appeared to have three periods, all activated by slow neutrons and decaying by fast electrons, and gold and iridium behaved similarly. It seemed too much of a good thing. "The results of this work so far [McMillan's group wrote] do not seem to be capable of any simple explanation without the introduction of a fantastic number of isomeric nuclei."[81] Candidate isomeric nuclei turned up everywhere after the summer of 1937, and frequently for the first time in Berkeley; of the seventeen pairs of artificial isomers established by 1939, eleven were discovered or first confirmed by members of the Laboratory.[82]
Livingood and Seaborg were the most successful hunters (plate 8.2). Earlier investigators had not carried their separations of zinc activated by neutrons very far. Seaborg went further. He and
[79] Snell, PR, 52 (1937), 1007–22, sent 3 Aug; Livingston and Bethe, RMP, 9 (1937), 326, are agnostic about (d,2n); Livingood and Seaborg, RMP, 12 (1940), 38, accept it. Cf. Segrè and Helmholz, RMP, 21 (1949), 271–2, 291.
[80] Walke, as quoted in Cooksey to Lawrence, 17 June 1937 (4/21); cf. Seaborg, Jl., 1 , 296 (26 Nov 1937), on the excellence of Snell's work. The original three bromine activities were confirmed by Bothe and Gentner, Nwn, 25 (1937), 284, letter of 19 Apr, by photoexcitation.
[81] McMillan, Kamen, and Ruben, PR, 52 (1937), 375–7, rec'd 19 Jul.
[82] Walke, Rep. prog. phys., 6 (1939), 26, and Walke, Williams, and Evans, PRS, A171 (1939), 360–82. Some thirty-four isomeric pairs were known in 1940, and over seventy-five pairs by 1949. Frisch, Ann. rep. prog. chem., 36 (1940), 16–21; Segrè and Helmholz, RMP, 21 (1949), 255–9.
Livingood in consequence added two new nickels to their treasury of isotopes, cleaned up earlier misattributions by Livingood and by Robert Thornton, and identified isomers of Zn69 . In all there were but three zincs, which Livingood and Seaborg prepared in a total of eleven different ways. Their attributions have stood.[83] Then there were iron and its neighbors. With separated fractions from fifteen bombardments of iron, four of chromium, and two of manganese, all by deuterons, and several irradiations of the same with alpha particles and neutrons, Livingood and Seaborg straightened out a great many reactions and uncovered a pair of isomers in Mn52 .[84] The most interesting and complex of the isomers came to light during a lengthy study of activated tellurium, which attracted Livingood and Seaborg not only as another radiochemical puzzle, but also as a possible quarry for a useful radioactive iodine.
Their first irradiation of tellurium with deuterons took place on March 26, 1938. They moved on to iodine, then back to tellurium, glimpsing, losing, and finally establishing the existence of an iodine with the biologically useful period of 8 days. That brought them through the summer. In September Livingood left for Harvard; he set up an electroscope in his kitchen to examine samples mailed him by the indefatigable Seaborg.[85] There were too many telluriums. Joseph Kennedy, Seaborg's graduate student, helped to untangle them. He soon confirmed his collaborators' conjecture that the 8-day iodine, I131 , descended from not one but two telluriums, isomers of Te131 with periods of 1 hour and 1.2 days. But on further examination, the hour period shrank to 45 minutes and then split into two, of 30 and 55 minutes; and, in addition to the three telluriums that had replaced Kennedy's two, there were three more, of periods of 10 hours, 1 month, and several months. After
[83] Livingood and Seaborg, PR, 53 (1938), 765, and PR, 55 (1939), 457–63, rec'd 15 Dec 1938; Thornton, PR, 53 (1938), 326, and Livingood, PR, 50 (1936), 425; Seaborg, Jl., 1 , 412, 416 (3 and 15 Dec 1938). Alvarez, PR, 53 (1938), 606, and Mann, PR, 54 (1938), 649–52, also helped to purge Thornton's "zincs."
[84] Livingood and Seaborg, PR, 54 (1938), 51–5, rec'd 10 May, and PR, 54 (1938), 391, rec'd 11 Jul; Seaborg, Jl., 1 , 360–1 (3 Jul 1938).
[85] Seaborg, Jl., 1 , 333, 339–43, 347–8, 353, 359–61, 367, 373, 377–8 (26 Mar–6 Sep 1938); Livingood and Seaborg, PR, 54 (1938), 775–82, rec'd 7 Sep 1938, which itemizes five radioiodines, three of which were new.
