9.5—
The Genes for Ribosomal-RNA
More is known about the properties, organization and function of the genes for rRNA than any other part of the genome. These DNA segments direct the synthesis of rRNA molecules which form the structural 'backbone' of ribosomes, without which cells cannot function. It has been known for some time that they are associated with the nucleolus because certain mutants of the toad Xenopus, which lack nucleoli, are incapable of synthesizing rRNA. During development these mutants die because they are unable to synthezise ribosomes.
9.5.1—
DNA-RNA Hybridization
The genetic origin of any RNA molecule can be studied by DNA-RNA hybridization. This technique is similar in principle to DNA-DNA reassociation. It depends upon the fact that any RNA molecule synthesized on a DNA template has a base sequence complementary to the DNA strand from which it was transcribed. Under suitable conditions, therefore, DNA can be induced to combine with complementary-RNA (cRNA) to produce an RNA-DNA double helix. This provides a means of locating and measuring the genes that code for a particular type of RNA.
One of the commonest procedures is to prepare pure DNA, denature it by heating or treatment with alkali, and to attach the single strands to a solid matrix such as a nitrocellulose filter. During subsequent treatments the immobilized DNA strands are prevented from reassociating but they are capable of forming hybrids when they are placed in a solution of nucleic acid containing complementary base sequences. Using radioactive RNA the formation of DNA-RNA hybrids can be detected by measuring the amount of radioactivity that becomes associated with the filter. The reaction is affected by a number of factors such as RNA concentration, salt concentration, temperature, the presence of compounds such as formamide, which lowers the Tm, and the reaction time. With rRNA at a concentration of 2 µg cm–3 at 70°C in 6 × S.S.C. the reaction is virtually complete within a couple of hours (Fig. 9.8). After a suitable time the filters are washed, given a mild treatment with ribonuclease to remove any unhybridized RNA, and the amount of radioactive RNA remaining is measured in a liquid scintillation counter. If the amount of DNA initially applied to the filter is known then the extent of hybridization can be calculated from the specific radioactivity of the RNA. The amount of DNA that hybridizes to rRNA can either be calculated directly from the saturation plateau (Fig. 9.8) or, more accurately, from a double reciprocal plot of the data (Scott & Ingle, 1973). In most plants between 0.05 and 1% of the DNA hybridizes to rRNA. This represents from a few hundred to several thousand genes per diploid nucleus, depending on the species (Table 9.5). The 258 and 18S rRNA molecules hybridize to separate sites on the DNA in accordance with the ratio of their molecular weights (1.3:0.7; approximately 2:1 by mass but 1:1 molar ratio).
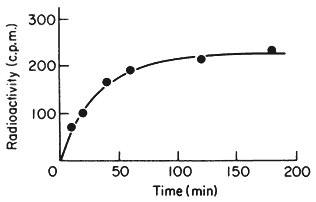
Figure 9.8
Saturation hybridization of nuclear DNA from Phaseolus
aureus with radioactive 25S rRNA. 25s rRNA (molecular weight
1.3 × 106 daltons) labelled with [3 H]-uridine at a specific activity
of 1.4 × 104 c.p.m. µg–1 was hybridized to nuclear DNA (2 µg
per filter) for increasing periods of time in 6 × S.S.C. at 70°C.
(From Grierson, 1975).
|
This indicates that there are equal numbers of genes for each type of rRNA. In cases where they do not hybridize in equimolar amounts this may be due to sequence homology between the two RNAs, or alternatively may arise by contamination of the RNA samples with other types of RNA.
Multiple genes for rRNA are a physiological necessity in order to provide sufficient sites of synthesis for rRNA molecules to meet the high metabolic demand for ribosomes. Plants often contain thousands of these genes although the number varies between varieties of the same species and between different species. Most of the available evidence suggests that all the copies are identical and this provides a clear example of repetitive DNA with a known function.
Their localization within the nucleolus has been confirmed by in situ hybridization of rRNA to cytological preparations (Brady & Clutter, 1972).
