Preferred Citation: Sauer, Jonathan D. Plant Migration: The Dynamics of Geographic Patterning in Seed Plant Species. Berkeley: University of California Press, c1988 1988. http://ark.cdlib.org/ark:/13030/ft196n99v8/
![]() | Plant MigrationThe Dynamics of Geographic Patterning |
Dedicated to three of my teachers
who were students of plant migration:
Preferred Citation: Sauer, Jonathan D. Plant Migration: The Dynamics of Geographic Patterning in Seed Plant Species. Berkeley: University of California Press, c1988 1988. http://ark.cdlib.org/ark:/13030/ft196n99v8/
Dedicated to three of my teachers
who were students of plant migration:
Preface
Ever since I was a botany graduate student in the 1940s, I have been fascinated and bothered by the field that is usually called historical plant geography. Wulff (1943) accurately depicted the state of the field at that time, and a perceptive resurvey (Stott 1981) shows it has changed little since then in basic concepts or methods. These were largely developed during the nineteenth century as biogeographers came to recognize that spatial distributions required explanation by both present and past processes of dispersal and environmental control. The need for historical explanation became obvious as soon as maps of species and other taxa revealed range disjunctions too wide to be bridged by normal seed dispersal. Nondisjunct ranges, although less provocative, have sometimes also evoked historical explanations.
Unfortunately, in many taxa and regions plant geographers lacked fossil or other genuine historical evidence and could construct only hypothetical migration stories deduced from present patterns. Historical plant geography has relied so heavily on these deductions that the name of the field is something of a misnomer.
The field has always depended on a typological approach: distributions of species or other taxa are classified on the basis of rough similarity into types, such as arctic–alpine, eastern North America–western Europe disjuncts, and South African endemics. A basic assumption is that taxa have similar present ranges because of shared migrational patterns rather than because of convergence from formerly dissimilar ranges.
This approach has had some notable successes, particularly in deducing continental drift long before plate tectonics became accepted. I believe this approach will continue to play a fruitful and respectable role in plant geography provided that its deductions are recognized as working hypotheses and that it works with alternative hypotheses, not just the simplest one. I am troubled by a common tendency to accept untested hypotheses as established
truth and to form partisan bands championing certain deductive methodology. Currently one of the most dogmatic of these bands claims universal validity for their incredibly simple cladistics–vicariance methodology; their claims have been thoughtfully criticized by Stoddart (1981).
Vicariance and similar approaches are beguiling because they promise grand leaps from ancient origins to explanation of modern patterns without the difficulties of working out intervening processes. Historical biogeography has been mainly in the hands of taxonomists working with museum collections, which are poor clues to processes. Herbarium taxonomic methods implicitly recognize this weakness by the practice of pooling all locations of a taxon, as if they were contemporaneous, and plotting them only on small maps, often the size of postage stamps. As a result, only gross migrations and grand environmental controls are likely to be postulated; changes in sea level, continental positions, mountain ranges, and climate have been especially favored. Migrations are commonly visualized as proceeding ponderously through geologic time spans. For example, modern species ranges may be assumed to be still shaped by locations of Pleistocene refugia, with no mechanism for such slow migration discussed.
In this book I want to try a completely different approach: a survey of case histories for which there is direct evidence of the process of migration through time. Unlike taxonomists committed to the study of a particular taxonomic group or regional flora, this survey can focus on cases with real time depth in the evidence. There are many such case histories, minor and grand, that have not been assimilated into the literature of historical plant geography. Most of these come from research not concerned with the process of migration per se, for example, by ecologists more concerned with changing community composition than with changing species borders, by paleoecologists mainly concerned with past landscapes and climates, or by paleobotanists mainly concerned with phylogenies. This kind of inductive approach can promise only limited generalizations, not any universal synthesis, but I believe it will show that real migrations are more interesting than hypothetical ones.
The references cited are not exhaustive but are intended to give initial access to the literature. They contain extensive reviews of earlier sources. Complete citation of relevant sources would expand the bibliography to more than ten times its present length.
Research assistance was given by Charmion Burns and Eileen Killory Sauer. The manuscript was word processed by Tina Schroeter. The maps were prepared by Amalie Jo Orme.
For helpful criticism of portions of the manuscript, I am indebted to Daniel I. Axelrod, Herbert G. Baker, Karl W. Butzer, Margaret B. Davis, Richard A. Minnich, Garry F. Rogers, Matsuo Tsukada, Philip V. Wells, and Jack A. Wolfe.
Introduction
The Concept Of Migration In Seed Plants
In different branches of biogeography, the term migration is applied to such diverse processes as diurnal vertical movements of plankton, mass seasonal journeys of animal populations, and gradual advances and retreats of plant species borders with changing climate. All these fit under a broad definition of migration as any change in spatial distribution of a species or other taxon through time. In this sense, organisms in general are capable of migration, although mobile stages in the life cycles are quite different. In the case of seed plants, the new diploid embryo is normally mobile while juveniles and adults are sedentary, which is quite the opposite of many animals. Even the seed plants' closest relatives, the ferns, live in a different world as regards migration processes. Ferns generally release astronomical quantities of microscopic spores that commonly remain viable for months and can be carried indefinitely around the world by wind. If it arrives in a suitable site, a single bisexual fern spore can establish a new sexually reproducing colony. Like other spore—bearing plants, ferns may follow Beijerinck's Law: everything is everywhere but the environment selects. Wide geographic gaps in suitable habitats mean little to such plants. Even on the most remote oceanic islands, ferns have relatively low endemism (Tryon 1970). Pollen of some seed plants is also carried great distances by the wind and can maintain gene flow between isolated populations of the species, but pollen cannot found a new population of a species where it is not already established.
In the seed plants, for good practical and theoretical reasons, species distributions are customarily based on locations of successfully established plants, mainly sexually mature adults. Ungerminated seed and young seedlings are seldom noted or collected and are often unidentifiable; moreover, they are not contributors to the future of their species. In this book, migration
is defined as any change in the distribution of successfully established mature plants as the years and generations pass. The term includes both advances and retreats and involves both continuous and disjunct ranges.
Model Of The Mechanisms Of Geographic
Patterning In Seed Plants
The world has about 250,000 living species of seed plants, each with a unique present geography and migrational history. However, the process of geographic patterning can be generalized in a very simple abstract model. Geographic patterning results from interplay between opposing forces: a positive centrifugal force that tends to expand the area occupied by the species versus a negative centripetal force that tends to limit the area.
Positive force . The main centrifugal force is seed dispersal. By definition, a seed contains a new embryo enclosed in an integument or seed coat; it usually also contains an endosperm or food storage tissue. The dispersal unit may be a seed, pure and simple, for example, a pine nut or a bean; commonly the dispersal unit includes surrounding ovarian or other maternal plant tissue, such as a coconut or a plumed dandelion achene. The wonderful variety of these associated structures and their role in seed dispersal by various agencies have been systematically surveyed (Kerner von Marilaun 1896; Mueller-Schneider 1977; Pijl 1982; Ridley 1930). Dispersal will be a constant concern in tracing case histories of migration. Clonal spread and vegetative propagation may contribute to the positive force, usually on a local scale.
Negative force . The main centripetal force is environmental control of seed germination and of plant development. Identifying the limiting environmental variables is often the knottiest problem in understanding species borders.
Equilibrium . A species border may be static even though dispersal and environmental selection are proceeding actively: recruitment and mortality are in balance. For example, Canavalia maritima , a pantropical seashore vine, has buoyant seeds that remain viable for many months while drifting in the sea. Its seeds are familiar to beachcombers worldwide, but the species is established only in humid tropical climates. Herbarium records of the species show no change in its gross range since the eighteenth century. Such equilibrium may be expected when dispersal and environment have been stable long enough.
Initiation of migration . When a border is moving, the first question is whether the lack of equilibrium is due to a change in dispersal or environment or both. When a border is advancing, a further question is whether it is keeping up with and controlled by a gradually shifting environmental limit or whether it is free from environmental restraint and limited only by dispersal. When a border is retreating, its position may lag behind a shifting environmental control, with relict populations persisting where they can no longer establish or reproduce themselves.
Problems Of Scale
As noted above, the mechanism that maintains a static species distribution when recruitment and mortality are in balance is the same mechanism that causes migration when they are not. Thus, whether a species distribution is perceived as static or dynamic may be simply a matter of the scale of study. If locations of individual plants are plotted on very detailed maps, micromigrations would be perceived that on a more generalized map would be considered mere changes in density within a static range. Intuitively, biogeographers tend to consider micromigrations as trivial and macromigrations as significant, but the difference may be elusive.
Similarly, drawing a line between local and long-range dispersal may be quite arbitrary. Purely local seed dispersal has survival value in allowing escape from competition with the parent plants and reducing density-dependent mortality from disease, seed predation, and competition between seedlings. Selection would not be expected to develop adaptations for long-range dispersal if the habitat occupied by a species is spatially continuous and truly isolated from other suitable sites. However, in the more usual pattern where suitable habitats are patchy with gaps of highly variable widths, selection would be expected to favor longer range dispersal. In some species, adaptations for seed dispersal function over a broad spectrum of distances. For example, two wide-ranging seashore shrubs of the Indian and Pacific oceans, Scaevola taccada and Tournefortia argentea , have seeds that remain dormant on the ground under the mother plant even when rained upon. If washed away by waves, they remain dormant while floating in the sea, sometimes for over a year. However, after incubation in seawater, if they are stranded and rained upon, dormancy is broken (Lesko and Walter 1969). The distance carried by ocean currents is apparently irrelevant.
Similar examples are found in other habitats. Some tropical riverbank lianas, for example, Dioclea, Entada , and Mucuna spp., have extremely hard, buoyant seeds that are indifferent to the length of time they float. Whether
they float a few meters or a few hundred kilometers down a river, they remain dormant until stranded and buried in sediment, where the seed coat is presumably attacked by microorganisms. A burr tangled in animal fur may be carried for minutes or weeks. Seeds that remain viable while passing through the digestive tract of waterfowl may remain in the same lake or be dispersed on long-range migrations. Some plants have apparently evolved separate adaptations for local and long-range dispersal, for example, Cakile spp., the sea rockets. These are quick-growing annuals that colonize ocean beaches, mainly during the quiet summer season. When the seeds are ripe, the indehiscent pod breaks in two. The bottom segment with half the seeds remains attached to the dying mother plant, which commonly gets buried in the sand; the top segment with the rest of the seeds is commonly washed away by storm waves to drift in ocean currents.
Limitations Of The Data Base
The same mechanism causes trivial micromigrations and significant macromigrations; there is no theoretical basis for drawing a line separating them. However, in practice there is no danger of becoming bogged down in cases of trivial changes in distribution because the available data seldom reveal changes in such detail. Available data on distribution of both plant species and environmental variables are generally very sketchy, even for recent time in better known regions. The ideal would be time-lapse synoptic maps based on field surveys at whatever scales and time intervals were appropriate for the scope and rate of migrations. Instead, migrations have to be traced from an irregular patchwork of bits of information with many gaps in space and time.
Ideally, also, maps should distinguish between three kinds of areas: a reproductive core in which plants are producing seed, a marginal establishment zone where plants are not reproducing, and an outer seed shadow where seed germination is suppressed or abortive. Data to draw such maps are simply nonexistent. In practice, the reproductive core and marginal establishment zone are usually lumped and the outer seed shadow excluded from the definition of the species range. Because gene flow is unidirectional from the reproductive core to the other zones, environmental conditions in those zones play no role in shaping adaptions of the species. Of course, with a change in the habitat, the outer zones may become part of the reproductive core. A dramatic example followed drainage of the Northeast Polder in the Netherlands, when 48,000 ha (hectares) of former seafloor were exposed.
Nobody suspected that seed carried out to sea by wind and streams was lying dormant in seafloor sediment. Botanists who had expected to follow gradual plant colonization of the new land were amazed to see masses of vegetation spring up from accumulated seed even before the land dried out. Some dominants were salt marsh species, such as Aster tripolium and Scirpus maritimus and some freshwater marsh species, such as Senecio palustris and Phragmites australis . The exploding population of Senecio palustris produced wind-borne seed in quantities never seen before, which started new mainland colonies, some over 300 km away (Bakker and Zweep 1957; Schroeder 1969).
Distribution of marginally established plants outside the reproductive core sometimes fluctuates greatly from year to year and may be mistaken for a long-term trend. In Britain, rare wild orchids are a favorite target of plant watchers, who have kept detailed records of historical occurrence. Two of the most sought after are Himantoglossum hircinum and Orchis militaris , natives of dry, rocky, calcareous sites. Both are common on the European continent but extremely rare in Britain, being found mostly as single plants at widely scattered localities, flowering irregularly, and rarely surviving more than 1 to 2 years. Britain is evidently outside the reproductive core of these species, but within the seed shadow of the continent (Good 1936; Perring 1974). An even rarer orchid in Britain is Spiranthes romanzoffianum , native to wet meadows and bogs scattered widely across North America. Its dustlike wind-borne seeds evidently cross the Atlantic only rarely. It appeared in a few wet meadows and bogs in Ireland in the nineteenth century and at a few others in Scotland and England since 1900. Perring (1974) suggested that some sea beach plants that are extremely rare in Britain may normally be recruited from immigrant, sea-dispersed seed rather than British seed. Abundant in the Mediterranean region, they appear in Britain only as precarious ephemeral colonies. Although the number of occurrences of these species in Britain may be fairly constant over the long run, their situation can be alarmingly misconstrued by noting the few extant colonies on maps that show the sum of former occurrences.
Sedentary ephemeral species, for example, desert annuals and fire-following herbs in chaparral, present a special problem in defining species distributions and migrations. They appear only briefly after good rains or burns and then disappear for many years. Some species can evidently survive in situ as long-dormant seed between cyclical appearances. Some others with less long-viable seed may survive by local migration between sites.
I propose that we recognize once and for all that the data base is imperfect and, instead of harping on its imperfections, proceed to see what we can do with it.
Exclusion Of Artificially Controlled Migrations
The historical geography of cultivated plants is the subject of an extensive literature that we shall not survey here. Being under deliberate and continuous human control, cultivated plant migrations lie less in the domain of natural history than of cultural and economic history. We shall consider only a few cases of cultivated plants that have escaped and become naturalized. Neither shall we survey cases of nonselective obliteration of plant species by permanent clearing for pasture, agriculture, or urbanization; the process is too obvious and ubiquitous to need presentation of examples here.
Cases will, however, be included that involve human modification of species distributions. In most of the real world, it is impossible to exclude the agency of Homo sapiens during at least the last 10,000 years. Even if feasible, it would be artificial to segregate fires set by lightning and by humans or acorns dispersed by birds and by humans. Moreover, as has been generally recognized in the study of evolution since Darwin and Mendel, some of the best evidence on how a natural process operates can be obtained by observing effects of human intervention.
Definition Of Weeds
In folk usage, the word weed is used in a variety of ways, for example, for a useless, obnoxious, or ugly plant. Whether a plant is a weed in this sense depends entirely on who is looking at it.
The term will be used here in an ecological, not psychological, sense. Ecologically defined, plants are considered weeds when they volunteer in artificially modified habitats. The same species may occur elsewhere in a wild state, that is, in completely natural habitats, or in cultivation. For example, pokeweed, Phytolacca americana , has its main populations as uninvited weeds in gardens, roadsides, and other human-modified habitats, but it grows wild along riverbanks and around badger diggings and is occasionally cultivated as an edible potherb and sold by nurseries as an ornamental.
Definition Of Natives
The word native is sometimes used as synonymous with indigenous to indicate the place of origin. In this sense, Britain would have almost no native fauna or flora, and much of the natural flora of California would have to be
classed as immigrant. For many plant species, even the general region of origin is unknown.
The general practice in botanical literature is to consider a species native wherever it is believed to have arrived and established itself without human help. Even species that evidently arrived recently, such as Spiranthes romanzoffianum in Britain (mentioned above) are commonly included in the native flora if not believed to be human introductions. The standard botanical definition of native species will be followed here but with a time dimension added. In cases of known migration, a species will be considered native where it was evidently naturally established at the start of the record; it will be considered an immigrant if it arrived thereafter.
Part I
Modern and Historical
Migrations
Rather than proceeding chronologically, we shall start with recent cases because in them we can observe the cause and course of migration most clearly. In prehistoric migrations, movement of species borders, changes in dispersal, and even the environment cannot be observed directly, but have to be inferred. I believe background knowledge of relatively clear-cut recent cases will help interpretation of more ancient ones.
Much of the subject matter of recent plant migrations is shared with ecological studies of vegetational succession, but the approaches differ. Succession is usually treated as change in a community as a whole within fixed spatial borders. Both the standard relay floristics model and the initial floristics model of succession usually assume a constant pool of available species, whether the seed source is inside or outside the borders of the study area (Egler 1954; McIntosh 1980). Variations in seed availability are often treated as random complications not covered by the model. The focus here will be on migrations of species individually, with the changing spatial patterns of dispersal and survival treated as integral variables in the process.
1
Shoreline And Other
Naturally Open
Habitats
Mangrove Swamps
By definition, mangroves are trees rooted in substrates that are flooded by seawater, either constantly or periodically. Mangroves belong to many different angiosperm families, some of which also include upland genera, but all the mangrove genera are tightly confined to their saline swamp habitat. Most mangrove genera are tropical, with a minority ranging into the subtropics. Members of the genus Avicennia, a tree belonging to the Verbena family, range farthest into temperate regions. Within their latitudinal limits, mangroves have very broad edaphic and climatic tolerances. Because they are able to draw water molecules and nutrients from the sea, they are remarkably indifferent to substrate, growing in rock crevices and many kinds of sand and mud. Where there is good circulation of seawater, they are also remarkably indifferent to rainfall, growing adjacent to rain forests, deserts, and everything between. Nevertheless, suitable mangrove habitats are extremely discontinuous because of the need of shelter from wave action for seedling establishment.
Within a mangrove forest, species patterns often show striking zonation, which has often been interpreted to be the result of autogenic succession: a pioneer fringe advances seaward, traps sediment, builds up land, and prepares the way for advance of the next zone. However, case histories show the dynamics are usually more complex.
River Deltas, Southern Gulf of Mexico
(Thom 1967; West et al. 1969)
The great uncontrolled rivers of Tabasco and Campeche have provided a dynamic habitat complex in which mangrove distribution patterns are continually changing. In historical time, active river mouths have switched between some distributary channels that fan out over the deltas. During floods, active distributaries build ever higher and wider natural levees along their channels. The sand they pour into the Gulf of Mexico is carried by longshore currents to be laid up by waves as a series of accretion beach ridges parallel to the shore. The constant loading of additional sediment causes regional subsidence of the whole delta. When a distributary is becoming inactive, the old channel is slowly filled with fine sediment deposited by general floods during the winter rains. During the dry season, saline water invades abandoned stream channels and lagoons, and penetration increases during the years as subsidence goes on. Water levels are less affected by the trivial lunar tides than by changes in wind direction, the highest levels coming during coincidence of maximum river discharge and strong north winds during winter. These northerly storms, called nortes, also cause severe beach erosion. After the sand supply has been diverted by a shift in a river mouth, huge areas of beach ridge systems near the abandoned mouth are eventually lost to the sea.