three weeks of hard work in January 1939, Kennedy and Seaborg proved that the 30-minute (corrected to 25-minute) activity was a parent of I131 and the lower of a pair of isomers whose upper level was the activity of 1.2 days.[86]
To shorten a story already sufficiently long, Seaborg, Livingood, and Kennedy labored on the tellurium system until the end of December 1939, when they declared on the basis of sixteen different reconfirmed reactions that there exist precisely four radiotelluriums, three of which come in two isomers each. With a clever chemical technique, soon to be described, they showed which isomeric state was the lower; and, with the help of two graduate students, Carl Helmholz and David Kalbfell, who examined the specimens in a beta-ray spectrograph, they identified conversion electrons knocked out by the gamma rays emitted in transitions from upper to lower isomeric states.[87]
After Livingood, Seaborg took up with an isomer hunter of even greater resourcefulness, Segrè, who brought the experience of identifying isomeric Cu65 in cyclotron scrap.[88] Segrè required something in addition to Seaborg before he would begin their planned search for isomers of element 43: a better detector than Livingood and the Laboratory's other hunters of new activities employed. This detector consisted of an ionization chamber of a type used in Rome connected to a dc amplifier built by Lee DuBridge during a summer at Berkeley.[89] Segrè's electrometer (plate 8.3) later served in the detection of H3 , C14 , and plutonium. It began by registering a beta ray, no gamma ray, and an x ray from activated molybdenum, which Seaborg and Segrè diagnosed as an electron converted from a gamma ray with almost 100 percent efficiency and the associated K radiation of element 43. They sent a letter to the Physical Review announcing these results; but
[86] Seaborg, Jl., 1 , 364, 394, 403, 406–7, 424, 427–30, 432–6, 439 (28 Jul, 11 Oct, and 8 Nov 1938, 11–30 Jan and 2 Feb 1939); Seaborg and Kennedy, PR, 55 (1939), 410, letter of 31 Jan.
[87] Seaborg, Livingood, and Kennedy, PR, 55 (1939), 794, letter of 31 Mar.
[88] Seaborg, Jl., 1 , 361 (9 Jul 1938); Perrier, Santangelo, and Segrè, PR, 53 (1938), 104–5. Cf. Segrè in Nicolini et al., Technetium , 8.
[89] Seaborg, Jl., 1 , 361–4 (9–18 Jul 1938); Segrè to Lawrence, 7 Jan 1938, and reply, 7 Feb 1938 (16/14). The standard armamentarium of detectors in 1940 is described in Seaborg, Chem. rev., 27 (1940), 207–10.
their pleasure suffered an interruption when Lawrence told them that Oppenheimer thought so high a degree of conversion impossible. Lawrence asked them to withdraw their report. The frequency with which the Laboratory had had to retract published results had declined since Lawrence had gone full time into fundraising and administration, and he did not wish to risk a throwback. Seaborg and "a rather agitated Segrè," who had lately been the master of his own ship, complied. Ten days after this contretemps, the Physical Review published a letter from Bruno Pontecorvo describing a similarly high conversion in rhodium. Lawrence conceded that Segrè and Seaborg should resubmit.[90]
They soon confirmed their observations. They asked a graduate student, Philip Abelson, who had built a good spectrograph, to determine whether the x radiation they had found belonged to element 43 (it did), and they established that the lower isomer associated with the 6-hour activity had a life of at least forty years. These are the isomers of Tc99 (the lower has a life of almost a million years), the clinically important species of technetium.[91] The collaboration was close and demanding. Segrè participated in the chemical separations and Seaborg in the physical measurements, and they wrote up their results together.[92]
Since atoms of isomeric nuclei possess precisely the same chemical properties, it might not seem advisable to think about ways to separate isomers chemically. Segrè thought it could be done, however, by modifying the Szilard-Chalmers process, in which nuclei rendered active by an (n,g ) process (e.g., radioiodine) and knocked out of a compound (e.g., ethyl iodide) by the neutrons they absorb, are collected by combining them with suitable molecules (e.g., in a precipitate of silver iodide). His thought was at first received unenthusiastically because the recoil from the isomeric
[90] Seaborg, Jl., 1 , 367–8, 376, 381, 383, 390, 393, 396 (26 Jul, 1 and 30 Aug, 14 and 20 Sep, 8 and 14 Oct 1938); Segrè and Seaborg, PR, 54 (1938), 772, letter of 14 Oct (originally 14 Sep); Kalbfell, ibid., 543, determined the energy of the conversion electron.
[91] Lawrence to Sproul, 31 Dec 1938 (16/14); Seaborg, Jl., 1 , 399, 405, 412–5 (23 Oct, 12–14 Nov, 3–10 Dec 1938); Seaborg and Segrè, PR, 55 (1939), 808–14; Segrè, Ann. rev. nucl. sci., 31 (1981), 9.
[92] Seaborg, Jl., 1 , 366ff., 418 (20 Dec 1938), 424 (10 Jan 1939), 437–8 (1 Feb 1939).
transition seemed insufficient to free an atom from its chemical bonds. Ralph Halford, an instructor in the Chemistry Department, with whom Seaborg discussed the matter, thought that he might be able to effect a chemical separation. Segrè and Seaborg irradiated a liter of ethyl bromide, which, after treatment by Halford, gave a hydrobromic acid enriched in the lower isomer of Br80 . This isomer thus stood revealed as the 18-minute activity observed by Fermi's group, by Kurchatov, and by Snell.[93] Seaborg and Kennedy immediately applied the scheme successfully to tellurium, confirming the double origin of 8-day iodine; and then, together with Segrè, tried hard, but with few positive results, to separate isomers of several other metals, from manganese to platinum.[94]
The Groaning Board
The periodic table of the elements began as a sort of Ouija board, arranged on arbitrary principles and operating with mysterious powers. The concept of natural isotopes and the theory of the nuclear atom diminished the mystery by explaining that the principle of arrangement by weight was a happy approximation to the true regulation by atomic number. Why the natural isotopes have the weights they do remained a mystery. The discovery of means to split atoms and make natural isotopes radioactive made possible determination of the exact binding energies of nuclei and an understanding, or the beginning of one, of the genetic interrelationships among the elements.