In many eukaryotes ribosomal DNA occupies a separate position in caesium chloride gradients relative to main band DNA. However, since they represent 1%, or less, of the genome these genes are not easily detected by studying the distribution of the DNA. Nevertheless, they can readily be pinpointed by hybridizing rRNA with separate fractions of DNA from the gradient. The fractionation of main band DNA from the rRNA genes of Phaseolus aureus is shown in Fig. 9.9. In general, ribosomal DNA from plants has a higher density
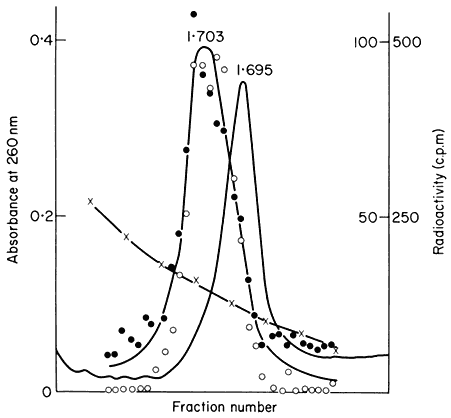
Figuer 9.9.
Separation and detection of the DNA sequences coding for
ribosomal RNA. Nuclear DNA from Phaseolus aureus was
fractionated in caesium chloride (see Figs. 9.4 and 9.5) and
the fractions from the gradient were divided into two and
hybridized to either 25s (cytoplasmic) or 23s (chloroplast)
rRNA labelled with [3 H]-uridine. Smooth, curve, absorbance
of DNA at 260 nm; solid circles, hybridization with 25s rRNA;
open circles, hybridization with 23s rRNA. The gradient of CsCl
is shown and the calculated densities of the rRNA genes and
the main band DNA are indicated. (Unpublished result of D. Grierson.)
than main band DNA. This is consistent with the fact that in higher plants rRNA has a base composition in excess of 50% GC, whereas the bulk of nuclear DNA often has a lower GC content. In this sense rRNA genes represent a type of satellite DNA. However, in different plants the rRNA genes are often present in similar numbers and with a similar density irrespective of the presence or absence of large amounts of satellite DNA. It therefore appears that ribosomal DNA and other satellites may occasionally band together in caesium chloride but this is entirely fortuitous (Ingle et al., 1975).
9.5.2—
Electron Microscopy
The rRNA genes can also be studied by electron microscopy of preparations from isolated nucleoli. This approach provides a clear indication of their organization. Figure 9.10 shows an electron micrograph of the partially
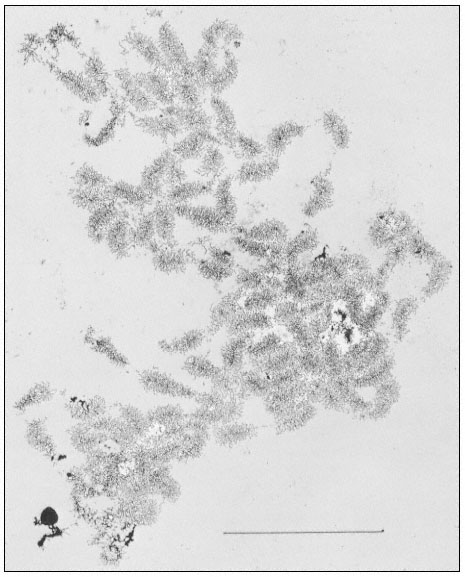
Figure 9.10
Spread contents of a complete nucleolar aggregate subunit from Acetabularia
mediterranea. Note the numerous 'matrix' units. The scale indicates 5 µm.
(From Spring et al., 1974.)
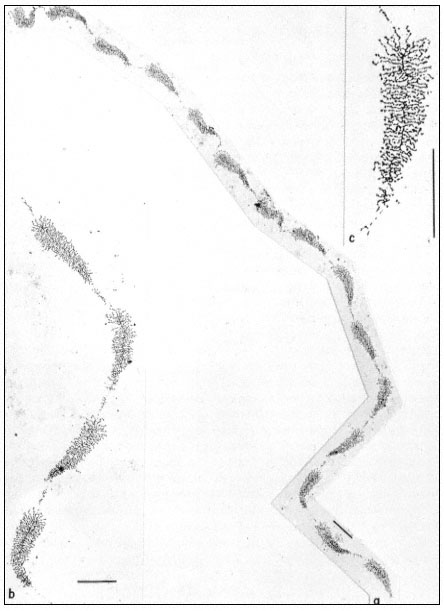
Figure 9.11
(a) Well spread nucleolar material showing the regular pattern of 'matrix'
units and 'spacer' segments. Note the length gradient of lateral fibrils within
each matrix unit. (b) Note occasional groups of small fibrils associated with the
spacer units. (c) At higher magnification, the dense packing of lateral fibrils within
the matrix unit is clearly seen. Scales indicate 1 µm, (From Trendelenburg et al., 1974.)