The dominant mangrove species in this region are Rhizophora mangle, Avicennia germinans, and Laguncularia racemosa ; all produce nondormant seedlings capable of long flotation. The mangroves do not colonize natural levees being built by active distributaries; there the first colonists are marsh plants, such as Phragmites, Spartina, and Typna spp. When a distributary is being abandoned and becoming saline, Rhizophora seedlings floating in from the sea usuallv colonize the banks. The tangle of stilt roots of the Rhizophora fringe catches debris and sediment, and the mangroves add organic detritus of their own. As the channel fills and narrows, the Rhizophora fringe widens by establishment of new seedlings, while Avicennia and Laguncularia seedlings join the older Rhizophora in the rear, forming a mixed mangrove forest. As long as peat formation under the mixed mangrove forest compensates for subsidence, the species may persist in situ indefinitely unless the forest is destroyed by retreat of the seashore or is overrun by a new active stream channel.
A different geomorphic—biotic sequence begins on the back side of the natural levees, which slope gradually to mudflats along seasonally saline lagoons. As the mudflats are built up by sedimentation during floods, they are first colonized by Spartina and Batis maritima, a prostrate perennial halophyte. Here Avicennia is usually the pioneer mangrove, with the other
two joining in as sedimentation proceeds. When the distributary becomes inactive and sedimentation is shut off, subsidence will cause the mangrove fringe, with Avicennia in the lead, to move toward the crest of the sinking levee. Lagoons enlarged by subsidence eventually have enough fetch for generation of waves, which undercut the mangroves and wash them away.
Thom (1967) postulated a variety of other geomorphic sequences in this deltaic complex. His general conclusion was that in certain situations, mangroves trap sediment and lay down peat so that vegetationally controlled, autogenic succession may temporarily prevail, but that over the long run habitat changes controlled by the mangroves are overwhelmed by a grand geomorphic cycle controlled by the rivers and the sea.
Coral Cays, Belize
(Stoddart 1962, 1963, 1969)
Mangroves occupy a totally different geomorphic setting on the other side of the Yucatan peninsula, where they grow on coral cays with no river or terrestrial sediments. The cays lie on the barrier reef and on atoll-like structures beyond. The cays most exposed to wind and surf have beach ridges built of coarse coral debris, which are colonized by species discussed in the next chapter. On these exposed cays, mangroves are confined to a fringe on the leeward shore. On more sheltered cays, typically shoals with no dry land, mangroves occupy the whole area. Rhizophora mangle is generally strongly dominant, particularly on the outer margins of the mangrove forest, joined by Avicennia germinans and Conocarpus erectus on slightly higher areas. Laguncularia racemosa occurs infrequently.
Autogenic succession, with mangroves advancing as they trap sediment and lay down peat, cannot be a general process in these cays. Some of the mangrove cays apparently have permanent shorelines with rock foundations rising from fairly deep water. There, mature mangroves grow to the edge with no seedling colonization beyond. Other unconsolidated cays are gradually migrating shoreward; whether they are constant in area, increasing, or disappearing probably depends on the reef flat topography, sand supply, waves, and currents, not on the vegetation.
Catastrophic changes in mangrove patterns were documented by Stoddart's (1962, 1963, 1969) surveys before and after Hurricane Hattie in 1961. His 1960–1961 maps of the cays show vegetation patterns that may have been in approximate equilibrium. Most of the cays had not had a major hurricane strike for 30 years; the southernmost part of the surveyed area may have had hurricane damage 15 years before. The center of the 1961 cyclone passed directly over the cays. Sustained wind speeds were estimated at 250
km/hr with gusts to 320 km/hr. Atmospheric pressure was so low that over an area about 100 km in diameter, the sea rose about 5 m above normal level. Huge waves were superimposed on this storm surge.
The storm broke branches and stripped leaves from mangroves over a wide swath. Stoddart's 1965 resurvey found mangroves were generally dead within a swath about 65 km wide, the only survivors being in very small patches on the leeward sides of the larger cays. Within the dead stands, the ground was still occupied by the old roots and there were a few seedlings. New seedlings were establishing mainly in new bare areas.
Great Barrier Reef, Queensland
(Hopley 1982; Stoddart 1980; Stoddart et al. 1978)
Queensland, Australia, has a much richer complement of mangroves than the neotropics, with 27 species belonging to many different angiosperm families at least locally dominant. All are sea dispersed and shared with the East Indies. With ocean swell shut off by the Great Barrier Reef and a tidal range of 3 to 6 m, mangrove swamps require only minimal topographic shelter and are very extensive.
Within a mangrove stand, species commonly show rather regular zonation, which has evoked deductive models of predictable, autogenic succession. However, where distribution patterns have actually been monitored over time, in some cases by detailed remapping over nearly 50 years, irregular and unpredictable changes were found. Rather than actively advancing by autogenic succession, the mangroves generally passively follow changes in reef morphology with coral growth and destruction.
Naturalized Mangroves, Hawaii
(Wester 1982)
Hawaii is better situated to receive drift from Alaska than from the tropical Pacific. It had no native mangroves, the nondormant seedlings evidently being unable to survive the circuitous voyage up the coast of Asia, across the northern Pacific, and back down to the tropics.
In 1902, the American Sugar Company introduced Rhizophora mangle from Florida to Molokai, Hawaii (fig. 1), where it was planted on the south coast in an attempt to stabilize sediment produced by erosion on the slopes of the island. Seedlings were transplanted from Molokai to Oahu and have since volunteered on the islands of Hawaii, Maui, Lanai, and Kauai, evidently
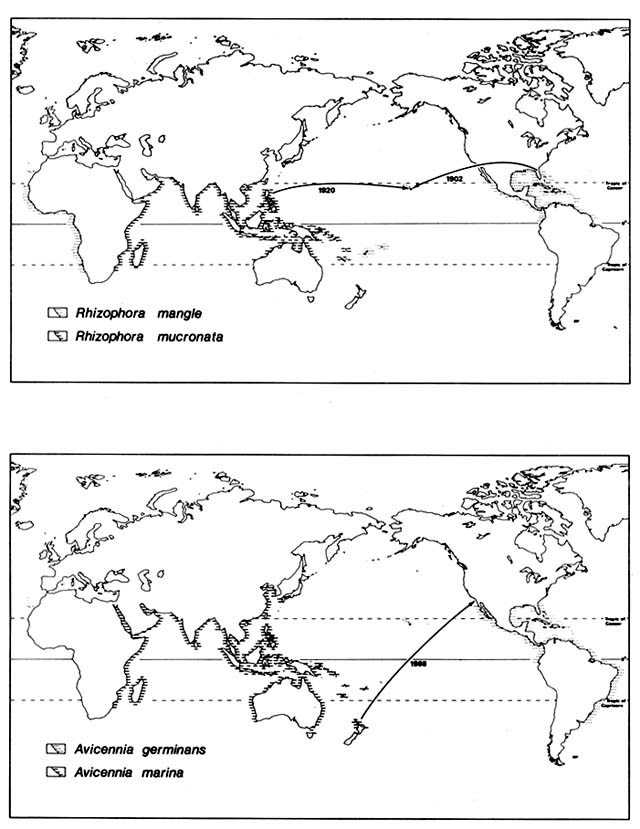
Figure 1. Recent Introductions of Certain Mangroves Outside Their Native Ranges. Mangrove species
generally are well adapted for ocean disposal and have very broad natural ranges. They were, however,
unable to reach some suitable habitats as shown by naturalization following recent artificial introduction.
spreading within the archipelago by natural drift. The neotropical button mangrove, Conocarpus erectus, was also planted in various places along the coast but has not naturalized.
In 1922, four Philippine mangrove species were introduced by the Hawaiian Sugar Planters Association and planted on mudflats around Oahu. Ceriops tagal promptly died; Rhizophora mucronata and Bruguiera parviflora survived for a few years. Bruguiera gymnorhiza has survived and reproduced in several sites on the Oahu coast, in spite of much exploitation of its flowers for leis.
Some of the Hawaiian mangrove colonies depend on artificially modified habitats. The prehistoric rock-walled fish ponds, built in the sea by the Polynesians, offer sheltered habitats for dense mangroves. Mangroves infest canals, including the Ala Wai behind Waikiki, where they must be periodically removed. Extensive stands occupy deltas below sugar mills, where sediment from cane washing is deposited. A 40-acre stand of Rhizophora mangle colonized such a delta in Pearl Harbor between 1951 and 1975. However, other colonies are established on shores naturally sheltered by reefs and embayments. Presumably, these would have been colonized prehistorically if viable seedlings had arrived.
Naturalization of Avicenna, California
(Moran 1980)
Several neotropical mangrove species range naturally up the Pacific coast of Mexico across the Tropic of Cancer. They extend above 29°N latitude in the Gulf of California, but none extend above 27°N on the Pacific side of Baja California.
In 1963, two of these species, Avicennia germinans and Lagunclaria racemosa, were planted experimentally at about 32.5°N latitude in California. The site is a salt marsh dominated by Spartina foliosa on Mission Bay in San Diego. It is administered as a nature reserve by the University of California, San Diego. Neither of these mangroves succeeded, although one Laguncularia survived for several years.
In 1968, seedlings of two Indo-Pacific mangroves were planted in the same marsh: Aegiciras corniculatum from tropical Queensland and Avicennia marina from 37°S latitude in New Zealand. The Aegiciras has survived and flowered but not reproduced. Avicennia marina established thousands of seedlings, now in the third generation; its success threatened the native biota of the marsh. Since 1976, university students have stopped Avicennia reproduction by removal of flowers and seedlings; in 1979 about 12,000 rooted seedlings were pulled up, but a few were left.
Comment
Normal dispersal of mangroves is entirely by ocean currents. Many species are viviparous, that is, seedlings germinate before release from the mother tree and continue to develop while floating. With light, water, and nutrients available, dormancy is pointless. Seedlings occasionally strike root in rock crevices, even on open shores. On loose substrates, they need quiet water. Even where there are only gentle waves, it is common to find dead and dying mangrove seedlings stranded in the drift zone. on the new island of Krakatau (discussed in the section on Fresh Lava and Pyroclastic Deposits), live seedlings of various mangrove species have repeatedly been stranded, but have failed to establish for lack of shelter.
Once established, mangroves are very resistant to storm and wave damage. The catastrophic destruction in the Belize cays (discussed above) is an extreme case. The same hurricane struck Grand Cayman Island less violently than Belize, but Grand Cayman had heavy surf and winds over 100 km/hr; these had little effect on the mangroves. Part of the distribution of mangroves is too close to the equator to be subject to any cyclonic storms. In some sites, mangroves survive in situ indefinitely, as shown by deep peat deposits. However, many populations are only temporary and are inevitably destroyed by gradual or catastrophic habitat changes.
Both the highly disjunct spatial pattern and the temporary survival of local populations imply strong natural selection for dispersibility and perhaps for long-range dispersibility. It is sometimes argued that plants do not evolve adaptations for long-range dispersal through natural selection but that long-range dispersal happens only occasionally and incidentally through adaptation for local dispersal. However, in the case of species obligately dispersed by ocean currents, there is complete elimination of any disseminules that do not complete the voyage to another shoreline. Seeds dropped at random in wind or by bird dispersal have some chance of survival, but seeds that sink at sea do not. Selection in mangroves would not favor long-range dispersal per se but rather long buoyancy, regardless of the rate of drift and distance covered. The more sites, near or far, that a seed or seedling can probe, sometimes by repeated stranding and refloating, the better chance of finding a safe place to take root. In the tropical Indo-Pacific region, which is the center of mangrove diversity, archipelagos of islands offer all degrees of spatial disjunction between suitable sites. Also, the seasonal reversals of monsoonal winds and ocean currents allow genetic feedback from successful distant colonies.
Not all mangroves are known to be capable of long ocean voyages, but various species have ranges spanning the Indian and western Pacific oceans; others span the Atlantic. That their dispersal capacities are not unlimited is shown by the lack of any pantropical species and by the absence of mangroves from Hawaii before the introductions discussed above.
Tropical Sea Beaches
Coral Cays, Belize
(Stoddart 1962, 1963; Stoddart et al. 1982)
Supratidal vegetation, as well as the mangroves discussed above, was mapped on coral cays in Belize just before the devastating hurricane of 1961. The interval since the last storm had probably been long enough for the beach vegetation to reach near equilibrium. The more exposed beaches had a sparse, low outpost vegetation of perennial herbs and vines, all well adapted for long-range ocean dispersal. Many are pantropical, including a beach grass, Sporobolus virginicus ; a beach morningglory, Ipomoea pes-caprae ; and sea purslane, Sesuvium portulacastrum . Others are also widespread but only within the Caribbean region, for example, a spider lily, Hymenocallis littoralis , and a bushy spurge, Euphorbia mesembrianthemifolia .
The larger cays had beach ridge thickets of spray-swept shrubs and trees; these are adapted for both sea and bird dispersal. The dominants included some pantropical species, such as Suriana maritima and Sophora tomentosa , but most are neotropical, such as Thrinax radiata, Coccoloba uvifera, Cordia sebestena, Bursera simaruba, Conocarpus erectus, Tournefortia gnaphalodes, Borrichia arborescens . The East Indian almond, Terminalia catappa , was naturalized on some cays. Many had been largely cleared for coconut plantations.
This beach vegetation was almost identical to that of the Cayman Islands. Before striking Belize, Hurricane Hattie passed close enough to Grand Cayman to cause washing out of the outer few meters of vegetation on some beaches, but the recovery was completed in about 5 years.
On the Belize cays, Hurricane Hattie caused catastrophic damage to beach vegetation over a swath about 65 km wide. Destruction was not as total as in the mangroves. Outpost beach vegetation was damaged mainly by storm waves reworking the substrate. In the most extreme cases, vegetated cays as long as 120 m were completely washed away and have not since reappeared above the sea. More commonly, the outpost vegetation merely lost some ground to beach erosion and washovers or was buried under fresh coral rubble. Parts of the cays were stripped of all loose sand and shingle down to beachrock. In places, losses were selective: waves swept away Ipomoea and Sesuvium but left Sporobolus , and Hymenocallis reemerged where neighboring species were killed by burial.
The beach ridge thickets suffered less from direct wave action than from defoliation and breakage. Here, also, losses were partly selective, Cordia being uprooted more often than neighboring species. The native Thrinax palm stood up to the wind better than the planted Cocos .
Within 6 months, outpost species were recolonizing some of the bare sand, either vegetatively or by seedlings; Sporobolus, Sesuvium , and Ipomoea were among the first. Seedlings of a thicket shrub, Tournefortia , were common. The former distinction between zones was blurred, with outpost species appearing inland of their former position. Some weedy annuals that were not noted on beaches before the hurricane, for example, Portulaca oleracea and Cenchrus incertus , were prominent as early colonizers of fresh sand.
By 4 years after the storm, there was vigorous but patchy colonization of loose sand by both outpost and thicket species. All the outpost species that were common before the storm were well represented and were displacing Portulaca . Among the shrubs that had been prehurricane dominants, Tournefortia was best represented by seedlings; there were also some seedlings of Suriana, Sophora, Thrinax, Conocarpus , and Borrichia . The other thicket species were present as survivors but not as recolonists. Areas of stripped beach rock, coarse shingle, and mats of dead coconut roots remained mostly bare.
By 11 years after the storm, effects of the hurricane were no longer generally obvious except for relic dead and broken trees. Resurveys commonly found more species than the prehurricane roster, perhaps because of more thorough search. However, only a few seedlings of some formerly important thicket species had appeared: Coccoloba, Cordia, Bursera , and the naturalized Terminalia .
Comparative mapping over the same 11-year time span extended to cays south of the swath of Hurricane Hattie. These showed less change, of course, but they still showed quite dynamic cay morphology and vegetation patterns. The maps usually showed only single dominants for each area, so changes may be quantitative rather than advances and retreats of species borders. However, they leave no doubt that micromigrations have gone on, partly as recovery from a storm 15 years before Hattie and partly as a result of chronic instability of sand cays. During the decade, a few species became extinct on some of the smaller cays; some of these had been present originally as individual bushes or trees.
Coral Sand Beaches, Mauritius
(McIntire and Walker 1964; Sauer 1962)
Mauritius is a large, rugged volcanic island, but along with basalt sea cliffs and boulder shores, it has extensive coral sand beaches behind fringing reefs.
Early in 1960, after 15 years without any significant storms, Mauritius was raked by two strong cyclones; the second, Carol, was probably the most
intense in the recorded history of the southwestern Indian Ocean. Instrumentally recorded wind velocities at several stations on Mauritius reached 240 km/hr. Waves superimposed on the storm surge left piles of drift 3 to 4 m above normal wave reach on exposed coasts.
A few months before the storms, the coastal vegetation had been surveyed with belt transects and maps of small sample areas; the vegetation was then in apparent equilibrium and neatly zoned. Outpost vegetation on coral sand beaches was dominated by the same pantropical herbs and vines as on Belize cays. Beach ridge thickets also had the same pantropical shrubs, but two of the dominants were Indo-Pacific species, Tournefortia argentea and Scaevola taccada . Inner beach ridges behind the thickets were generally planted with an introduced timber tree, Casuarina equisetifolia . There were only a few coconut plantations.
Here, as in Belize, destruction by the hurricane of beach outpost vegetation was mostly due to reworking of the sand substrate by storm waves rather than to direct damage to the plants. This was dramatically evident where the same species survived pounding by storm waves where rooted in crevices on basalt but were completely swept away where rooted on sand. Waves also bit into the outer margins of thickets on loose sand and shingle ridges, but survivors of all the species were left in each site.
By contrast, destruction of Casuarina trees extended far beyond the reach of the waves, and mortality in large areas was nearly total. The coconut plantations had mortality of only about 25%.
Three months after Cyclone Carol, a resurvey showed complex changes in beach profiles but little change in shoreline positions because sand had been redeposited as the storm abated. Most of the area reworked by waves was still completely bare, but recolonization was beginning. The commonest new colonists of the outpost zone were Canavalia maritima seedlings and vegetatively propagating Ipomoea pes-caprae and Cyperus stoloniferus , the latter more abundant than before the storm. Some beaches were invaded by seedlings of inland weeds, such as the Mexican prickly poppy, Argemone mexicana , perhaps aided by low salinity after the heavy rains. Except for these weeds, recolonization of the outpost zone was invariably by species that had been present in the same sites before the storm. This outcome was not inevitable, since Ipomoea pes-caprae was the only species that had been present in over 50% of the sites. The storm was evidently ineffective in coastwise dispersal within the outpost zone, probably because seed afloat during the storm was stranded mainly in the thicket zone or farther inland.
In the thicket zone, a few survivors of all the previously present species were found in almost every site; the dominant Tournefortia and Scaevola were resprouting vigorously where damaged. Shrub and tree seedlings were generally few.
Where the storm waves had left bare sand back in Casuarina and Cocos
plantations, there were many new seedlings of species not present before the hurricane. These included all the common outpost and thicket species and also some inland weeds, for example, Portulaca oleracea and Ricinus communis , and crops, for example, Nicotiana tabacum and Ipomoea batatas . Drift stranded during the storm evidently contained a mishmash of propagules derived partly from excavation of beaches and partly from flooding rivers.