The Laboratory made very material contributions to the new knowledge. The cyclotron plugged two holes in the periodic table, the spaces at 43 (technetium) and 85 "eka-iodine," later astatine. The first, we know, was found by Segrè and Perrier in
[93] Szilard and Chalmers, Nature, 134 (1934), 462 in Szilard, CW, 1 143–4; Szilard to Hopwood, 28 Aug 1934 (Sz P, 17/197); Seaborg, Jl., 1 , 422–3 (4–7 Jan 1939); Segrè, Halford, and Seaborg, PR, 55 (1939), 321–2, letter of 13 Jan. De-Vault and Libby, ibid., 322, followed up Segrè's suggestion with different chemistry; Seaborg had long been interested in ways to separate radioelements without recourse to carriers, e.g., Grahame and Seaborg, PR, 54 (1938), 240–1, and Seaborg and Livingood, JACS, 60 (1938), 1784–6.
[94] Seaborg and Kennedy, PR, 55 (1939), 410, letter of 31 Jan; Seaborg, Jl., 1 , 465–6, 469–82, 478–84 (24 Mar–5 May 1939).
molybdenum irradiated by deuterons in the 27-inch; the second, found in an experiment that Segrè proposed, came from bombardment of bismuth by alpha particles in the 60-inch. At Hamilton's bizarre suggestion, astatine was fed to guinea pigs, who obligingly concentrated it in their thyroids to demonstrate its similarity to iodine; the Laboratory was nothing if not interdisciplinary.[95]
The total record appears in figure 8.4. It indicates the percentage of reactions known in May 1935, July 1937, and December 1939 that the Laboratory either discovered or helped to clarify. The comparison with Cambridge is particularly striking: in 1935 Berkeley matched the Cavendish only in reactions initiated by deuterons; by 1937 the Laboratory dominated study of deuteron interactions and was extending itself vigorously into neutron and proton work; by 1939 it had risen to dominance in alpha- as well as deuteron-induced reactions, and to hegemony in neutron work, while Cambridge, under the management of W.L. Bragg, had lost its position in nuclear chemistry. Other laboratories, for example, Fermi's in Rome, Bothe and Gentner's in Heidelberg, and DuBridge's at Rochester, specialized in one sort of reaction only.[96] A cruder, though perhaps more impressive index to Berkeley's contribution appears from comparison of the percentage of all reactions known at a given time for which Berkeley was credited or co-credited: in 1935, one reaction in ten; in 1937, one reaction in four; in 1939, almost one reaction in two.[97]
[95] Corson and Mackenzie, PR, 57 (1940), 250; Corson, Mackenzie, and Segrè, PR, 57 (1940), 459, letter of 16 Feb, and PR, 58 (1940), 672–8, rec'd 16 Jul; Hamilton, Jl. appl. phys., 12 (1941), 454–5; Cornog in Trower, Discovering Alvarez , 26.
[96] These generalizations can be documented in other reviews than those used for table 6.2, e.g., Grégoire, Jl. phys. rad., 9 (1938), 419–27, and the running score in Diebner and Grassmann, Phys. Zs., 37 (1936), 359–83, 38 (1937), 406–25, 39 (1938), 469–501, 40 (1939), 297–314, and 41 (1940), 157–94.
[97] Birge, in U.C., 1939 prize , 23, credits the Laboratory with the discovery of over one half of all isotopes (223, according to him) "discovered" by cyclotrons.
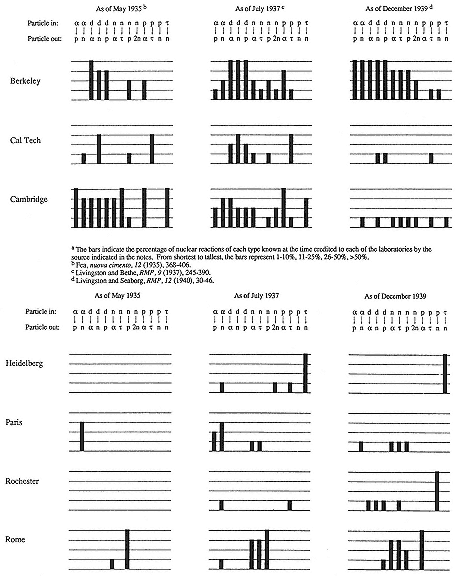
Fig. 8.4
The Laboratory's contribution to the inventory of artificial radioactive substances
during the 1930s.