Composite Cones with Possible Geothermal Potential—As Yet Unproven by Deep Drilling
Mount Shasta
Mount Shasta, one of the southernmost of the Cascade volcanoes, is located in northern California. With a volume of 350 km3 and an elevation of 3050 m, this mature composite cone is also the largest Cascade volcano. The summit crater, two older central vents, and a line of flank vents are located along north-south trends (Christiansen et al ., 1977). Large dacitic cones and flows have erupted from both summit and flank vents. A 450-km2 area northwest of the volcano is covered by a hummocky debris avalanche deposit, which Crandell et al . (1984) interpreted as a sector collapse of the main cone ~300,000 years BP.
The cone is made up of equal portions of lava flows and blocky pyroclastic debris, including pyroclastic flow deposits and volcanic mudflows (Christiansen et al ., 1977), most of which are composed of pyroxene-andesite and hornblende-bearing andesite. Basalt is found only below an altitude of 2100 m in cinder cones and small lava flows. Mount Shasta formed during the last 500,000 years, and four periods of cone-building activity have occurred during the last 250,000 years (Christiansen, 1985).
There are summit fumaroles and areas of intense local hydrothermal alteration on each of Mount Shasta's four main cones, but nowhere else. Theoretically, there should be a small hydrothermal system within or below this large composite cone; however, there is no evidence for such a system because of the effective shield established by high flux of cold water from rain and
snow on the mountain. To date, there has been no drilling on Mount Shasta to test for a geothermal system.
Mount Hood
Mount Hood, also in Oregon, is located within a broad graben that follows the summit of the north-south-trending topographic high, which is the backbone of the high Cascades of the Pacific Northwest. Mount Hood was an obvious target for geothermal exploration and research, and a cooperative effort was begun in 1976 by a consortium of federal and state agencies (Williams et al ., 1982).
Mount Hood is a 2200-m-high mature composite cone with an approximate volume of 188 km3 . Eruptions have occurred there over the past 700,000 years; the most recent was less than 200 years ago (Wise, 1969; Crandell and Meyer, 1977). Mount Hood is composed of interbedded thin lava flows and pyroclastic debris (ashfall deposits, ignimbrites, and laharic breccias). Little is known or has been inferred about intrusive rocks in Mount Hood (shown in cross section in Fig. 7.24) except for the "plug" dome that comprises andesitic and hornblende andesitic lava; peripheral cones are composed of basalt.
The only surface manifestations of a hydrothermal system on Mount Hood are summit fumaroles, at temperatures of 50 to 85°C, and areas of hydrothermal alteration surrounding the plug dome. Although 25 shallow wells have been drilled on the flanks of Mount Hood, no shallow magma chamber or large hydrothermal systems were detected; the shallow wells did not penetrate the near-surface outflow zone of cold groundwater.
The deepest geothermal gradient hole drilled at Mount Hood is located on the lower flanks of the volcano near faults in basalt flows that predate Mount Hood; the bottomhole temperature of this well is 120°C at a depth of 1.8 km (Priest, 1982). The thermal gradient of ~60°C/km in this corehole could be related to magmas in the cone, but it also can be explained solely by the high heat flow in this tectonically active area.
Because of the enormous terrain corrections required for analysis, and the presence of a cold groundwater shield below the slopes of Mount Hood, most traditional geophysical
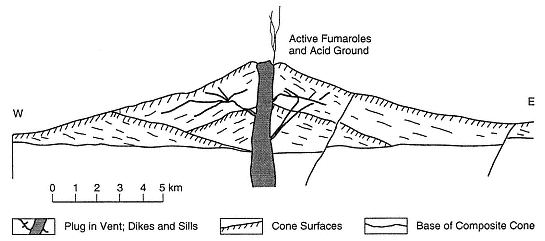
Fig. 7.24
Composite cross section of Mount Hood in Oregon.
(Based on work by Wise, 1968; Williams et al. , 1982; Priest, 1982.)
exploration methods did not reveal the presence or absence of a hydrothermal system. Shallow drilling penetrated some zones of warm water, but most drillholes never reached beyond the cold, near-surface groundwater. There may be a hydrothermal system below this large, young composite cone, but it has not yet been observed.
Mount Adams
Located east of the main Cascade trend, Mount Adams in Washington is a large (~200-km3 ) composite cone with an elevation of 3743 m. The composite cone is near the center of a basalt-to-rhyodacite volcanic field with more than 60 vents of Quaternary age. Flank vents, which occur at elevations of 2000 to 2500 m, are composed of mafic andesitic to dacitic lava flows and block-and-ash flows (Hildreth and Fierstein, 1985). Peripheral basaltic cinder cones and lava flows erupted on the lower flanks have a volume of 70 km3 . Volcanic activity began about 500,000 years ago, and the youngest eruptions took place 3500 years ago; most of the composite cone was constructed between 20,000 and 10,000 years ago.
There are summit fumaroles and warm springs near the base of the volcano. The breccia and scoria core of this cone has been severely altered by acid waters. The 4-km2 area of altered core is a source of avalanches and debris flows because of the gravitational instability of the clay alteration products (Hildreth and Fierstein, 1985).
On the basis of the petrology of erupted materials, Hildreth and Fierstein concluded that it is unlikely there is any significant magma reservoir within Mount Adams. High precipitation (3500 mm/year) makes the mountain an important recharge site and the extremely permeable carapace is saturated with cold water that does not remain long enough to be heated.
Mount Fuji
One of the Earth's most famous composite cones, Japan's Mount Fuji is composed of a group of 3 overlapping cones and 100 peripheral cones. Most of these vents are located along a north-northwest-south-southeast-trending line that is parallel to regional structures (Kuno, 1962; Tsuya et al ., 1981). The 1.5- × 0.7-km summit crater is 750 m deep. Older lavas at Fuji are olivine basalts, whereas the younger cones are composed of andesite and peripheral cones of olivine basalt. The cones comprise interbedded ashfall deposits, lahar deposits, ignimbrites, and lava flows. The complex is ~80,000 years old, and the most recent activity occurred in 1707 AD (Kuno, 1962).
All thermal anomalies on Fuji are masked by movement of shallow groundwater. Yuhara (1974) reported that each year 77% of the precipitation flows out at the foot of the cone and another ~20% is lost by evaporation. Tritium analyses have revealed that water emerging near the base is not old. Shallow aquifers follow scoria-lapilli beds and fractures in lava flows. Impermeable units are mudflow deposits and older basement rocks. Very little of the recharge water enters the volcano's interior to be heated. Mount Fuji has not been drilled for geothermal resource evaluation.
From Yuhara's (1974) observations at Fuji and Mount Yotei (also in Japan), it was determined that recharge into the hydrothermal system of a composite cone may not be adequate to maintain a hydrothermal system. Conditions necessary for adequate recharge will most likely depend upon the structural framework of the individual volcano, its maturity, fault patterns, and the degree of intrusion and hydraulic fracturing associated with dike-sill systems and small plutons. As described earlier, eroded, mature volcanoes in areas with thick continental crust expose large volumes of hydrothermally altered lava and pyroclastic rock.