During 1961 and 1962 several other cyclones passed near enough to Mauritius to produce heavy surf and beach erosion, mainly on the west coast. The strongest, in 1962, had winds gusting to 200 km/hr. When revisited in 1963, west coast beaches showed additional losses of shrubs that had survived Carol in 1960, but new colonies of outpost herbs, especially Sporobolus and Cyperus , had appeared. On the east coast, recolonization by these and other outpost herbs and vines was further advanced; there were also scattered seedlings of thicket shrubs, mainly Scaevola . The wreckage of the Casuarina plantations had mostly been converted to charcoal, and some replanting had begun.
Pyroclastic Beaches, Krakatau
(Docters van Leeuwen 1936)
After the cataclysmic eruption of Krakatau in 1883, the first expedition to the new island found no sign of plant life. After 15 years, the beaches of volcanic ash and sand had acquired a rich flora including outpost vines, such as Canavalia maritima and Ipomoea pes-caprae ; thicket shrubs, such as Scaevola taccada and Morinda citrifolia ; and beach trees, such as Cocos nucifera, Casuarina equisetifolia, Terminalia catappa, Calophyllum inophyllum , and Pongamia pinnata . Within another 10 years, the island had nearly all the species to be found on beaches of any tropical Indo-Pacific island. The most notable missing species were Tournefortia argentea and Suriana maritima. Tournefortia seedlings have been found occasionally on Krakatau beaches but have not survived. Suriana has not been reported, although there can be little doubt that its sea-dispersed seeds can reach the island. These two species are probably barred from the island by unsuitability of the volcanic substrate; they generally grow on coral and limestone.
The rapid colonization by sea-dispersed beach species is explainable partly by the location in the Sunda Strait between Sumatra and Java. Ocean currents there vary greatly with tides and monsoonal wind shifts, so the island receives drift from both Sumatra and Java, as well as from more distant sources. Success of some species was probably partly due to symbiosis with nitrogen-fixing bacteria; these species include not only the legumes Canavalia and Pongamia but also Casuarina .
Introduced Indo-Pacific Species, Mauritius
(Sauer 1961, 1967b )
Several Indo-Pacific shrubs and trees that are now naturalized along the Mauritius coast would probably be mistaken for natives without the historical record of their introduction.
Mauritius was uninhabited and visited only occasionally by Portuguese and Dutch ships before being temporarily colonized by the Dutch in the seventeenth century. Permanent settlement began with arrival of the French in 1715. Soon after, Mauritius began playing a leading role in the great exchanges of tropical plants between botanical gardens of European colonial powers. Its great botanical gardens at Pamplemousses and Reduit were world famous. Beach species were a very minor part of their collections.
In the mid-eighteenth century, Mauritius acquired Casuarina equisetifolia and Calophyllum inophyllum from Madagascar and Terminalia catappa from Bengal. In the early nineteenth century, Morinda citrifolia was imported from India and Desmodium umbellatum from an unspecified source. In the late nineteenth century, Pongamia pinnata was imported from the Seychelles. All of these have become naturalized as volunteers in natural coastal habitats on Mauritius, probably spreading around the island by floating seeds. Terminalia is also dispersed by the native fruit bats or flying foxes.
The story of Cocos nucifera is less clear. Coconut palms were reported on the Mauritius coast in 1598 by the first Dutch expedition. Whether they arrived on their own or were introduced by the Portuguese is unknown. In any case, coconuts are rarely found today along the Mauritius coast except where planted.
It is not immediately obvious why any of these species should have had to await human transport in order to reach Mauritius. All except Casuarina are known to be capable of long-range dispersal. Casuarina seeds survive immersion in salt water indefinitely but are not buoyant. They are believed to cross ocean barriers by rafting, particularly by floating pumice on which they have been seen germinating. Moreover, all these species were native on various other Indian Ocean islands, including the Seychelles, which lie in the same longitude as Mauritius (fig. 2).
The Seychelles were uninhabited when discovered by a British ship in 1609; wild coconut palms lined the beaches then. The archipelago remained uninhabited until colonized by French from Mauritius in the mid-eighteenth century, initially to exploit the wild coconuts. The first colonists also encountered coastal Casuarina, Calophyllum , and Terminalia groves. They were amazed at the size of the Terminalia trees, which they had known in Mauritius as saplings. Morinda and Pongamia were probably also naturally established
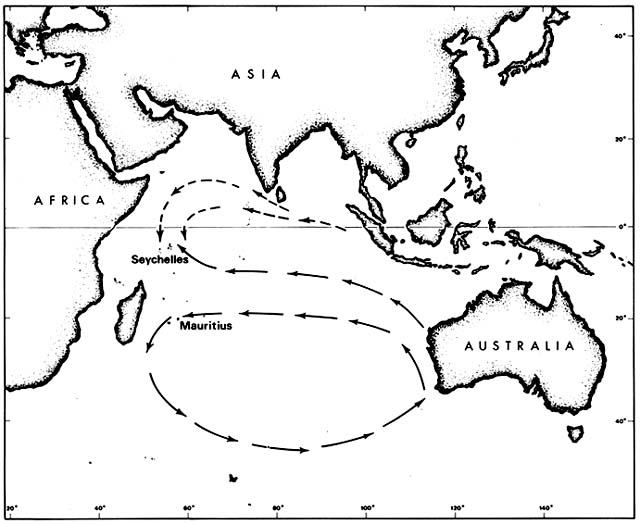
Figure 2. Prevailing Indian Ocean Currents Upstream from Seychelles and Mauritius. Although the currents
are not constant, drifting seeds of tropical beach plants are more likely to reach the Seychelles than Mauritius.
Some such species that arrived prehistorically in the Seychelles have since been artificially introduced to Mauritius.
in the Seychelles, although the first clear records are from the nineteenth century.
The rich native beach flora of the Seychelles, as compared to Mauritius, may result from the pattern of ocean currents. The Seychelles lie in the Equatorial Current downstream from Java, the rest of the East Indies, and many Pacific islands. Mauritius is far enough south to lie within the great counterclockwise circulation around the southern Indian Ocean and is thus downstream from the southern and western coasts of Australia. Such currents are variable, of course, but evidently not enough to erase different effects on dispersal.
Transfers Between Old and New Worlds
(Sauer 1982)
Some of the Indo-Pacific beach species introduced to Mauritius were also introduced through botanical gardens to the West Indies. Casuarina equisetifolia and Terminalia catappa from the East Indies were being grown in Jamaica by 1790, and Morinda citrifolia probably arrived soon after. All three were widely planted in the New World tropics during the nineteenth century, Casuarina for timber and fuel, Terminalia as a shade tree, and Morinda as a medicinal and dye plant. Herbarium specimens show that by mid-nineteenth century, all three had escaped from cultivation and were spreading on their own along seashores. Once in the region, Terminalia and Morinda were quite capable of island hopping by sea dispersal; both are recorded as having arrived in Grand Cayman, for example, as drift seeds. Casuarina and Morinda mainly colonize open seashore habitats, but Terminalia invades dense coastal thickets and overtops native trees.
Not all introduced Indo-Pacific seashore species have been so successful. Cocos nucifera, Barringtonia asiatica , and Scaevola taccada are commonly planted in the Caribbean region, often around beach houses, but are not spreading on their own.
Various Caribbean beach species have been widely planted in the Indo-Pacific region, including Mauritius, and have escaped to a few beaches, for example, spiderlily, Hymenocallis caribaea ; yellow bells, Tecoma stans ; whitewood, Tabebuia pallida ; frangipani, Plumeria spp.; and cocoplum, Chrysobalanus icaco . The cocoplum has become particularly successful on beaches of Viti Livu, Fiji. It had escaped to several beaches by 1962, and by 1984 it was extensively codominant with the native shrubs in beach ridge thickets on Viti Livu.
Anacardium occidentale , the cashew, has naturalized more extensively. Native to coastal dunes of northeastern Brazil, the cashew was introduced
by the Portuguese early in the colonial period to West and East Africa, India, and the East Indies; other people soon joined in planting the trees. The fruits are attractive to many animals, including monkeys, and the seeds are capable of at least local sea dispersal. Herbarium specimens show that by the mid-nineteenth century, the cashew was naturalized on many seashores of East and West Africa, tropical Asia, and the East Indies.
Comment
Like mangrove swamps, tropical sea beaches have a rich and very discrete flora. Few species transgress between these beaches and other kinds of habitats. There are minor exceptions, including the cases noted above when inland weeds temporarily invaded beaches after hurricanes and torrential rains. Conversely, beach species can invade adjacent inland sites when their normal competitors are removed. For example, in the Caribbean and Indian Ocean after World War II, abandoned military installations were commonly overrun by Canavalia maritima and Ipomoea pes-caprae vines.
For the outpost species, normal survival in a habitat within reach of storm waves involves perpetual micromigration, even on a stable coastline. Less extreme situations than the hurricanes discussed above include winter northerly storms and summer calms along the Mexican Gulf coast and monsoonal wind shifts on the East African coast. Beach ridge thicket species live in habitat largely controlled by a salt spray gradient and only marginally affected by storm waves. Where a coastline is progressively eroding or advancing, both outpost and thicket species may survive by local migration without any species extinctions within sites or recruitment of species from other sites.
Sea dispersal of tropical beach species has allowed them to colonize habitats that are spatially extremely discontinuous, not only on islands but on mainland coasts. Both outpost and thicket species are excluded from topographically sheltered shorelines by mangroves and inland species. Some beach species are excluded from bedrock or shingle coasts or from black sand or quartz sand beaches. Moreover, unlike mangroves, beach species need fresh water. They can survive a dry season by drawing on the fresh water lens floating in the sand above the denser salt water, but they are excluded from coasts where there is too little rain to maintain this lens.
Unlike many mangroves, the beach species produce seeds that remain dormant while afloat, with the embryos protected from seawater. As discussed in the Introduction, in two beach ridge thicket species, Tournefortia argentea and Scaevola taccada , breaking of dormancy requires a sequence of flotation in the sea, brief or long, followed by stranding and wetting by rain. Other beach species have seeds with extremely thick, hard integuments that remain impermeable during flotation for at least several months; they also
resist scarification by surf and sand. Presumably they germinate when the integument decays after long burial in moist sand.
As in the mangroves, adaptation for sea dispersal of beach species is probably not the result of selection for long-range dispersal per se, but for persistent buoyancy, since any seeds that sink at sea are eliminated as contributors to the gene pool of their species. The effectiveness of such selection is shown most dramatically by the outpost species, a dozen of which have naturally pantropical ranges. A few thicket species also have long-buoyant seeds and have achieved naturally pantropical ranges. Most thicket species have seeds that float for more moderate time spans or have fruits attractive to birds and bats, or both. It is within this latter group that recent human dispersal has permitted certain species to naturalize on beaches they had not been able to reach before.
Temperate Sea Beaches And Dunes
Mid-Atlantic Barrier Islands, United States
(Dolan et al. 1977, 1980; Leatherman 1979; Martin 1959)
A chain of barrier islands, with salt marshes and lagoons behind them, lies along much of the mid-Atlantic coast of the United States. Beaches are composed mainly of quartz sand, much more easily moved by the wind than the calcareous sand typical of tropical beaches. As a rule, dunes begin not far above the normal high water mark, leaving only a narrow, discontinuous storm beach at the top of the foreshore. This precarious habitat has a small but distinctive native herb flora. Some species are annuals, for example, sea rocket, Cakile edentula ; seaside spurge, Euphorbia polygonifolia ; and a rare beach pigweed, Amaranthus pumilus . Others are perennials, for example, beach pea, Lathyrus japonicus , and beach sandwort, Honkenya peploides . Only the Amaranthus is endemic; most are shared with beaches of the Great Lakes. Lathyrus and Honkenya are circumboreal.
The dunes form primarily under coarse rhizomatous grasses: in the north, beach grass, Ammophila breviligulata , shared with the Great Lakes and the Atlantic coast up to Newfoundland; in the south, sea oats, Uniola paniculata , shared with the Gulf of Mexico coasts as far down as Tabasco. Both are capable of virtually unlimited horizontal and vertical growth. Thriving under sand burial, they build high, steep dunes. These dune grasses grow in irregular clumps and patches, leaving many bare pockets and swales. Toward the dune front, these bare places have scattered individuals of the
same plants that colonize the storm beach. More protected swales and older dunes to the rear have a much richer flora. A few members of this flora are primarily coastal species: salt marsh cordgrass, Spartina patens ; beach plum, Prunus maritima ; bayberry, Myrica pennsylvanica ; beach heather, Hudsonia tomentosa ; and seaside goldenrod, Solidago sempervirens . These have wide coastal ranges outside the mid-Atlantic region and most also occur in scattered inland saline and sandy habitats. The bulk of the species present in the inner dune flora are primarily inland in distribution. Some are pioneers of open habitats, for example, little bluestem, Andropogon scoparius ; red cedar, Juniperus virginiana ; and poison ivy, Rhus radicans . In protected dune hollows, forests develop that have wind-shorn tops but share tree species with inland forests.
Prevailing winds are generally offshore, thus, traumatic events for the beach and dune vegetation are concentrated during storms. Occasional tropical hurricanes, with huge waves superimposed on storm surges, cause washovers and sometimes cut new inlets clear through barrier islands. Each year, 35 to 40 ordinary winter cyclones have onshore winds and waves strong enough to erode beaches. Occasionally one of these causes catastrophic erosion and washovers comparable to hurricane effects.
These barrier islands are believed to be inherently unstable and, in the long run, not under control of the vegetation. Large masses of sediment were moved landward during the great postglacial rise in sea level. About 6,000 years ago, as sea level began to stabilize at nearly its present level, waves, longshore currents, and winds working together formed barrier islands from the surplus sand. As long as the inshore zone contained sufficient sand, the islands built seaward, with the oldest, highest dunes at the rear. This progradation continued until about 2,000 years ago when the islands were much wider than now. Thereafter, net removal of sand offshore began; also blowouts and washovers pushed some sand over the islands into the lagoons. Thus, at present the islands are becoming narrower and migrating landward. As the islands become narrower, washovers are becoming more frequent, causing extensive burial by sand of the salt marsh vegetation along the lagoons. In the southern part of the region, marsh plants, including Spartina patens and Solidago sempervirens , commonly survive in the washover fans by upward regrowth. In the northern part, overwash fans are often colonized by Ammophila breviligulata , initiating a new, inner line of dunes. Remains of former inner dune forests are being exposed on some eroding foreshores.
Air photography is now being used to monitor shoreline changes over a 630-km reach of barrier islands. Transects at 100-m intervals, 6,300 in all, have been plotted spanning 15 years for the whole island chain and 30 years for much of it. Overall retreat averages 1.5 m/year, with more rapid retreat toward the northern ends of most islands and some accretion toward the southern ends. Retreating roughly 1 mile per century, changes are obvious
to residents. Inevitably, attempts to stabilize the islands are being made. A common approach is to develop continuous stands of dune-building grasses by planting, fertilizing, and watering. This commonly produces a higher, steeper foredune. With greater shelter from the foredune, vegetational succession at the rear proceeds toward an inland forest type. However, since the sand budget is not balanced, the steepened foredune is expected eventually to be undercut and washed away by storm waves, with complete washover of the islands following.
Mustang Island, Texas
In 1959, I laid out what were intended to be permanent 1-m-wide belt transects across the dunes of Mustang Island off Corpus Christi Bay. As usual on Texas barrier islands, there was a gently sloping intertidal beach, about 20 m wide. Just above normal high tide mark and at the base of the abruptly rising foredune there were a few widely scattered plants of Cakile geniculata and Amaranthus greggii , annual herbs endemic to Gulf of Mexico beaches on both sides of the tropics. The dunes were dominated by Uniola paniculata (discussed in the preceding section), joined here by the pantropical Ipomoea pes-caprae and the Caribbean Croton punctatus . On the backslope of the foredune were scattered grasses and dicot herbs, such as Spartina patens, Sporobolus virginicus, Oenothera drummondii , and Heterotheca latifolia . About 20 or 30 m behind the foredune, stabilized dunes had dense vegetation in which the outpost species were joined by Physalis viscosa, Rhynchosia americana, Ambrosia psilostachya , and a variety of other species. Nearly the whole assemblage was shared with the tropical Gulf coast. The transects were based on a government triangulation structure and were staked.
In 1961, the eye of Hurricane Carla crossed the coast close to the study site with winds of about 275 km/hr and waves reaching 7 m above ordinary high water mark. In 1962, the triangulation structure and stakes had disappeared and the transects could be only approximately relocated. Instead of being abut 20 m wide, the intertidal bare beach was now 80 to 90 m wide, extending clear through what had been the foredune zone and into the formerly stabilized dunes behind. Above a steep sand bank, freshly cut by the storm waves, the old dune vegetation appeared undamaged. At the top of the new beach and at the base of the cut bank, there were a few isolated seedlings of Sesuvium portulacastrum and Ipomoea pes-caprae and some resprouting rhizomes of Uniola and Spartina . Near the transected area, where washovers of barrier islands had occurred, seedlings of various beach outpost species were colonizing bared areas far back in the wrecked dunes, the seed presumably having ridden in on the storm waves.
Intercontinental Migration of Ammophila
(Cooper 1958; Dicken et al. 1961; Franklin and Dyrness 1973; McLaughlin and Brown 1942; Rosengren 1981; Sweet 1981)
Marram grass, Ammophila arenaria , native to western Europe, is even more vigorous as a dune builder than the eastern North American A. breviligulata (discussed above). Marram grass has been widely planted for dune stabilization. Where it has been introduced, it commonly has naturalized and spread.
On the Atlantic coast of the United States, marram grass is now naturalized locally, but has not generally displaced its native congener. On the Pacific coast, it has widely displaced a native dune grass, the circumboreal Elymus mollis , which is a relatively weak dune builder.
The amount of sand carried down western North American rivers was drastically increased during the nineteenth century by hydraulic mining, grazing, logging, and farming. Beaches near river mouths built out faster than vegetation could follow and surplus sand moved inland in great sheets and moving dunes. Starting in 1869, the active dune field that was to become San Francisco's Golden Gate Park was stabilized by planting marram grass. Soon after, the Coast Guard planted it to stabilize dunes along Humboldt Bay in northern California. Between 1910 and 1934, the Forest Service, other government agencies, and property owners planted marram grass on many dunes along the Oregon coast. Beginning in 1935, a huge dune field on the Clatsop Plains, south of the Columbia River mouth, was planted with marram grass. The eastern North American Ammophila breviligulata has also been planted on Pacific coast dune fields, but to a much lesser extent.
At the present time, Ammophila is thoroughly naturalized on innumerable stretches of the Pacific coast and has become a powerful geomorphic agent by building fairly continuous wall-like foredunes, which were not previously characteristic of this region. Sheltered behind these walls, formerly active sands have become vegetated with a mixture of native and exotic species. Prominent among these is Scotch broom, Cytisus scoparius , native to sands and sea cliffs of western Europe, which was also deliberately introduced in the late nineteenth century for sand stabilization and has now become thoroughly naturalized.
Marram grass has had a similar history in temperate Australia. Two native Australian beach grasses, Spinifex longifolius and S. hirsutus , are moderately effective sand binders, but they do not form steep, stabilized dunes as Ammophila does. Ammophila is currently replacing Spinifex hirsutus at latitudes above 33°S on many Australian beaches. Substituting stabilized linear foredunes for active transverse dune fields allows advance of woody inland
vegetation, especially Acacia spp. at the expense of various beach pioneer species.