dispersed contents of a subunit from the nucleolar aggregate of Acetabularia. This shows a regular arrangement of fibril-covered 'matrix' units and fibril-free 'spacer' regions arranged along a central axis. A higher magnification of a 'matrix' unit is shown in Fig. 9.11. The central axis is the double-stranded ribosomal DNA itself. The 'matrix' units represent ribosomal genes which are being transcribed into RNA at a number of points simultaneously. The fibrils of the 'matrix' unit are growing chains of rRNA precursor molecules (see the section on RNA synthesis). RNA polymerase enzymes can be seen clustered on the DNA. About 120 enzymes are engaged in the simultaneous transcription of the DNA in each 'matrix' unit. The marked polarity reflects the fact the growing chains of attached RNA molecules increase in length as the polymerase enzymes responsible for their synthesis proceed along the DNA axis. In addition there is a tendency for the free ends of the RNA chains to form terminal knobs as they become folded, probably in association with proteins.
Multiple rRNA genes therefore alternate in a precise arrangement with non-transcribed regions of the DNA. Hybridization evidence shows that separate 'cistrons' for the 25s and 18s rRNA are present in the DNA, but there is no evidence for the existence of separate transcription units. In contrast, in Acetabularia each transcribed region of double-stranded DNA is approximately 2 µm long. This indicates that it codes for an RNA molecule with a molecular weight of approximately 2 × 106 daltons. This is the combined mass of the 25s and 18s rRNA molecules (1.3 + 0.7 × 106 daltons). There is clear evidence (discussed in the section on RNA synthesis) that ribosomal RNA is first transcribed as a polycistronic precursor molecule. In many plants this precursor has a molecular weight of approximately 2.4 × 106 daltons, and contains one sequence of 25s RNA plus one sequence of 18s RNA together with some extra sequences which are subsequently removed. In order to reconcile such a mode of synthesis with studies on the organization of the rRNA genes it is necessary to propose that each 'matrix' unit consists of an 18s and 25s sequence and some transcribed 'spacer' DNA. Fortunately, in cases where the organization of ribosomal DNA has been studied in detail, for example in Xenopus, evidence for precisely this type of arrangement has been obtained (Birnstiel et al., 1968). Furthermore, recent studies with the electron microscope have provided evidence for a common organization of the rRNA genes in all animals examined (Schibler et al., 1975). A model for the repeating unit, which probably applies also to plants, for it is compatible with all the available evidence, is shown in Fig. 9.12.
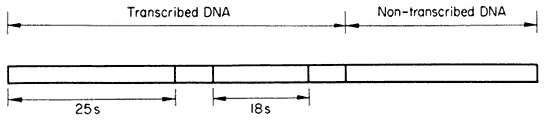
Figure 9.12
Suggested structure of the repeat unit of ribosomal DNA in plants.
(Modified from Schibler et al., 1975.)
By carrying out partial denaturation studies it has proved possible to map certain areas rich in GC or AT base sequences. In addition, it is known that both the non-transcribed 'spacer' regions and the transcribed spacer can vary significantly in length and composition between species. Possible functions for such regions are discussed below. In contrast, however, the regions that code for rRNA are remarkably constant in composition. This probably reflects the importance of their role in providing structural RNA for ribosome formation.
As shown in Fig. 9.9, chloroplast rRNA also hybridizes to nuclear DNA (Ingle et al., 1970; Tewari & Wildman, 1968). This is a surprising result in view of the fact that chloroplasts contain their own DNA and synthesize their own rRNA (see chapter 11). The location of these genes within the nucleus is not known, and at present there is no evidence that they are ever transcribed in the nucleus.