Naturalization of Chrysanthemoides , Australia
(Gray 1976)
Chrysanthemoides monilifera is a shrubby composite native to the temperate southeastern coast of Africa, where it grows behind the storm beach on dunes and sea cliffs. The seeds are not buoyant and are probably bird dispersed. The species spreads vegetatively with vigor.
The species has been cultivated in the Sydney Botanical Garden and elsewhere in Australia since the late nineteenth century. Early in the present century, it escaped to beaches in New South Wales and on Lord Howe Island offshore. After 1950, it was much planted by the Soil Conservation Service to stabilize dunes and revegetate sand mining areas. This planting was stopped when it was found that Chrysanthemoides was invading and in places eliminating the complex native dune flora. In 1981, I saw several dense pure stands of this exotic on New South Wales beaches between 28° and 36°S latitudes, but none on any Queensland or Victoria beaches.
A reciprocal invasion of native South African coastal vegetation by introduced Australian Acacia cyclops and A. saligna is out of control (Taylor 1978).
Naturalization of Mesembryanthemum , California
(Blake 1969; Ferren et al. 1981; Moran 1950; Vivrette and Muller 1977)
The genus Mesembryanthemum s.l. (sometimes split into more than 100 genera) has thousands of species native to South Africa. Many of these are widely cultivated by fanciers of succulent plants. For example, M. edule (= Carpobrotus edulis ), native to the Cape Province and Natal, has been cultivated in Europe since the seventeenth century and in Australia and California since the nineteenth century. Although now widely naturalized there on sea beaches, it is generally recognized as an exotic that has escaped from cultivation.
Migration of a species very closely related to Mesembryanthemum edule , namely M. aequilaterum (= Carpobrotus aequilaterus ) is problematic. It is not known from South Africa and is generally considered to be native to either Australia, Chile, or California, or all three. The species was described in 1798 by Haworth from plants cultivated in England that were reported to be
"native to the country around Botany Bay." Either by oceanic drift or by transport on a ship, the species reached Chile before 1810, when it was collected at Valparaiso and named M. chilense . The first California record is from Bodega Bay in 1841. There are various plant species that apparently have naturally disjunct ranges between Chile and California, presumably due to dispersal by migratory birds. However, in this particular case, nineteenth-century human introduction appears likely. Mesembryanthemum aequilaterum is still actively spreading on the California coast. For example, at Point Dume in Los Angeles County, local residents have observed its expansion; in what was planned as a natural coastal reserve, it is spreading as a thick blanket that is overwhelming the native dune vegetation. However this species reached Chile and California, it seems that it originated in Australia along with several very closely related species that are Australian endemics. One of these, M. glaucescens (= Carpobrotus glaucescens ), appears in a water color painted at Sydney by Governor Arthur Philip about a year after the arrival of the first British colonists. The progenitor of these endemic Australian species must have come from South Africa long before, perhaps by oceanic drift.
Several other Mesembryanthemum species that are known to have been imported from South Africa have escaped from cultivation and are naturalized along the coasts of California, Baja California, and the offshore islands, for example, M. crystallinum and M. nodiflorum , which have been spreading since 1890, and M. croceum , which has been spreading since 1940.
Naturalization of Cakile , Australia and Western North America
(Barbour and Rodman 1970; Rodman 1974)
Various species of Cakile , the sea rockets, grow as extreme outpost pioneers on storm beaches. As noted in the Introduction, they have a peculiar mechanism for dividing their seeds between in situ reproduction and sea dispersal. Part of the seed is buried with the dead annual parent and part floats away, remaining viable for at least 10 weeks in seawater, but germinates only after being stranded and rained upon.
Cakile edentula is native to the Atlantic coast of North American from Labrador to Florida and the shores of the Great Lakes. In Australia, it appeared near Melbourne before 1863 and near Sydney by 1870, supposedly brought in sand ballast by an American sealing ship. It soon spread on its own to all the southeastern Australian states, including Tasmania, and to Lord Howe Island (fig. 3). By 1922 it was moving north along the temperate Queensland coast and by 1960 reached Heron Island on the Tropic of Capricorn. In 1981, I did not find it on any tropical Queensland beaches. Moving
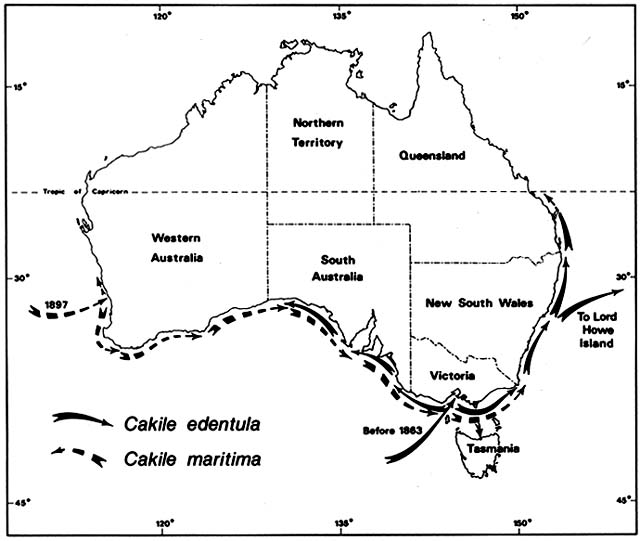
Figure 3. Naturalization of Cakile on Australian Beaches. Temperate beach species have repeatedly found open
niches distant from their native ranges following introduction by human agency. Two species of sea rockets that
arrived in Australia in the late nineteenth century spread by sea dispersal from their entry points along Australian
beaches, often advancing at a rate faster than 50 km/year until reaching their climatic limits. These grow closer to the
sea than native beach plants.
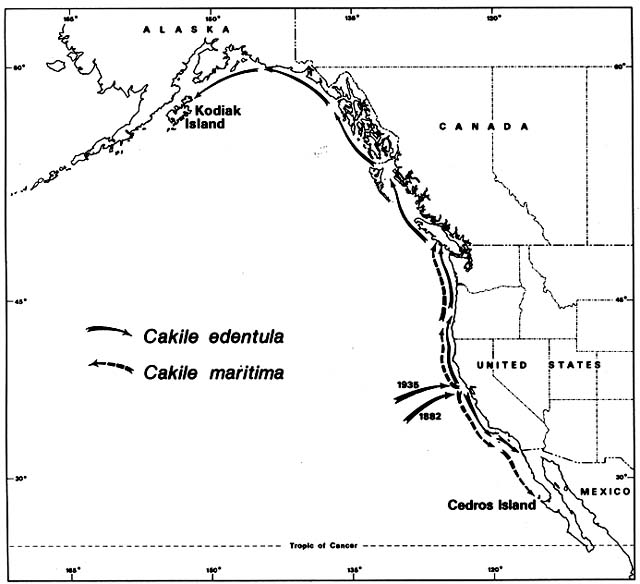
Figure 4. Naturalization of Cakile on Western North American Beaches. The sea rockets that invaded Australian
beaches were also accidentally introduced to the California coast. Cakile edentula from the Atlantic coast of North
America arrived first in San Francisco Bay. It then spread rapidly northward and southward. Before its migrational
history was investigated, some botanists mistook it for a native. Cakile maritima arrived much more recently from
Europe and has always been recognized as introduced.
westward from Melbourne along the south coast, C. edentula met and mixed with another invading sea rocket, C. maritima , which was moving in the opposite direction.
Cakile maritima is native to the Mediterranean and Black seas and to the Atlantic coasts of Europe as far north as southern Scandinavia and Scotland. It arrived at the port of Fremantle, Western Australia, by 1897. By 1963, it was ubiquitous on Western Australia beaches from 31°S southward; I found it on every one of 18 beaches studied in this region. Moving eastward along the south coast, C. maritima had entered South Australia by 1918 and Victoria by 1922. For a while, C. maritima and C. edentula shared the coasts of South Australia and Victoria, but C. martima now has sole possession of these Mediterranean type, dry summer coasts. Cakile edentula remains dominant along the temperate east coasts of New South Wales and Queensland, where summers are wetter.
On the Pacific coast of North America, the same sea rockets have immigrated in a pattern remarkably parallel to that in Australia. Again, Cakile edentula arrived first (fig. 4); the earliest record was on the Berkeley shore of San Francisco Bay in 1882. By 1891 it was common around the Bay, and outside the Golden Gate it had moved 30 km south to Half Moon Bay. By 1906 it had arrived at San Diego, where its southward expansion ended. By 1952 it was on the Channel Islands off the coast of southern California. Meanwhile, moving northward from San Francisco, it arrived in Oregon by 1901, Washington by 1907, British Columbia by 1909, and Kodiak Island off Alaska by 1931. The northward expansion had averaged 65 km/year over a 50-year period.
Local botanists, aware of the rapid spread of Cakile edentula , recognized it as an immigrant. However, the then dominant Harvard taxonomist, M. L. Fernald, temporarily confused the story by suggesting the Pacific coast populations belonged to a native endemic variety, which he named C. edentula var. californica . He postulated a formerly continuous boreal range, which became disjunct during the Pleistocene, a concept closely related to his nunatak hypothesis for other North American disjuncts. This variety is no longer considered distinguishable from the species proper.
The European Cakile maritima arrived in California so recently that no botanists have mistaken it for a native. It was first recorded in 1935 on a popular beach 25 km north of San Francisco. It spread almost as fast as C. edentula , averaging about 50 km/year in both directions. Northward it reached British Columbia by 1951, and southward it reached Cedros Island off the coast of Mexico by 1963. As in Australia, C. maritima almost completely replaced C. edentula in the region of Mediterranean type, dry summer climate. From Oregon northward, in areas with summer rain, C. edentula has remained predominant.
In both Australia and western North America, if only the present, static distributions of the two sea rockets were known, their nearly discrete ranges would appear to be directly controlled by climate. However, the actual history shows that Cakile edentula was quite successful outside its present climatic limits before the arrival of C. maritima . The outpost zone on a storm beach would at first glance seem one of the last places in the world where competitive displacement of one species by another would take place. Only a small part of the area is occupied by plants and for only part of the year. Nevertheless, if we recognize that the beach is not homogeneous, that there may be very limited safe sites for sea rocket establishment, and that the expanse of bare sand may be an uninhabitable wasteland for both species, we can visualize fierce competition in spite of low population density.
Comment
Temperate beach plants, like tropical ones, are relatively indifferent to rainfall gradients provided there is enough rain to maintain a freshwater supply under the sand. The greater seasonality of temperate coasts probably affects beach plants mainly via changing wave energy. Lacking fringing coral reefs, temperate beaches commonly have an annual cycle of strong erosion and accretion. Also, temperate zone beaches are generally composed mainly of quartz sand, which is more restricted in distribution in the tropics. Quartz sand is transported by longshore currents and is easily moved by the wind when dry, unlike calcareous sand, which is of local origin and normally immobile under the wind. Thus, many temperate barrier beach and dune systems are relatively extensive and dynamic. In spite of this, their floras are relatively poor in primarily coastal species except in South Africa and Australia, regions that are also phenomenally rich in primarily inland floras on ancient sand plains.
The primarily coastal, drift-dispersed outpost species have less broad and disjunct ranges in temperate than in tropical regions. A few that now have disjunct circumboreal ranges may be relicts from ancient distributions along former seaways. Similarly, the eastern North American beach species that now have disjunct ranges on the Great Lakes and Atlantic coast may have colonized the interior in early postglacial time when a marine embayment provided a continuous route, subsequently broken by isostatic uplift (Rodman 1974). Clearly, this species group is dependent not on salt water or salt spray but on waves, wind, and moving sand to create its habitat.
Like tropical beach pioneers, temperate outpost species need drift dispersal to occupy a dynamic and discontinuous habitat, but the temperate species have seeds that are buoyant for a shorter time. Perhaps this is because seeds
floating longer distances in temperate latitudes would have less chance of survival because the prevailing currents run north and south along the coasts rather than east and west through island stepping stones. The examples noted above of naturalization and spread following artificial introduction to distant regions demonstrate that the species had been unable to reach parts of their potential areas by natural long-range dispersal. They also demonstrate these species' capacity for rapid stepwise migration along a coastline and to offshore islands. Many similar cases, not discussed above, are known, for example, naturalization of Artemisia stelleriana and Carex kobomugi , native to northeastern Asia, on eastern North American beaches, and naturalization of Rumex frutescens , native to temperate South America, on British and Danish dunes. Some of these naturalized exotics have found an unoccupied niche closer to the sea than the native beach vegetation; others have invaded and displaced native dune species.
Salt Marshes
Limited Successions in Coastal Marshes, Britain
(Chapman 1959, 1976; Ranwell 1972; Tansley 1947)
Salt marshes have long been a favorite object of ecological studies in Britain; their history and dynamics do not support a model of succession leading to climax vegetation. Tansley (1947) concluded long ago that there is no good evidence that salt marshes can develop into nonmaritime vegetation by the mere accumulation of silt or humus.
A time-lapse study of a complex of Norfolk marshes over 25 years showed that in local areas where accretion of sediment happened to outrun regional subsidence, there was a predictable sequence of plant species. Commonly the first colonist of accreting mudflats, just above low tide mark, was a creeping monocot, Zostera noltii , followed in sequence by Salicornia europea, Aster tripolium, and Spartina townsendii s.l. The last is a rank, rhizomatous grass that is an exceptionally effective mud binder, at least temporarily; it is of hybrid origin and has only existed since about 1870.
There are many ways in which such autogenic salt marsh succession can be halted or reversed. Sedimentation may be offset by compaction so that there is little rise in marsh level. Accretion may shift rapidly to erosion because of a change in sediment supply or with strong onshore winds. Established marsh vegetation may be killed by deposition of flotsam and by
formation of stagnant pools and hypersaline flats. Also, marshes undergo cyclical deposition and dissection along tidal creeks. Steep banks inevitably develop along the creeks by a combination of deposition of natural levees under overbank flow on flood tides and scouring of channels on ebb tides. Eventually undercutting and collapse of banks starts the cycle over.
Limited Successions in Coastal Marshes, California
(Pestrong 1965; Warme 1971)
A similar cycle of bank buildup and collapse occurs along tidal creeks on San Francisco Bay mudflats. There is a correlated cycle of two dominant perennials, a pickleweed, Salicornia virginica , which grows on the natural levees, and a tall cordgrass, Spartina foliosa , which grows in the tidal creek channels, where it may endure 20 hr of submergence daily. As sediment is deposited on the natural levees at flood tides and scoured from the bank bases at ebb tides, banks become oversteepened and collapse. Salicornia does not survive submergence on the slump blocks but is replaced by Spartina , which traps sediment until bank buildup allows recolonization by Salicornia .
Salt marsh dynamics are more complex on Mugu Lagoon in southern California. Topographic maps spanning more than 100 years show that the eastern end of the lagoon, about 1.5 km long and as much as 0.5 km wide, has been mostly converted from open water to intertidal marsh. Recent measurements of staked areas show marsh advance at rates as high as 1 m/year. The marsh plants show fairly regular zonation, part of which can be fitted to an autogenic succession model. The subtidal lagoon has beds of eelgrass, Zostera marina , and ditchgrass, Ruppia maritima , which help trap sediment and shells and upgrade the substrate to the low tide mark. There is a barren zone above low tide mark where aggradation must be independent of plants. Spartina foliosa , which occurs at this level in other California marshes, is present here in only small, isolated patches. Above this bare zone, the lower fringe of marsh vegetation is dominated by annual Salicornia europea and perennial Salicornia virginica . Above 1.5 m above low tide mark, these chenopods are joined by 10 or so other species, mostly perennials, including other chenopods. These continue to the upper reach of highest tides, where they are joined by Arthrocnemum subterminale as a dominant. The vegetation of the middle and upper marsh is generally very thick and evidently capable of aiding sedimentation. However, rather than proceeding toward climax upland vegetation, older parts of the marsh become hypersaline areas, with salinities over twice that of seawater during the rainless summers, where expanding bare salt pans develop.
The Mugu marsh is strongly affected by various geomorphic processes that are not under control of autogenic vegetational succession. The marsh lies at the mouth of Calleguas Creek, normally a minor intermittent stream. During exceptionally heavy rains, the creek pours huge quantities of sand into the lagoon. The lagoon and marsh lie behind a barrier beach, the last of a series that formed sequentially offshore on an uplifted fault block since postglacial sea level stabilized. Sand being brought down the coast by longshore currents enters the lagoon through the tidal inlet. Also, during winter storms, ocean sand is poured over the barrier beach by washovers. A deep submarine canyon, with its headwall in unconsolidated sediments, has cut back almost to the mouth of the tidal inlet. It is on the verge of capturing the longshore sand supply and reversing the whole process of marsh development.
Primary Succession on a Mediterranean Lagoon, France
(Braun-Blanquet et al. 1958)
Soon after hearing about Clementsian succession theory, Braun-Blanquet initiated a time-lapse study to test the postulated relationship between salt marsh zonation and primary succession. The site chosen was on an aggrading tidal lagoon shore near Montpellier; initially it included a cove 30 m wide behind a narrow sand spit. The site was mapped nine times over a 43-year span; the study ended when the site was artificially disturbed. Seaweeds and marine angiosperms, for example, Ruppia maritima and Zostera marina , were present below low tide mark but were not mapped.
When the study began in 1915, the sand spit was mainly bare except for a narrow fringe near the high tide mark of annual chenopods, such as Salicornia europea, Suaeda maritima, and Salsola soda, and a few common reeds, such as Phragmites australis , that had taken root from stranded rhizomes. Along the shore of the lagoon was a belt a few meters wide dominated by perennial chenopods, such as Arthrocnemum spp. and Halimione portulacoides ; between them and higher ground was another narrow belt dominated by a rush, Juncus maritimus . Within its original area of about 100 m2 , the site also had 19 other less abundant species, all halophytes, such as sea lavender, Limonium vulgare , and sea aster, Aster tripolium .
By the end of the study in 1958, wave-deposited sand and shells had caused the shoreline at low tide mark to advance about 50 m, adding over 1,000 m2 to the study area. Fresh shell ridges had cut off two depressions within the former cove; the shallower one was covered with perennial Arthrocnemum spp.; the deeper one retained a pool of open water inside an Arthrocnemum ring. The thin fringe of annual chenopods and reeds had moved forward with the advancing shoreline. Behind it, better-drained sand
and shell areas were dominated by a steadily expanding Halimione colony. At the rear of the study area, the initial Juncus zone had remained relatively stationary, but had moved slightly seaward into the Arthrocnemum -dominated area and was in turn being invaded at the rear by Spartina versicolor , a tall grass growing in both saltwater and freshwater marshes. At the same time, the better drained Halimione -dominated areas were beginning to be invaded by tall Juncus acutus and Limonium vulgare .
Interrupting the steady seaward advances were setbacks, when strong mistral winds and waves opened up patches of established vegetation for recolonization by the pioneer fringe species. Also during the rainy season, ephemeral populations of weedy annuals appeared on the tops of shell ridges, including Polygonum aviculare, Stellaria media, Galium aparine, Sonchus oleraceus, and Senecio vulgaris .
Measurements of soil variables and plant osmotic pressures suggested that species distributions were largely controlled by soil moisture, salinity, and aeration. Halimione occupied sites with the greatest contrasts between rainy and dry season soil conditions and had the greatest range of osmotic pressure, from less than 25 to more than 60 atm.
As for testing the model of primary succession, the results are equivocal. The results generally agree with the model in showing a tendency for the vegetation zones to advance as sediment was deposited on the shore. The results do not prove the crucial assumption of a Clementsian autogenic succession model, namely, that changes in the ecosystem are directional and under control of the vegetation. In this case, the changes may have been allogenic, that is, due to surplus shell supply, leaching of sediments by rain, or other processes not under vegetational control. The study site was chosen on an aggrading shore. If vegetation changes passively follow shoreline changes, the zones may be static or retreating on other shorelines.
Natural Colonization of Artificial Inland Salt Marshes,
Europe and North America
(Ogle 1981; Simon 1958; Zumph and Rebmann 1932)
As a waste product of salt mining operations, saline ponds were created 40 km south of Berlin in 1907. Within 3 years the ponds were colonized by halophytes, including Triglochin maritima, Glaux maritima, and Plantago maritima . The botanists who studied the colonization believed the seed had been brought from seacoast marshes by waterfowl; it is also possible the seed had been transported from other saline inland places, although none were known closer than 30 km.
Commercial exploitation of a salt dome at Müllhausen, Alsace, began in
1904. Salt ponds created by slurry dumping were visited by migratory waterfowl. By 1954, the ponds had been colonized by halophytes, including Chenopodium botryodes ; the nearest seed source known for some of the species was 500 km away.
In western Virginia, 400 km from tidewater, pumping of brine wells since the late nineteenth century has created a habitat for various salt marsh species, for example, Salicornia europea and Eleocharis parvula . A new species, Scirpus robustus , was found in 1977; its population expanded rapidly in the next few years. All the species are believed to be dispersed by birds.
Naturalized Exotics, California
(Robbins 1940)
In the late nineteenth century, E. W. Hilgard, head of the California College of Agriculture, imported seed of various species as possible forage plants for alkali lands. From Australia, Baron Ferdinand von Mueller sent seed of several species of Atriplex , including A. semibaccata , which has grown successfully at the Tulare experiment station and from there has been widely distributed in California. The first escape to a tidal marsh was reported by botanist Alice Eastwood in 1901. She found it flourishing in a marsh north of San Francisco. By 1935, it was widespread along the mainland coast and on offshore islands. Today it is extremely common, even dominant, in the upper parts of coastal marshes and other saline habitats.
Several other salt marsh species arrived in California by unrecorded means, an obvious possibility being in sand used for ship ballast. Brass buttons, Cotula coronopifolia , native to South Africa, was found in San Francisco Bay in the Early 1850s and has since become common along the whole mainland coast and on offshore islands. Two grasses native to western Europe and the Mediterranean, Monerma cylindrica and Parapholis incurva , were first recorded near San Diego about 1900 and have since spread far northward along the California coast; Parapholis has also reached offshore islands. A European chenopod, Bassia hyssopifolia , may have arrived in California indirectly via Argentina as a contaminant in alfalfa seed. It was naturalizing in inland alkali and saline habitats by 1920; at present it is invading coastal marshes near Santa Barbara.
Comment
A few exceptional plant species, including Phragmites australis and Cotula coronopifolia are shared between saline, brackish, and freshwater marshes, but
on the whole, salt marshes have a very discrete flora. Most salt marsh species are confined either to coastal marshes subject to tidal flooding or to interior marshes that may be only seasonally flooded, but a few are capable of growing in both. For species capable of coping with the extreme osmotic pressures of these habitats, the reward is escape from competition from ordinary nonhalophytes.
In many salt marshes, plant species can survive indefinitely with only micromigrations and no capacity for long-range dispersal. There are cases of salt marsh species that have evolved as localized endemics, for example, several Atriplex species in the San Joaquin Valley of California. However, many salt marsh species have very wide and disjunct distributions. Some species are known to be capable of long-range dispersal by ocean currents, such as Salicornia spp., which have seeds capable of floating for months (Ranwell 1972). Such dispersal may explain some grand disjunctions, such as the transatlantic ranges of Salicornia europea and S. virginica . It cannot explain the presence of these two species on both Atlantic and Pacific coasts of North America. For many such disjunctions, particularly involving inland populations, bird dispersal seems to be the best explanation. Disjunct salt spring floras in the interior of Europe have sometimes been attributed to fragmentation of populations that were continuous during Pleistocene arid periods, but Aellen (1958) argued that known cases of recent bird dispersal obviate the need for such explanation.
The cases noted above of naturalization following long-range human dispersal involved disjunctions that birds were unlikely to span.
Freshwater Aquatic Habitats
Vernal Pools, California
(Griggs 1981; Jain 1976; Keeley and Keeley 1979; Thorne and Lathrop 1969)
Seasonal lakes and pools in California grasslands are tiny relicts of native herbaceous vegetation in a sea of European annuals. They harbor over 200 species of flowering plants in about 50 genera, many of which are found in no other habitat. Most are in the Central Valley on Pleistocene alluvium underlain by an impervious hardpan or claypan. Some are on ancient lateritic soils on mesas formed by basalt flows.
Under the present Mediterranean climate, habitat conditions vary drastically with the seasons and from year to year. Most of the species are annuals.
These may bank large quantities of seed in the soil in wet years; the seed may remain dormant for years or decades. For example, in one pool a rare grass, Orcuttia pilosa , grew abundantly one year, disappeared completely for 4 years, and reappeared in abundance the fifth year, when the soil still contained 50 dormant seeds for every one that germinated. Orcuttia seed germinates only when infested with certain fungi, which are abundant only when the pond is flooded. Orcuttia seedlings start life as submerged aquatics, then develop floating leaves and become tall emergents when the water level drops; when the pool dries, flowering begins within days.
Annual censuses of the flora of a vernal marsh on the Mesa de Colorado in southern California showed species appearing that had not been seen in previous years, for example, Potamogeton pusillus and Elatine californica in 1968 and Orcuttia californica and Myosurus minimus in 1969. Whether these were new immigrants or from dormant seed is not known.
Some vernal pool species are endemic to California or at least to the far western United States, including several congeners in Orcuttia, Limnanthes, Elatine , and Downingia . Some are disjunct between temperate North and South America, for example, Deschampsia danthonioides, Elatine chilensis , and Lilaea scilloides . Some are shared between North America and Eurasia, for example, Crassula aquatica, Eleocharis palustris, Callitriche palustris, Potamogeton pusillus , and Myosurus minimus . A few range from the Old to the New World and from the northern to the southern hemisphere, such as Juncus bufonis and Lemna minor .
The likelihood of long-range bird dispersal of these species is discussed at the end of this chapter. Local bird dispersal of recently introduced exotics appears likely in a few cases. Naturalization of the South African Cotula coronopifolia in California salt marshes was noted in the preceding chapter. This species has also spread inland around vernal pools, including those on the Mesa de Colorado. Another annual, Lythrum tribracteatum , native to southern Europe and the Near East appeared in California in 1930 in a vernal pool near Sacramento. By 1948 it had spread 200 miles southeast in the Central Valley to a lake that dries each year; other widely scattered populations have since appeared in seasonally flooded central California sites. In 1978, Aponogeton distachyus , the Cape pondweed from South Africa, was discovered in vernal pools on Kearny Mesa in San Diego. The species is widely planted in ponds as an ornamental; it has also escaped to natural freshwater habitats in northwestern Europe, western South America, and southern Australia.
Many California vernal pools have quite recently been destroyed by conversion to rice paddies or drainage for other crops. Preserving a few relicts of this increasingly disjunct habitat type is currently an issue in California.
Intercontinental Migrations of Elodea and Hydrilla
(Mitchell 1977; Sculthorpe 1967; Yeo and McHenry 1977)
Two closely related waterweeds have invaded streams in various continents following long-range human introduction. Both are dioecious perennial herbs, rooting in sediment and with the submerged stems bearing whorled foliage like a bottlebrush. Flowers of the pistillate plants open at the water surface to receive floating pollen. Both species are easily propagated vegetatively and have become favorite freshwater aquarium plants.
Elodea canadensis , native to lakes and slow streams of North America, arrived in the British Isles in the 1840s, supposedly imported accidentally with American water lily rhizomes. Although only female plants were present initially, the species spread rapidly by human and perhaps bird dispersal. It was widely naturalized in the British Isles and on the continent by 1879, when a male clone arrived and seed production began. For some years, Elodea proliferated excessively, impeding water flow and boat traffic; control efforts were futile because of rapid regeneration from fragments of the plants. After a few years, populations generally declined spontaneously, possibly due to buildup of predators. Since 1900, Elodea has subsided to become merely another member of Europe's freshwater flora.
In Australia, Elodea escaped by 1960; its spread is unclear because for a while it was confused with the native Hydrilla . It has become a common weed of rivers and ditches in the New South Wales—Victoria border region.
Hydrilla verticillata is regarded as native in temperate and tropical eastern and northern Australia, the East Indies, southeast Asia, and various western Pacific and Indian Ocean islands. It can reach the surface and establish a dense canopy in water as deep as 15 m. Introduced to North America in the aquarium trade, it was first noticed growing wild in Florida in 1958; by 1967 it occupied tens of thousands of acres of waterways in Florida and neighboring states. Only male plants are present there, but the species is dispersed vegetatively, probably by waterfowl and trailered boats. Drag lines haul it out by the ton to keep waterways open, but it promptly grows back.
In California, Hydrilla was found to have become naturalized in the Sacramento Valley in 1976; it has since spread widely in the state. It has become a serious problem in canals and irrigation ditches in southern California. In 1985, the state Department of Fish and Game authorized the Hydrilla Control Research Project to stock all canals of the Imperial and Coachella valleys with 78,000 Amur River grass carp. A mutant triploid strain of the fish is being used, which is expected to live about 5 years and die without reproducing.
Hydrilla has escaped in many other warm regions in both natural and artificial lakes and streams. Gatun Lake, Panama, has a flourishing infestation.
A similar case of naturalization following long-range dispersal through aquaria involves Lemna minuscula , a tiny floating aquatic native to the New World; it has apparently been spread by bird dispersal through European lakes and streams (Landolt 1979).
Intercontinental Migrations of Eichhornia and Alternanthera
(Bock 1968, Hockley 1974; Maddox et al. 1971; Mitchell 1977; Russell 1942; Varshney and Rzoska 1976; Vietmeyer 1975)
Water hyacinth, Eichhornia crassipes , and alligator weed, Alternanthera philoxeroides , are native to the great river systems of eastern South America from the Orinoco to the Paraná. Both root in shallow water along stream banks and may extend out over deep, quiet water as floating mats. Both are capable of rapid vegetative reproduction. Water hyacinth has nitrogen-fixing bacteria within the leaf and grows well in clean water. Alligator weed grows best in nutrient-enriched water; it can tolerate brackish water better than water hyacinth. In their native habitats, neither species is especially abundant or conspicuous. When introduced abroad, both have commonly produced prodigious biomass, presumably because of release from natural predators.
At home or abroad, both species survive devastating floods. When the bulk of the floating mats are carried off, survivors in backwaters remain. Water hyacinth also stores dormant seed in the mud, which remains viable for at least 15 years. Outside its native area, alligator weed seems to reproduce only vegetatively; although it has bisexual flowers, a clone may be incapable of self-fertilization.
The long-range migrations of water hyacinth are easily explained by deliberate human transport; it produces beautiful flowers and shiny foliage. Alligator weed is an unattractive plant with no known record of deliberate planting. In spite of their differences, the two have had rather similar macro-migrations.
Water hyacinth was introduced from the Orinoco region to New Orleans, Louisiana, in 1884 and spread rapidly as a planted ornamental in pools and ponds all over the southeastern United States. Alligator weed arrived by unknown means in Florida by 1894 and in Louisiana by 1897. These two exotics have profoundly changed the geomorphic processes of the Mississippi Delta and other southeastern waterways. They are more indifferent to water depths than the native aquatics and produce much greater biomass. Bottom sediments under masses of these species are much higher in organic content
and nutrients and lower in oxygen; the whole economy of the water bodies is affected. Moreover, the result is not permanent filling of bayous and marshes with organic sediment that would initiate autogenic succession; the accumulation of weeds and sediment is eventually ripped out by floods.
Expensive efforts to control these species by mechanical or chemical attack have had only brief effect. Alligator weed is exceptionally tolerant of herbicides; herbicides kill water hyacinth plants but not the buried seed. Since 1960, several insect predators found on these weeds in South America have been released in the southeastern United States and are evidently having some effect.
Relatively minor colonies of both species have become established in California. Starting in 1904, water hyacinth spread gradually along the Sacramento and San Joaquin rivers and their tributaries. It has also established a few colonies in southern California, but there are no extensive suitable habitats there. Since 1965, alligator weed has colonized some artificial reservoirs and effluent ponds in southern California. Release of insect predators has been tried; ironically, the weed populations are so small and localized that the insect predators cannot maintain viable populations.
In Australia, water hyacinth has been widely planted since 1894. In New South Wales, it escaped to become a temporary nuisance along some rivers but has been nearly eliminated since 1935. In Queensland near the Tropic of Capricorn, construction of a barrage in 1970 on the Fitzroy River estuary greatly encouraged water hyacinth by stopping incursions of tidal seawater and flushing by freshwater floods. By 1973 the lower Fitzroy was covered with water hyacinth for over 50 km above the barrage. A costly spray and cutting program was followed in 1975 by release of a South American weevil obtained from a biological control program used in the southeastern United States. Alligator weed arrived in New South Wales by 1945, being first noticed on heaps of ship's ballast dumped near Newcastle. It has subsequently colonized about 10 localities, mainly in irrigation canals and rivers with sewage effluent.
In Java, water hyacinth was grown in the Buitenzorg Botanical Garden in 1894 and soon escaped to streams flowing through the garden. In Bengal, it was introduced from Australia in 1897. By shortly after 1900 it was being widely planted in Ceylon, Malaya, Siam, Indo-China, and China; its spread was partly in botanical gardens, largely in private hands. Its flowers were used as temple offerings, and it was planted in farm ponds to produce pig fodder, feed fish, and provide green manure. It has escaped to the Ganges, Mekong, and other rivers where it interferes with navigation and aggravates floods during cyclone passages. It chokes many of the canals in Bangkok. The alligator weed is rather uncommon in the East Indian—Southeast Asian region, although it arrived in Java by 1895 and has reached the mainland.
In Africa, the earliest available record of water hyacinth is from El Gizeh near Cairo, where it was growing in ditches and ponds in 1930. Evidently the lower Nile region offered little suitable habitat because the great invasion came by another route. In 1954, water hyacinth escaped from ornamental plantings in the Belgian Congo; it rapidly exploded into a major pest, interfering with navigation on the Congo River. In 1957, it was discovered in a remote part of the Sudan 300 km upriver from Khartoum on the White Nile. The watershed between the Congo and White Nile is narrow; perhaps cranes or other water birds carried the seeds across. In 1958, a survey found that the species was established along hundreds of kilometers of the White Nile; by 1962, it extended from Juba to the Gebel Aulia dam near Khartoum, a huge expanse bracketing the Sudd region. The Sudd is an enormous freshwater marsh, dominated by emergent aquatics, such as Cyperus papyrus and various species of Typha and Phragmites . Maintaining navigation channels through this morass had required heroic efforts; invasion by water hyacinth frustrated these efforts and, by raising the water level of the marsh and increasing evaporation, reduced the whole flow of the Nile. Work was begun on a 350-km-long canal to bypass the Sudd and increase the Nile flow, but has been halted by a long civil war.
Comment
Freshwater aquatic habitats harbor a rich and discrete flora. Whole genera and even families have evolved in these habitats. Monocots are especially well represented, but there are a variety of dicots and some gymnosperms. Growth forms range from the tiniest of herbs to great swamp trees. As noted in the preceding chapter, only a very few species are shared between freshwater, brackish, and salt marshes.
Geographic patterns of species are heterogeneous, but in general, microdistributions are sharply limited by water depth and other local variables while gross ranges are diffuse and disjunct. In the long run, survival of disjunct populations in dynamic habitats requires constant colonization. Local populations are subject to extinction by both catastrophic habitat change and by autogenic succession in low-energy habitats. In the classic theory of primary succession, Kerner von Marilaun (1863) postulated how a lake becomes a marsh and a marsh becomes a meadow.
Disjunctions in ranges of freshwater aquatics are so great that they have sometimes raised doubts about being caused by natural dispersal. Scirpus californicus , for example, has a highly disjunct range from California to Patagonia, Easter Island, and Hawaii. Heyerdahl (1971) suggested that this reed had been planted in the crater lake on Easter Island by prehistoric South American Indian voyagers who arrived on balsa rafts made from the reed. Howevever, growing in the same Easter Island lake is a species of no known
use, Palygonum acuminatum , which also has a widely disjunct range in the Americas. Heiser (1978a ) argued that bird dispersal of both species was more likely. Study of fossil deposits in Easter Island lake may determine when Scirpus arrived on the island.
Wind dispersal may be responsible for moderately wide disjunctions in some species, such as Senecio spp., and for extremely wide ones in others, such as the cosmopolitan cattails, Typha spp., and the common reed, Phragmites australis .
For most freshwater aquatics, migratory birds appear to be the most important agents of long-range dispersal. Ridley (1930) summarized a wealth of evidence that ducks and other water and shorebirds disperse aquatic plants. Some of the evidence is circumstantial, for example, colonization of isolated ponds and marshes that are visited by waterfowl and lack of colonization of those that are not visited. Much of the evidence, however, is quite direct, including many cases where shot waterfowl had viable seeds and vegetative parts in mud on their feet, adhering to their plumage, or carried internally. More recently, deVlaming and Proctor (1968) fed captive waterfowl seeds of 23 species of aquatic or semiaquatic plants. They found that viable seeds of most species were passed by killdeer or ducks or both after 20 hr or more, long enough for the birds to fly over 1500 km. Viable seeds of Potamogeton pectinatus were retained for 75 hr by both killdeer and mallards, and viable seeds of Eleocharis quadrangulata were retained 120 hr by killdeer.
Some wide disjunctions of aquatic plants fit neatly within major flyways of migratory waterfowl. The southern water hemp, Amaranthus australis , has its main populations in the Everglades and other marshes of the southeastern United States. Its seeds, ripening in the fall, are a major food of migratory birds taking off southward over the Gulf of Mexico and the Caribbean. Distribution of this species south of the United States is extremely disjunct, always in freshwater lakes and marshes: five localities in five different states in Mexico, seven localities in four West Indian islands, one lake in Venezuela, several rivers in the Guianas, and one locality in southern Brazil, which is 4,000 km from the closest Guiana locality but on the same flyway (Sauer 1972). Kloot (1984) noted that nearly all the plants shared between the pre-European flora of temperate South Australia and other continents, mainly Eurasia, are aquatic or marsh species that presumably were introduced from the northern hemisphere by migratory waterfowl.
In North America, slough grass, Beckmannia syzigachne , has evidently been dispersed northward by waterfowl. It has a disjunct but fairly coherent range in marshes of the north central and western United States and in the Canadian prairies, where its seed heads are fed upon heavily by waterfowl moving up the Central Flyway. Farther north its distribution is sporadic. In an intensive study of the arctic Mackenzie River Delta, Gill (1974) found Beckmannia only on lakes frequented by waterfowl and, on those lakes, only in places with especially heavy concentrations of waterfowl.
East—west disjunctions of aquatics between North America and Eurasia are less obviously related to flyways, but there are many records of birds breeding in the Nearctic but wintering in Eurasia and birds breeding in the Palearctic but wintering in the Americas. An example of the latter is the Eurasian widgeon, which breeds in the Palearctic from Iceland to Siberia but has been recorded as a casual visitor over much of North America (Edgell 1984).
The same species of Beckmannia discussed above is found in eastern Asia, the exchange presumably via Alaskan waterfowl breeding grounds. Heslop-Harrison (1953) suggested a similar relay of a number of aquatic plant species shared between eastern North America and Britain; waterfowl from both regions converge on the same summer breeding grounds in Greenland.
The cases of recent naturalization following human dispersal show that, however great the radius of natural aquatic plant dispersal, it was not global.
Riparian Habitats
Mackenzie Delta, Canada
(Gill 1973a,b )
In its great alluvial delta, the complex streams of the Mackenzie River constantly erode banks and destroy old vegetation on the outside of their shifting bends. At the same time, they create new ground for plant colonization on point bars deposited on the inside of their shifting bends. The details of erosion and deposition are complicated by freezing and thawing of the river and of the ground above permafrost and by flooding and ebbing of the river. However, accreting point bars have a fairly consistent sequence of colonization. The first pioneers are typically a nonseed plant, Equisetum , and Salix alaxensis , both capable of surviving regular flooding and rapid sediment deposit. After the site builds up above normal flood level, about 3 to 4 years later, these are joined by seedlings of Populus balsamifera , which for a while is the sole dominant tree. Under the canopy of this poplar, Alnus crispa and several Salix spp. form a shrub layer. The herb layer includes Hedysarum alpinum and several Arctostaphylos and Artemisia spp. The Alnus and Hedysarum have symbiotic nitrogen-fixing bacteria. Eventually, on the uppermost natural levee surface, which is only rarely flooded, Picea glauca invades and becomes the sole dominant as Populus dies out. The other pioneers remain in the understory of the spruce forest and are joined by many shade-tolerant herbs.
An almost identical succession is reported along accreting banks of Alaska floodplains (Van Cleve and Viereck 1981).
Meramec River, Missouri
(Dietz 1952; Sauer 1952)
The Missouri Botanical Garden Arboretum at Gray Summit is largely covered with an Ozark oak—hickory forest, but the Meramec River floodplain has a different kind of vegetation. Every spring the Meramec rises over its banks and changes the topography of its floodplain by erosion and deposition. Old maps and air photographs from eight different time levels over a 100-year span showed that the floodplain vegetation was generally less than 30 years old before being recycled. Pioneer colonization was dominated by Salix interior and other willows. Willow seed floating down the river on the spring flood is viable for only a few days and becomes established only if stranded on fresh alluvium while the flood is ebbing. In the area studied, willow seedlings had formed new rows in only 4 of the preceding 10 years. After 1 year, willows are too deeply rooted to be easily dislodged by the next flood. On accreting sandbars, older willow thickets are invaded by Populus deltoides, Ulmus fulva, U. americana , and Acer saccharinum , which soon become dominant. Other common riparian trees are Platanus occidentalis and Celtis occidentalis . All these trees have seeds dispersed by wind and water.
In addition to the riparian trees, other growth forms colonize sites opened by the river. Sunny sand and gravel bars have clones of switchgrass, Panicum virgatum , a coarse perennial with creeping rhizomes that are very resistant to erosion during floods. Other fresh alluvium unoccupied by trees has patches of rank annual herbs, such as giant ragweed, Ambrosia trifida , and rough pigweed, Amaranthus retroflexus ; both germinate from buried dormant seed when the substrate is disturbed. In a single growing season, one individual of either species may produce tens of thousands of seeds, some of which survive burial in the soil for decades until the soil is again disturbed.
A different colonizing pattern is followed by pokeweed, Phytolacca americana . Like many pioneers, it only germinates in disturbed soil. However, it grows well in both sun and shade and, once established, is not easily eliminated by competitors. The perennial root sends up new shoots every year for 30 to 40 years. The berries attract birds, which disperse the hard seeds widely; the seeds may remain dormant in the soil for decades until disturbed. Pokeweed is not restricted to stream banks, but they probably were its evolutionary cradle.
I mapped the distribution of individual pokeweed in a 500 by 1,500 m study site on the arboretum's Meramec floodplain and adjacent bluffs in 1947
and 1950; the same area was remapped by Alan Covich in 1962 (unpublished data). In 1947 the floodplain part of this site had 175 pokeweed plants, some in areas that had been recently logged, but many others in places with no trace of human activity. In the next 15 years, most of the original plants were carried away by flood erosion or crowded out by competition, so that only about a dozen of the original plants survived in 1962. During the same period, nearly 100 new seedlings became established, mainly in freshly disturbed sites. Such perpetual micromigration is probably the normal way of life for riparian species.
San Pedro River, Arizona
(Zimmerman 1969)
Arid southwestern North America has riparian vegetation remarkably similar to the humid east, considering their quite different regional vegetation. Some eastern species, including pokeweed, drop out west of the 100th meridian, but others, including Panicum virgatum , do not. As a rule, the eastern species are replaced by closely related congeners. In the Sonoran Desert of Arizona, the riparian annuals include Ambrosia aptera and Amaranthus palmeri ; riparian trees include Populus fremontii, Platanus wrightii , and Salix spp. Unlike the adjacent upland desert species, which have seedlings established mainly during summer rains, the Arizona desert riparian trees behave like their eastern congeners. Their seedlings are established on substrates wetted by spring snow melt, and they colonize water courses with sustained flow, not summer flash floods. The same is true of some desert riparian shrubs, such as the seep willow, Baccharis glutinosa .
A separate group of riparian species in the desert region is negatively associated with regularly flowing streams and concentrated along washes with ephemeral flows and no groundwater supply. An example is Chilopsis linearis , a tree with seeds that germinate when buried in warm, wet sand after a summer flash flood.
Tamarix and Other Riparian Invaders,
Southwestern North America
(Everitt 1980; Harris 1966; Robinson 1965; Turner 1974)
The tamarisks or salt cedars include various species of shrubs and trees native to riverbanks and wet saline places of the Mediterranean region and
arid parts of Eurasia. Beginning early in the nineteenth century, various Tamarix species were imported by United States nurseries and widely planted for their graceful feathery foliage and pink flowers. The different species are seldom separately identified by American laymen or botanists, so the tamarisk invasion has to be dealt with on the genus level. In this chapter, only the invasion of natural riparian habitats will be considered; the effect of dam building and altered river regimes will be discussed in Chapter 4.
Herbarium specimens record sporadic naturalization along rivers in the Southwest after 1875, for example, on a sandbar in the Gila River, Arizona, in 1898, but occurrences were local until after 1920. As late as 1926 the U.S. Department of Agriculture was experimenting with planting tamarisk along the banks of the Rio Grande for erosion control. Before 1930, the main tamarisk invasion was under way, and during the 1930s came general awareness from Texas to California of its arrival as an aggressive invader.
The greatest tamarisk infestations in natural habitats in the Southwest are along major rivers that flood from snowmelt in late spring and early summer. Like native riparian trees and shrubs, tamarisk produces seeds that are only briefly viable. Flowering and fruiting, however, extend from late spring through summer, so viable tamarisk seed is blowing in the wind and floating in streams in normal flood season. Tamarisk seeds can germinate while floating. Their best chance of establishment is when they are stranded on fine, fresh alluvium that remains moist enough for rooting.
The native Baccharis glutinosa , tamarisk's most direct competitor, has an advantage in producing seed all year, and if spring melt is early, its seedlings get a headstart. On the Gila and San Pedro rivers, Arizona, however, tamarisk eventually invaded established Baccharis thickets and took over. Tamarisk thickets also prevent establishment of new seedlings of Populus .
Along streams in the southwestern United States and northwestern Mexico that are dominated by summer flash floods, tamarisk has remained a relatively minor component of riparian vegetation. This may not be due directly to the seasonal difference but rather to the coarser sediments and rapid drying. However, scattered tamarisks have colonized sandy washes.
Tamarisk has recently jumped about 40 km of ocean to colonize Santa Cruz Island off the southern California coast. Starting in 1977, isolated tamarisk bushes have been found every few years at various places on the island. Each time the tamarisk was destroyed without being allowed to produce seed. The nearest known seed source is at stream mouths on the mainland coast near Santa Barbara. It seems likely the seeds are blown across the channel by the occasional strong northerly winds.
Several other exotic species deliberately introduced into the southwestern United States have escaped and spread widely on streambanks; Russian olive, Eleagnus angustifolia , and Siberian elm, Ulmus pumila , are examples.
The coastal mountains in southern California have a fairly rich native riparian flora, although few of the streams are perennial. Gallery woodlands are dominated by deciduous species of Alnus, Populus, Salix, Platanus, Acer , and Juglans and by evergreen Quercus and Umbellularia . Understory species include Baccharis glutinosa and Artemisia douglasiana . Yet even where free from human disturbance, this apparently well-stocked community is commonly invaded by exotic shrubs, especially the African castor bean, Ricinus communis , and the South American tree tobacco, Nicotiana glauca . The castor bean is believed to have been introduced during the Spanish colonial period as a source of oil for lamps and medicine. The tree tobacco is native to riverbanks, gullies, and rocky cliffs in the Chaco and Mato Grosso, but may have come to California via Mexico, where it is widely naturalized. The earliest available California record is from 1879 at Los Angeles. German ivy, Senecio mikanioides , native to South Africa, is currently festooning some southern California riparian woodlands with masses of vines.
Riparian Invaders, Australia
(Buckley 1981; Meadly 1954)
In arid central Australia, few alien plants have penetrated most habitats, even along tracks of oil exploration. Water courses are the main routes of invasion. Just as in California, Ricinus communis and Nicotiana glauca are naturalized on stream banks; other aliens include a European sowthistle, Sonchus oleraceus , and a South American cocklebur, Xanthium spinosum . All of these had arrived in coastal Australia during the nineteenth century.
A prickly poppy, Argemone ochroleuca , native to arroyos of Mexico, had arrived in New South Wales by 1845; it has since spread widely, mainly as a weed but also along streams. In 1981, I saw thriving colonies of it along a natural stream channel 150 km inland from Townsville in Queensland where it was growing on fresh sand bars.
In the Northern Territory, a tropical American riparian shrub, Mimosa pigra , arrived at Darwin in 1947, believed to have come via Indonesia. In 1952 it was noticed along the banks of the nearby Adelaide River. By 1983 it was present in masses along various rivers across about 250 km of the Northern Territory coastal region. It is a spiny, rank-growing shrub, up to 4 m tall, that competes effectively with the native floodplain vegetation. The seed is dispersed by both water and animals and remains viable for long periods. Control is currently being attempted with imported New World insects.
Comment
Stream banks have evidently been primary breeding grounds for a large roster of colonizing species. Colonization by riparian species is commonly tightly integrated with river flooding, which opens up new habitat patches, disperses floating seed, and leaves moist sediment for seedling establishment. Vogl (1980) made the same point for trees growing along usually dry washes in the Mojave Desert of California. Flash floods destroy old populations and at the same time create suitable conditions for germination and establishment of new individuals. Some woody legumes, including the smoke tree, Dalea spinosa , require stream abrasion for seed germination.
For riparian species capable of perpetual micromigration, a drainage basin offers a relatively continuous and permanently available territory. However, riparian species are not usually confined to single drainage basins. In addition to being dispersed by water, they are evidently widely dispersed by wind or birds. Numerous cases of naturalization following even longer range human introductions show that species had not all filled their potential areas. Examples could be multiplied for other regions than those mentioned above. An extreme case occurs in New Zealand; according to Dansereau (1964), New Zealand stream banks have been so completely taken over by exotic plants that it is now unclear whether they have displaced native species or invaded an empty niche. The invaders are mostly European species of Salix, Alnus, Ulex , etc., but Lupinus arboreus from the California coast has joined in.
Debris Avalanches And Earthflows
White Mountains, New Hampshire
(Flaccus 1959)
Over 500 debris avalanches and earthflows were located on aerial photographs of the White Mountains. Vegetation was sampled across seven of these of known ages, ranging from 9 to 72 years; most were initiated by intense summer rains. Substrates were heterogeneous, including talus, glacial till, and bedrock. In general, where the slide left areas of stripped bedrock or rock debris, almost no revegetation had taken place. Areas where organic matter and soil were mixed into the displaced material were rapidly revegetated. On a 9-year-old slide, colonists of such areas included native meadow herbs, Achillea millefolium and Epilobium spp.; pioneer trees, Populus tremuloides, Betula lutea , and B. papyrifera ; and a Eurasian weed, Rumex acetosella .
On a 19-year-old slide, the pioneer herbs were almost gone in a dense thicket of aspen and birch. Picea rubens and Abies balsamea , the general dominants of adjacent mature forests, were rare on younger slides but were becoming dominant on a 72-year-old slide; there, shade-tolerant forest herbs were beginning to appear.
Gothic Mountain, Colorado
(Langenheim 1956)
In the spring of 1923, after a winter with twice the normal precipitation, saturated old morainal mantle slid off a steep slope taking some of the underlying friable shale with it. The slide extended from about 3,000 m down to about 2,750 m elevation.
A study 24 years later found the adjacent vegetation to be a sagebrush steppe, grazed by livestock; dominants were Artemisia tridentata and Festuca thurberi with groves of Populus tremuloides . On most of the slide, the dominant was Chaenactis alpina , a perennial herb that ranges widely through the high mountains of western North America on rock outcrops and talus. Other native pioneer herbs included Senecio atratus and Epilobium angustifolium. Populus tremuloides had colonized the slide but was not yet abundant. Eurasian weeds present on both the breakaway headslope and the debris toe included Poa pratensis, Sitanion hystrix, Bromus inermis , and Taraxacum officinale .
Valdivian Andes, Chile
(Veblen and Ashton 1978; Veblen et al. 1981)
In 1960, a great earthquake (8.75 on the Richter scale) in southern Chile set off thousands of dry debris avalanches, landslides, and mudflows. Aerial photographs showed that over 25,000 hectares of land slid, much of it on steep slopes blanketed with deep, water-soaked volcanic ash. The event was not unique; since the Spanish conquest, the region has had 7 comparable great earthquakes and 40 other strong ones. Volcanic activity is also frequent. The regional forests are typically even aged stands dating from slides or ash falls. Some older stands have been invaded by shade-tolerant, late successional species, but even where there has been no logging or clearing, equilibrium steady-state forests are perhaps not found anywhere. Various hardwood trees, mainly Nothofagus spp. and Eucryphia cordifolia , both genera having wind-dispersed seeds, dominate the forests of all ages.
In 1975–1976, vegetation on surfaces exposed by the 1960 slides and adjacent older surfaces was studied. In general, colonizing species were shared
between exposed ash and rock slides, although colonization of unstable ash was slower than on stable ash and rock slopes. Seedlings of the dominant trees in the surrounding forests had evidently begun colonizing within 4 to 5 years of the earthquake. On loose ash, the Nothofagus seedlings invaded gradually from the periphery, perhaps not because of dispersal limits but because of better substrate conditions, including available soil fungi that form symbiotic ectomycorrhizae on the roots.
Shade-tolerant shrub species in the adjacent older forests were rare or absent on the new slides. Some have heavy seeds, such as Persea lingue , but others are widely dispersed, and their failure to establish must be attributed to habitat problems. The common shrub colonists were mostly pioneer bird-dispersed species of forest edges, clearings, and burns, for example, Fuchsia magellanica, Coriaria ruscifolia , and Buddleia globosa . The native bamboos, Chusquea spp., which are extremely abundant in older secondary vegetation of the region, were missing from the slide surfaces. They flower and produce seed only at intervals of decades and vegetative spread is slow.
A native forest margin herb, Gunnera chilensis , colonized rock slides much more successfully than ash slides. It is a perennial with huge leaves and symbiotic nitrogen-fixing blue-green algae within its tissues; its succulent red fruits are probably attractive to birds. Most herb colonists on the slides are weedy Eurasian species, annuals and perennials, mostly wind dispersed, that probably seeded in from artificially disturbed habitats, for example, Poa pratensis, Holcus lanatus, Rumex acetosella, Prunella vulgaris, Digitalis purpurea , and Hypochoeris radicata .
Comment
Landslides are a normal feature of the earth's tectonically and volcanically active mountain systems. Innumerable examples could be cited; for example, an estimated 45,000 hectares of Darien Province, Panama, were denuded by earthquake-triggered landslides in 1976 (Garwood et al. 1979). Slides can be activated without earthquakes simply by saturation with heavy rains. Geomorphologists commonly use a Southeast Asian term, lahar , for slides with a high water content. Lahars are major contributors to the landscape of many regions, including northwestern North America (Franklin and Dyrness 1973). They are especially prevalent on volcanic ash but can occur on a variety of substrates.
Landslides do not have such discrete floras as the habitats previously discussed; they probably occur too erratically in space and time to support an unshared flora. However, it does not follow that this habitat has played an insignificant role in migration and evolution of pioneer plant species. Some generalist pioneer species may have always been shared between landslides and other disturbed habitats, all of which helped shape their adaptations
and distributions. For example, quaking aspen, Populus tremuloides , is one of the most cosmopolitan trees in North America; it pioneers in a wide variety of sites, including glacial outwash and volcanic cinder cones. A significant part of the species population lives on landslides in various mountain ranges. Aspen produces good seed crops every 4 to 5 years, releasing tremendous quantities of seed, buoyed by long silky hairs, that can be carried for miles by air currents. The ripe seed, which is produced in the spring, is nondormant and highly viable, although successful seedlings are infrequent. To survive, a seedling needs constantly moist, bare mineral soil. Once established, a single aspen can spread into an indefinitely large clonal grove by root suckering. Spread by suckering is stimulated by almost any kind of disturbance. A new and wet landslide provides ideal conditions for aspen seedling establishment, and continued instability aids clone expansion. If an aspen clone can escape being overwhelmed by competition from Picea or other later successional trees, within 20 years it will begin producing seed, which may colonize another landslide or other opening.
In the cases discussed above, except for the few species noted as bird dispersed, most of the pioneer colonists of landslides are capable of wide wind dispersal. This applies to both natives and introduced weedy species. However, the bulk of the populations of the latter are in artificially disturbed habitats, and landslides may be relatively insignificant as migrational pathways.
Recently Deglaciated Land
Mount Robson, British Columbia
(Tisdale et al. 1963)
Valley glaciers on Mount Robson, the highest peak in the Canadian Rockies, have been generally receding since 1780, with several halts during which recessional moraines were deposited. In 1963, vegetation was studied on three recessional moraines that had been abandoned by ice 50, 73, and 160 years before. The valley in which the moraines lie is obviously within the seed shadow of a dense, old Picea engelmannii forest growing on the unglaciated slopes above the glacial trim line.
In the moraine available for colonization for 50 years, plant colonization was confined to well-drained gravel and sand ridges. These had sparse, low-growing perennials, mainly Dryas drummondii, D. octopetala , and Hedysarum mackenzii , all three of which have root nodules with nitrogen-fixing bacteria. Rarer shrubs were Arctostaphylos alpina and Salix spp. The entire
roster are delegates, not from the adjacent spruce forest, but from high terrain above timberline. Dryas and Salix are wind dispersed; the others are dispersed by birds or streams.
Ridges exposed for 73 years still had the same species roster and a few Picea seedlings. On ridges exposed 160 years, Arctostaphylos was much denser, and the Picea tree canopy covered about 20% of the area. Wet swales between the ridges still had almost no vegetation.
Glacier Bay, Alaska
(Cooper 1939; Crocker and Major 1955; Lawrence 1958; Lawrence et al. 1967; Mirsky 1966)
Valley glaciers here have retreated nearly 100 km during the last 200 years, but most of the lost area has been invaded by the sea to form fjords. Revegetation of areas above sea level has been followed with the aid of maps and photographs dating back to 1886.
On the youngest moraines, which have been-ice free for 16 years, the first seed plant colonists, along with mosses and Equisetum , were Dryas drummondii, Salix arctica , and Epilobium latifolium . All of these are wind dispersed, presumably from above timberline; none are members of the nearby mature spruce forest, here dominated by Picea sitchensis . On slightly older moraines, these were joined by other Salix spp., Populus trichocarpa , and Alnus crispa , the last of which has root nodules with nitrogen-fixing bacteria, like Dryas .
The raw moraines had a high pH due to a high marble content, but as soon as plant colonization began, the acidity of the surface soil increased rapidly along with organic carbon and nitrogen compounds. During 20 to 40 years of dominance, Dryas increased the nitrogen content of the soil and plant system from about 35 kg/ha to about 400 kg/ha. Populus trichocarpa grew best after establishment of a Dryas cover. The carbon and nitrogen content of the soil increased more rapidly after arrival of Alnus and peaked after about 100 years under alder.
Picea sitchensis seedlings began to invade the alder thickets early but remained rare and stunted, the oldest trees in a given site being all very small compared to some younger spruce on the same site. From about 65 to 170 years after ice retreat, the spruce increased in growth rate and gradually replaced the alder entirely. After that, the nitrogen content of the soil declined. About 200 years after the area was free of ice, the spruce forest was invaded by hemlocks, Tsuga heterophylla and T. mertensiana . Occasional seedlings of these species had appeared much earlier but were suppressed. Like the first pioneers, all the later arriving trees are wind dispersed; it is inconceivable
that the sequence of immigration was controlled by differences in dispersal.
Poorly drained areas had a different history. The only common tree colonist was Pinus contorta . Eventually these areas became blanketed with wet, acid Sphagnum , which formed an expanding peat bog at the expense of conifer forest.
A sequence differing only in minor details was reported from moraines left by retreat of the Juneau Ice Field in Alaska (Crocker and Dickson 1957).
Rhone and Aletsch Glaciers, Switzerland
(Ludi 1958)
Valley glaciers here have been in general retreat since the mid-nineteenth century. The deglaciated valley floors lie below slopes forested with Picea abies , with Larix decidua higher up. Intensive study of areas that had been free of ice only 10 years or less showed that the first pioneers were alpine herbs that grow in meadows above timberline and along mountain streams, such as Saxifraga aizoides , a circumboreal arctic-alpine species. Some of these species were only present briefly and may have been derived from seed that had landed on the glacier and was deposited by meltwater.
About 10 years after ice retreat, some seedlings of woody species were present, for example, Alnus incana, A. viridis, Betula pendula , and Salix spp. By 25 years after exposure of the ground, Alnus thickets were established and producing seed; a few stunted individuals of Picea and Larix were present.
Laguna San Rafael, Chile
(Heusser 1964; Lawrence and Lawrence 1959)
By 1882, a lobe of the huge North Patagonian Ice Field that had reached tidewater in Laguna San Rafael began a slow recession. From 1910 to 1935 recession was rapid. Between 1940 and 1958, the edge remained nearly stationary, with a slight advance that overran some young forest just before the vegetation was studied in 1959. A total of 10 recessional moraines left between 1882 and 1940 were given approximate dates from historical records, maps, and tree rings.
Unglaciated mountain slopes above the glaciated valley bear a cool temperate rainforest dominated by hardwoods, Nothofagus spp., Weinmannia trichosperma, Drimys winteri , and a conifer, Podocarpus nubigens. Chusquea quila forms bamboo thickets where the forests have been cut or burned.
The youngest deglaciated surfaces available for study had been available for colonization about 25 years. Better drained moraines and outwash areas on those surfaces had been colonized by the gigantic herb, Gunnera chilensis , and its smaller mat-forming relative, G. magellanica ; both have nitrogen-fixing blue-green algae in their leaf axils. Other pioneers included tree ferns and flowering shrubs, such as various species of Empetrum, Pernettya , and Baccharis . Also, a few seedlings of rain forest trees, such as Nothofagus and Drimys , and a forest margin shrub, Fuchsia magellanica , had appeared but no Chusquea .
Better drained areas available for 50 to 75 years were covered with young Nothofagus and Drimys trees; the pioneer species were dying out and seedlings of Weinmannia and Podocarpus were establishing. Poorly drained areas on all these surfaces had grassy marshes and ponds with freshwater aquatics, such as Potamogeton and Myriophyllum spp.
Comment
In the cases cited here, freshly deglaciated terrain clearly presents edaphic problems that bar immediate immigration by species from adjacent mature forests. On better drained moraines, these barriers are evidently gradually relaxed after roughly 50 years, evidently by changes in soil under pioneer vegetation; nitrogen fixation by symbiotic bacteria may be crucial. Invasion of poorly drained areas is even slower.
Modern deglaciated terrain is too restricted in time and space to have a special flora. The pioneer colonists have a variety of primary habitats, including alpine tundra, mountain streambanks, and scree. All are adapted for fairly long-range dispersal, many by wind.
Emerging Nonvolcanic Islands
Baltic, Finland
(Barlund 1940; Luther 1961)
The Baltic Sea is constantly gaining new islands as isostatic rebound of the earth's surface, relieved of the weight of the continental glacier, still proceeds. The floras of 22 tiny islets off the southwest coast of Finland have been censused repeatedly, in most cases at least three times over a period of
more than 50 years. The islets are mostly bare granite and gneiss scoured by glacial ice. The largest is about 4 ha in area and about 10 m high. At the calculated rate of emergence of about 45 cm per 100 years, the oldest island has been exposed for about 3,000 years and the youngest about 150 years.
A total of 174 species of seed plants and 7 species of ferns were recorded on the 22 islets. One island had no flora in three censuses spanning over 50 years; it was not the smallest or lowest of the islands but one of the most isolated and exposed. Although about 2 m high and about 400 years old, it was still washed over by storm waves. Numbers of species in the floras of the other islands ranged from 1 to 108 in a given census. Ten of the islands had 1 to 15 species at their first census, and only five of these had more than 15 species at their last census. These low counts were found on islets where plant colonization was restricted to crevices in otherwise bare rock. The commonest species in these crevices were Allium schoenoprasum, Juncus gerardi, Festuca rubra, Puccinellia retroflexa, Agrostis stolonifera, Rumex crispus, Sagina procumbens, Cochlearia danica, Sedum acre, and Matricaria maritima . These are perennial herbs that are widespread in Europe, mainly in salt marshes and rocky seashores; some also grow in open inland habitats. None have obvious mechanisms for long-range dispersal, but they are believed to be carried by birds and flotation.
The other 11 islands had over 20 species at each census. Usually these included all the species noted above in rock crevices. Additional species were found in two other habitat types: pocket beaches and interior flats and hollows beyond reach of the waves. Beaches were colonized intermittently by a few common sea-dispersed species: the annual Cakile maritima ; the perennial vine, Lathyrus maritimus ; and the perennial grass, Elymus arenarius . These colonists did not always persist and may have been recruited repeatedly from the mainland.
Sheltered interior flats and hollows had a richer flora, both wind and bird dispersed. Hollows with freshwater pools had Typha latifolia, Lemna minor, and Triglochin palustre . Fringing the pools were marshy meadows, mossy bogs, and heaths with a rich mixture of sedges, rushes, and grasses, including Poa pratensis and Phragmites australis , and dicot herbs, including Galium palustre, Epilobium plaustre, and Cornus suecica . Shrubs included Juniperus communis and a variety of heaths and their relatives, particularly species of Calluna, Empetrum, and Vaccinium . Tree species mainly colonized the interiors of the largest islands. The only tree present during the first census was Sorbus aucuparia . By the later censuses, Pinus silvestris, Picea abies, Populus tremula, Betula pubescens, B. verrucosa, Salix aurita, and Alnus glutinosa were present.
Successive censuses found many changes in the floras of most islets. For example, one medium-sized islet was censused five times over a 62-year
period; it initially had 43 species of which 34 were found in all succeeding censuses. The number of species present increased with each succeeding census to 89 in the final count. A total of 103 species were found in at least one census, of which 25 disappeared at least temporarily in later censuses. A similar pattern was found on the other islets: almost every census showed more species than the previous one, but often a few species had died out. These ephemeral colonies often consisted of a single or few individuals, often nonflowering. The islets are probably continuously inoculated with seed of beach plants and inland pioneers produced by mainland populations upstream and upwind.
At the last census there was an outburst of seedlings of weeds and crops on islets used as nesting grounds by gulls. Shortly before, the gulls of south Finland had shifted their foraging behavior by increased feeding in agricultural fields and rubbish dumps. Luther (1961) found regurgitated gull pellets responsible for much seed dispersal. Seabirds evidently were also responsible for a few cases of species extinction. One islet originally had a stand of shrubs, including Juniperus, Empetrum, and Vaccinium, which died out completely after a buildup of nesting birds. Extinction of several herb species was attributed to an exceptional buildup of voles.
Plants on these rocky, windswept islands might be expected to be highly vulnerable to drought. Two exceptionally dry years occurred between the last two censuses; August in one of those years was the driest since records had been kept in southern Finland. On seven islets, several species present in small numbers before the drought apparently died out. Dieback of other species was heavy, but recovery by sprouting and seedlings was rapid in most cases.
The steady immigration of new species during the study period is not easily explained. For example, why should the highest island, which has existed for about 3,000 years, have had 33 species at the first census and 58 species 50 years later? On some of the other islands, the increases also seem too abrupt to be due to fortuitous dispersal of species that had not happened to arrive before. Uplift of about 25 cm during the study period would have increased the islet areas only slightly. In some islets, uplift might have raised surfaces above a threshold, beyond reach of sea ice and storm waves. Once freshwater pools are beyond reach of the sea, a slow process of autogenic succession could begin. As peat and turf develop and expand over surrounding rock surfaces, colonization by heath and tree species might begin. Also, there may be a positive feedback between complexity of an islet's flora and vegetation and the amount of bird traffic visiting the islet.
The islets discussed above are mainly bare rock. The processes of colonization and succession evidently proceed faster on nearby islets composed of loose morainic material. For example, in the Ahvenanmaa Islands, some islets
that emerged only 250 years ago already have thickets of Alnus glutinosa and Hippophae rhamnoides .
Gulf Of Bothnia, Finland
(Vartianen 1980)
Isostatic rebound here is even faster, about 75 cm per 100 years, creating shoals of new islands composed of glacial debris that has been reworked by the sea. Plant colonization has been studied by two complementary methods.
Time-lapse vegetation maps were made for four relatively large, old islands that emerged from the sea about 1,000 years ago. Maps in the national archives of the Surveyor General were used to plot vegetation in the years 1770, 1880, 1930, and 1958. Aerial photographs were used to plot vegetation in 1977. All the maps were reduced to the same scale, and they show distributions of sand beaches and dunes, boulder shores and ridges, sedge and grass meadows, swamps, lagoons, fens, heath scrub, deciduous woodland, and pine woods.
The map sequences show fascinating changes as the four islands rose and expanded. The changes may have been modified by sheep grazing, which continued on the islands until the 1940s. The A.D. 1700 maps, made when the islands were more than 700 years old, show bare sand and boulder areas, meadows, and heaths, but no woods. By 1880, the same types, greatly expanded in area, still covered the islands. By 1910, there were small patches of deciduous woodland and swamps in the interiors of the islands, while the meadow and heath areas were displaced toward the expanding peripheries. By 1930, deciduous woodland had expanded on some islands but had changed to a swamp on another; there were small areas of pine woods. The greatest change in vegetation came between 1930 and 1958 when the central deciduous woodlands expanded greatly into former heath and meadow areas; there was also some expansion of pine woods. Perhaps this was partly because the islands had passed a critical threshold of size or age before woodland could develop, and perhaps it was partly because of sheep removal. Between 1958 and 1977, there was less change.
Another study method used belt transects of 150 islands in the same archipelago to characterize species patterns in the modern vegetation. The data have no time depth except as the height above sea level indicates the time available for plant colonization since emergence. Most of the islands are ungrazed, but some have been visited by sealers and fishermen. Nearly 300 species were found. Their patterns show a rough zonation correlated with elevation and thus with age of the surface since emergence. Sea ice pushed ashore by the wind affects the lower margins of vegetation.
Lake Hjälmaren, Sweden
(Birger 1907)
In 1882–1886, this central Swedish lake was partly drained, its level being lowered about 2 m to reclaim 15,000 ha of farmland. A large residual lake remained in which 29 new islets had emerged; although none were more than 2 m high, 26 of them were no longer washed over by the gentle waves of the lake. Most of the new islets were separated by at least a few kilometers of water from older land surfaces. The islets were partly cracked rock surfaces and partly morainic material.
Plant colonists were censused after 4, 10, and 22 years. The first census found 113 plant species, mainly freshwater marsh plants and common annual agricultural weeds, but also found Betula verrucosa, Populus tremula, and Alnus glutinosa . Possible dispersal agents include wind, birds, water currents, and floating ice. It is unknown which species had dormant seed on the lake floor before drainage.
A total of 260 plant species were found in the three censuses combined, many only once. Most islands peaked in species number 10 years after emergence. The pioneer hardwood trees were then joined on some islands by Pinus silvestris and Picea excelsa . By 22 years after emergence, Quercus robur and Tilia europea had appeared; by then some of the pioneers were being crowded out by the developing tree cover. For example, the marsh annual Bidens tripartitus was present on 14 islands at the first census, all 26 islands at the second census, and 10 islands at the third.
Comment
This set of cases shows how difficult it is to generalize about colonization of new islands, even within a relatively restricted and climatically homogeneous region. Some islets had a rich flora, including several tree species, within 4 years of emergence. Another islet of similar size had no plants 400 years after emergence. One archipelago began acquiring trees 900 years after emergence; another was nearly 3,000 years old before it began acquiring trees. Some islands reached a peak in number of plant species 10 years after emergence, and then species numbers declined as pioneers were eliminated by competition. Other islands that were thousands of years old doubled their species numbers during 50 years.
It is impossible to explain such differences by any model based simply on quantitative measures of islands, for example, area, height, distance from seed sources, and age. Habitat quality must be included as a crucial variable. In these cases, the most obvious habitat variables are exposure and substrate.
The most rapidly colonized islands were in a lake with low-energy waves; their substrate included loose glacial till and water-borne sediment. The islands with the slowest colonization rates were exposed to sea waves and brackish spray; their surfaces were mainly rock. Islands with intermediate colonization rates had a complex of different habitats. Habitat complexity may be partly a function of island area and height, but the relationship is not linear. Habitat complexity may increase abruptly when size and height thresholds are passed. Once interior areas are beyond reach of waves and spray, a new flora can colonize and initiate successive changes that create opportunities for additional immigrants.
This set of cases all involves islands that are rather close to seed sources; the islands are probably within the normal seed shadow of far more species than have immigrated. In other words, screening of the colonizing species was generally by environmental selection rather than by dispersal barriers. However, dispersal has not necessarily been constant; for example, a change in seagull foraging patterns evidently led to an outburst of ephemeral crop and weed seedlings on gull nesting lands. Extinction of these unsuccessful immigrants was evidently due to lack of suitable habitats. Also, a few cases of temporarily successful immigrants becoming extinct are attributable to habitat change, for example, pioneer species being eliminated by succession and marginal populations being killed during extreme drought.
Fresh Lava And Pyroclastic Deposits
Paricutin, Mexico
(Beaman 1961; Eggler 1963; Rees 1979)
The town of Paricutin, Michoacan, was built on the slopes of a great Pleistocene volcano, which had been dormant long enough for even the steeper cinder slopes and lava benches to become heavily wooded. There were forests of fir, Abies religiosa, at high elevations, woodlands of Pinus and Quercus species at intermediate levels, and complex broadleaf subtropical forests in the deep barrancas. In the pine—oak zone, gentler slopes with good soil had mostly been cleared for milpas.
The new volcano began erupting in 1943 and continued to be active until 1951, eventually engulfing the town. Although a relatively small flank volcano, it emitted over 2 billion tons of ash and other pyroclasts and over 1 billion tons of lava, mostly of the blocky aa type. Lava flows and cinder cones wiped out all vegetation on about 2,500 ha; ash falls caused partial
kill on about 35,000 ha. Pines and oaks survived ash falls better than the higher elevation firs and the lower elevation subtropical trees. In milpas covered with shallow ash, only a few weeds survived, notably Cynodon dactylon , an African grass now ubiquitous in weedy vegetation of warm regions. Attention here will be focused on areas where revegetation was by colonization, not regrowth.
Colonization on lava flows proceeded faster than on cinder cones or ash. By 1960, lava flows had abundant blue-green algae, lichens, mosses, and ferns; crevices and pockets had scattered individuals of over 30 seed plant species. By 1978, young trees of three species were common on lava: Pinus leiophylla, Clethra mexicana, and Buddleia cordata . Early colonists of cinder cones included a few ferns; native shrub species, such as Phytolacca icosandra, Wigandia kunthii ; and cosmopolitan weeds, such as Digitaria sanguinalis, Conyza canadensis, and Sonchus oleraceus . Areas mantled with deep ash remained barren until the ash was enriched with water-transported soil or organic debris or where erosion exposed underlying soil. Such areas were colonized by shrubs and herbs native to rocky slopes and ravine banks; most were composites, for example, Baccharis, Eupatorium, and Senecio spp., or legumes, for example, Dalea, Desmodium, and Crotalaria spp. One was a Ceanothus species, which, although not a legume, probably has nitrogen-fixing root bacteria.
A few of the colonizing species are adapted for bird dispersal, including Pinus, Phytolacca, and Gaultheria , but most are wind dispersed. Screening of colonizing species from the rich regional flora is, however, obviously mainly by habitat conditions rather than dispersal limits.
Irazu, Costa Rica
(Parsons 1972)
This huge ancient volcano resumed activity in 1964–1965. Ash falls on the slopes below killed many oaks and other native trees and buried the grasses and other herbs in areas cleared for dairy pastures. After the eruption was over, the ash falls were rapidly invaded by Kikuyu grass, Pennisetum clandestinum , native to the volcanic highlands of Kenya. Kikuyu grass had been introduced to Costa Rica about 1928 but was generally considered a pest until the eruption. Because of its vigorous colonization of the ash falls, it has become the preferred grass for dairy pasture at elevations between 1,800 and 3,000 m on Irazu.
In 1976, I found that the new cinder cone at about 3,200 m elevation was still mostly devoid of vegetation. There were patches of Rumex acetosella, R. obtusifolia, and Melilotus alba , Eurasian annuals that are now cosmopolitan
weeds in temperate climates. There were also patches of the native Lupinus aschenbornii and Coriaria ruscifolia. Coriaria ruscifolia is a strikingly unusual shrub with a highly disjunct range in the American Cordilleran system at high elevations and in the southern hemisphere at high latitudes. In the Cordillera it ranges from the western Sierra Madre of Mexico down to Chile in a variety of open habitats, including ravine banks, landslides, and burns. The same species is native to New Zealand, where it was a prominent colonist of the Tarawara Volcano after a great eruption in 1886 (Cockayne 1927). The seeds are enclosed in a succulent fruit and are presumably bird dispersed. Although not a legume, Coriaria has symbiotic nitrogen-fixing bacteria.
Cameroons Mountain, Cameroon
(Keay 1959)
This colossal volcano towers more than 4,000 m above the shore of the Gulf of Guinea. An eruption in 1922 left a fresh lava flow 10 km long and over 1 km wide extending from 1,400 m elevation down to the sea. The adjacent vegetation was a tropical rainforest, the region being one of the wettest in the world. About 15 years later, the lower elevation parts of the lava flow had been colonized by various spore plants and over 40 species of flowering plants. Another study 29 years after the eruption, made by a different person in a slightly different place, produced an almost identical plant list, with only 5 additional species.
The dominant colonist was a tree in the coffee family, Hymenodictyon biafranum , common on open rocky sites in the West African mountains; its seeds are wind dispersed. The next most abundant was a dragon's blood tree, Harunga madagascariensis , with a wide, disjunct range extending to Madagascar and Mauritius. It grows in rocky banks, along streams, and in wet swales; it is evidently bird dispersed. Also bird dispersed were several species of Ficus and Psidium guayava . The figs are native; the guava was introduced from the American tropics as a planted fruit tree soon after 1500 A.D. and promptly escaped. Another common colonist on the Cameroon lava flow was kapok, Ceiba pentandra , one of the world's most enormous trees, also native to the American tropics where it is a pioneer in riparian and other open sites. Baker (1965b ) concluded that Ceiba arrived in West Africa in ancient times, probably by means of ocean-drifted fruits, and spread across Africa as a pioneer of riparian and other open sites. Its seeds are produced in huge quantities and are easily carried by the wind after a mature fruit dries and breaks open.
The lava flow had also been colonized by various herbs, both wind and bird dispersed, including Axonopus compressus, Paspalum conjugatum, Asystasia gangetica, Borreria ocymoides, and Emilia coccinia . These are mostly of doubtful nativity and are now common pantropical weeds.
Mauna Loa, Hawaii
(J. O. Juvik, personal communication 1977; Smathers and Mueller-Dombois 1974)
Kilauea is a still-active volcano at about 1,200 m elevation on the lower slopes of Mauna Loa. Kilauea has produced many prehistoric and historic lava flows, cinder cones, and ash beds. Under the tropical rainforest climate, a dense forest dominated by Metrosideros polymorpha has developed on some of the prehistoric lava, including the floor of a satellite crater, Kilauea Iki. In 1959, an eruption of Kilauea Iki buried this forest under a lava lake 100 m deep and 56 ha in area; the lava was mainly of the smooth, ropy pahoehoe type. The same eruption built a cinder cone covering 19 ha and scattered pumice over a larger area, in part of which the pumice was deep enough to cause total kill of the vegetation.
A study done 9 years after the eruption found that the lava lake had not yet cooled in the center, which steamed after every rain. Even where no longer heated from below, the dark lava was heated intensely by the sun, and water retention on the smooth pahoehoe was nil except in crevices. Around the totally bare, hot center was a wide concentric zone sparsely colonized by algae, mosses, and ferns. Although the lava lake lay downslope and downwind from an undamaged rainforest, less than 1% of the lava surface was colonized by seed plants. The species that had gone farthest onto the lava lake were three endemic woody sclerophylls present in the adjacent forest: Vaccinium reticulatum , which had colonies as far as about 100 m from the edge and about one-third of the way to the center, followed by Metrosideros polymorpha and Dubautia scabra . Behind them, edging onto the first few meters of the lava, were a motley group of soft-leaved perennials, mostly exotics. These included Lythrum maritimum , native to streambanks in the Peruvian coastal desert, which had somehow become naturalized in the mountains of Hawaii in the nineteenth century; Andropogon virginicus , native to savannas and marshes of eastern North America; and three recent garden escapes, Anemone japonica, Buddleia asiatica , and Fragaria vesca .
Moisture conditions on the loose pyroclastic deposits were not much better than on the lava. Water available to plants, defined as the difference between capacity at saturation and the permanent wilting point, was about 3% of the weight of the ash, less than in pure sand. By 9 years after the eruption, the cinder cone had 6 species of seed plants; other areas of total kill under deep pumice had 21 species. The most common species, both native and exotic, were the same as on the lava lake.
Beyond the total kill area, survival increased with decreasing depth of pumice. The Vaccinium, Metrosideros, and Dubautia resprouted even when badly damaged, and seedlings appeared in abundance; thus, retreat of the original species was trivial. The area of partial kill did, however, open a
temporary opportunity for colonization by exotics. About 40 exotic species were present there 9 years after the eruption, including all those found on the lava lake. Two of the most successful were an Asiatic raspberry, Rubus rosaefolius , and an American blackberry, R. penetrans . There were also many sedges and grasses, including Pennisetum clandestinum , Kikuyu grass. However, Kikuyu grass did not blanket pyroclastic deposits here as it did on Irazu (discussed above). On Kilauea Iki in 1977, I found it abundant only on roadsides.
In the same general region in 1977, Juvik conducted a field trip to examine older lava flows, including an 1855 flow on the northeastern side of Mauna Loa. This flow, largely of the blocky aa lava, extended from above 1,800 m elevation to below 1,000 m. The upper part is above the trade wind inversion, has only about 125 cm of rain per year, and was still extremely barren; the lava looked unweathered and very fresh. The middle level, about the same elevation as Kilauea Iki, has about 500 cm of rain per year, and it had a sparse vegetation dominated by the same species of Vaccinium, Metrosideros, and Dubautia that colonized the lava lake on Kilauea Iki. The lower level has about 750 cm of rain and had a similar but denser vegetation.
Metrosideros remains a dominant on the most ancient Hawaiian aa lava flows over a broad range of annual precipitation. This is a good example of the initial floristics model of succession, in which the later dominants are among the pioneer colonizers. Old Metrosideros stands are subject to a patchy dieback, but in a cyclical mortality and regeneration process that does not eliminate it from the vegetation (Mueller-Dombois 1980).
Mount Komagatake, Hokkaido, Japan
(Numata 1974)
In 1929, a violent eruption deposited pumice over a large area of Mount Komagatake from 600 m elevation down to 200 m. The theoretical climax dominant species of forests in the region is Fagus crenata , but the actual forests are mainly secondary, dominated by Quercus mongolica and species of Populus, Betula, Castanea, Prunus, and Sorbus . In the area of total kill, plant seedlings began appearing in 1932 in interstices between the pumice blocks. The colonizing species were mostly wind-dispersed pioneers common in disturbed habitats of the region. The first trees to invade were Salix, Betula, and Populus . The first herbs were widespread weeds: Miscanthus sinensis, Chenopodium album, Epilobium angustifolium, and Anaphalis margaritacea . By 1935, these were joined by other cosmopolitan weeds, including Poa annua and Rumex acetosella , and by a garden escape, Oenothera grandiflora . By 1942, the weedy herbs were being shaded out. By 1960, areas sheltered from the wind had a dense young forest of Populus, Betula , and Salix, with seedlings
of other natives, including Acer, Prunus, Hydrangea, and Rhus . By 1965, this forest had not changed in composition.
Volcanoes on Honshu, Japan
(Numata 1974)
Dated lava flows on several mountains on Honshu permit age and elevation comparisons. Mount Zao had a thick andesite flow about A.D. 750 in the subalpine zone, where Abies mariesii is the theoretical climax dominant species. At the time of study, saplings of this fir had just begun to colonize. The vegetation was still dominated by gnarled scrub of Pinus pentaphylla , with an undergrowth of Empetrum nigrum and other dwarf alpine plants that had moved downslope from their usual zone.
Mount Fuji had a basalt flow in A.D. 864 in a zone where Fagus crenata is the climax dominant. The lava is only slightly weathered, but a recent study found a dense growth of mixed conifers belonging to six genera. Pioneer hardwoods had already begun to colonize, particularly Quercus mongolica and Betula spp.
Mount Asama had an andesite flow in 1783 that extended from the subalpine zone, where the theoretical climax is a conifer forest dominated by Abies mariesii and Tsuga diversifolia , down into the Fagus crenata –dominated zone; at the same time, deep pumice was deposited in the lower zone. A recent study found the subalpine lava flow had been colonized only by lichens, mosses, and a few alpine seed plants, mainly ericads. The lower lava also remained barren of seed plants other than alpine ericads, except that sheltered hollows had scrubby thickets of Pinus, Larix, Alnus, and Betula . Pumice deposits in the lower zone had a dense, young pine forest, with an understory of Quercus, Prunus, and other hardwood seedlings.
Krakatau, Indonesia
(Borssum Waalkes 1960; Docters van Leeuwen 1936; Richards 1964; Whittaker et al. 1984)
The famous eruption of 1883 transformed the old Krakatau Islands, which had been covered with dense forests, into a wasteland of hot pumice and ash. The three new islands are all rugged, the highest peak being over 800 m elevation. Revegetation has been studied by repeated scientific expeditions.
The rapid immigration of sea-dispersed beach species and the assemblage of a rich coastal flora was noted in the section on Tropical Sea Beaches.
Evidently the distances from seed sources were insignificant for this flora, and the environmental obstacles to colonization of fresh pyroclastic beaches were significant for only a few of the member species.
For the inland flora, which is the object of attention here, both dispersal and environmental obstacles have evidently been effective in screening colonizing species. The overseas journey of about 80 km from Sumatra or about 45 km from Java has probably been accomplished by only a fraction of the bird-, bat-, and wind-dispersed floras of those islands.
An 1886 expedition 3 years after the eruption found the only plant life on most of the island surfaces to be blue-green algae and ferns, of which 11 species were reported. There were only 15 seed plant species, mostly sea-dispersed beach plants, and a few wind-dispersed grasses and composites. In the absence of competition, some of the vines normally confined to the beach outpost zone, such as Canavalia maritima and Ipomoea pes-caprae , were moving inland.
By 1897, the inland invasions of beach species were beginning to be contained by competition from inland pioneers that had not been present in 1886. In particular, two coarse perennial grasses, Imperata cylindrica and Saccharum spontaneum , had begun forming savannas over the lower hills and valleys. Both are extremely common and widespread in both naturally and artificially disturbed sites in southeastern Asia and the East Indies. They soon displaced the pioneer ferns and inland incursions of beach plants.
In turn, the savanna grasses were eventually displaced, except on special sites such as steep ridges, by development of a tree canopy. The first trees to arrive were Macaranga tanarius and three species of Ficus . All of these are common on riverbanks and in secondary woodlands in the East Indies, and all are probably dispersed by birds and fruit bats. By 1908, these four species were widespread on Krakatau and fruiting heavily. By then they had been joined by Neonauclea calycina , a secondary rainforest tree from Java which is wind dispersed, and also by Cyrtandra sulcata and Leucosyke capitellata , both understory species in mountain forests of Java and both bird dispersed. Two other bird-dispersed species that are American natives, Carica papaya and Lantana camara , also arrived in Krakatau by 1908; both had escaped from cultivation in the East Indies long ago.
On Krakatau a positive feedback evidently developed between increasing attractiveness of the vegetation to fruit-eating birds and increasing introduction of bird-dispersed species. The earliest plant colonists had been entirely sea and wind dispersed; by 1900, only six bird-dispersed species were present. Since then, the number of bird- and bat-dispersed species has increased over ten-fold, while the number of sea- and wind-dispersed species has increased comparatively slowly.
The first reports of weeds believed to be introduced by human agency were in 1919. From 1916 to 1921, Krakatau was inhabited by a small group of people engaged in pumice extraction. During that time, 17 weedy species
were found on the island for the first time, mainly near the boat landing and around the settlement. An example is Eleutheranthera ruderalis , an American native that had been introduced to the East Indies via botanical gardens; it had escaped in the nineteenth century to become a common weed in Java and Sumatra. Only 5 of these 17 species remained in 1929, the rest probably having been eliminated by competition.
The peak rate of immigration of new species evidently was reached in the 1920s when the developing forest canopy provided suitable habitats for more epiphytes and for species requiring shade and humus.
By 1951, behind the narrow fringe of beach vegetation, forests dominated monotonously by Neonauclea covered almost the entire main island up to 750 m elevation. Macaranga and various Ficus species were subdominants. On the summit above 750 m, Neonauclea and Macaranga were present but did not form a closed canopy; there was a dense shrub layer and patches of Sacharum grassland.
In 1979, a Hull University expedition found that the coastal vegetation had changed little in 60 years, except that Casuarina had lost most of its former area to broadleaf coastal trees. A few more inland species had arrived, such as Dysoxylum caulostachyum , a tree characteristic of primary lowland rainforest in Java, presumably bird or bat dispersed. The rate of immigration appeared to be slowing. Over the preceding 50 years, known additions to the flora averaged about 25 species per decade, while apparent subtractions averaged about 20 species per decade, giving a net observed increase of 5 species per decade. The successive surveys, however, were not equally intensive nor did they sample the same areas, so it is not known what proportion of the apparent species turnover is real.
The cumulative total of all seed plant species observed on Krakatau is slightly more than 300. Even if all these were still present, the flora is not nearly as rich as in comparable terrain in Java or Sumatra.
Surtsey, Iceland
(Fridriksson 1975)
The new island of Surtsey appeared above the sea in 1963. When the eruption was over in 1964, the island had an area of a few square kilometers, about half ash and half ropy pahoehoe lava. Only scientific research workers have been allowed to visit the island; all persons and equipment have been inspected to prevent accidental species introductions. However, some tubs filled with fresh water have encouraged bird visits.
The new island is less than 6 km from an old islet and not much farther from Iceland proper, which has a flora of several hundred seed plant species. Researchers on Surtsey have recorded arrival of seed in abundance, mostly
of species common in Iceland. Some is wind dispersed, for example, Eriophorum, Taraxacum , and Senecio . Seedlings have been found in sea gull droppings and carcasses. Snow buntings from Britain, stopping over on their spring northward migrations, are credited with bringing seeds of marsh plants, such as Polygonum persicaria and Carex nigra . None of these were successful colonists. The only seed plants that have survived to maturity on Surtsey are Cakile arctica and Elymus arenaria , both sea-dispersed beach plants; they may be repeated immigrants from Iceland rather than self-reproducing local populations. No inland seed plant species were established on Surtsey, only blue-green algae, mosses, and other lower plants.
Comment
Fresh volcanic deposits are too erratic in occurrence in space and time to support a discrete flora. Pioneer colonists are a hodgepodge of delegates from various habitats. If pyroclastic deposits are on a seashore, colonists arrive by sea dispersal from ordinary beaches. Inland pioneers are mostly wind or bird dispersed and come from rock outcrops, ravine banks, landslides, burns, and other disturbed habitats; some are weeds and escapes from cultivation.
Because dispersibility and ability to establish in disturbed habitats tend to be correlated, it is hard to sort out their relative importance in determining the roster of immigrants. In the cases of Krakatau and Surtsey, it is unclear how much effect the ocean barrier had on colonization patterns. In all cases, it is obvious that colonization by many species was not barred by failure of seed to arrive but rather by habitat. Fresh lava and pumice are clearly an impossible substrate even for some pioneer species. Lack of lime in pyroclastic beaches evidently prevents establishment of certain beach plants. A more general barrier is evidently lack of available nitrogen; prominent among early colonizers are both legumes and other plants with symbiotic nitrogen-fixing bacteria, such as Casuarina, Ceanothus, Coriaria , and possibly Saccharum .
The case histories generally suggest a relay floristics succession, with the pioneers modifying soil and microclimate until replaced by later colonists. However, the process is slow, even in humid tropical lowlands, and the available histories mostly show early stages.
The Hawaiian succession is anomalous, approaching an initial floristics model. The pioneers on fresh volcanic deposits include Metrosideros polymorpha , the ultimate dominant in old rainforests. For a rainforest tree, Metrosideros is also exceptional in its capacity for long-range dispersal. Its natural distribution extended from New Zealand and Australia through much of Melanesia and Polynesia. In the center of its range it is mainly a forest tree and is important as a pioneer on lava flows on the periphery of its range in New Zealand and Hawaii.
2
Vegetation Subject
To Natural
Perturbations
Recurrent Fire
Arctic and Alpine Timberlines, North America
(Black and Bliss 1980; Heinselman 1981)
Along the Arctic timberline in Canada and in the absence of fire, the dominant black spruce, Picea mariana , can maintain itself indefinitely by vegetative reproduction, regardless of weather. The forests at timberline are probably destroyed by fire at intervals of about 100 to 200 years on the average. Abundant viable seed is released from cones of the burned trees; if temperatures are above normal, a new forest is rapidly established without migration. However, seed germination is suppressed by low temperatures, and after a few consecutive years that are cooler than average, no viable seed remains. The burned area can then be reforested only by gradual reimmigration during favorable years. If the burn is large, which is not unusual, the consequent slow advance of the treeline would be hard to distinguish from migration controlled by climatic change.
Similar oscillations of timberlines may occur on Rocky Mountain subalpine levels, where there is a delicate balance between forest and tundra. Picea engelmannii, Abies lasiocarpa, Pinus albicaulis, and Pinus flexilis are capable of persisting for a very long time in the absence of fires, which may recur
at intervals of up to 500 years. When the forest does burn, recolonization can be expected to be very slow because tree reproduction and growth are very uncertain.
Mosaics in Native Vegetation, California
(J. Keeley 1981; S. Keeley et al. 1981; Minnich, in press; Vogl 1967)
Postulating purely natural fire regimes in western North America is highly hypothetical because burning by aboriginal people has probably been common as long as the present climatic and vegetational patterns have existed. However, natural fire regimes are relevant in a longer evolutionary context. In California, a great many native species have adaptations to survive or regenerate on a site under recurrent burning. Without migrating, they mostly show great resilience under widely fluctuating fire frequencies. There are some cases, however, of chronic local migrations integrated with fire.
Chaparral, a sclerophyll scrub vegetation, is generally burned to the ground about every 20 years, but all the shrub species are capable of either resprouting from the roots or recolonizing immediately from seed stored in the soil or both. The shrub species can be divided into relatively xeric and mesic groups. The large xeric group includes the most abundant species, some, but not all, of which resprout after burning. All of them produce seed that is locally dispersed and stored in the soil until stimulated to germinate by fire. The small mesic group includes Quercus dumosa, Heteromeles arbutifolia, Prunus ilicifolia, and a few other species. They resprout vigorously after a fire but do not establish seedlings in the burns. Their seeds are widely dispersed, usually by birds, and the seedlings are shade tolerant and able to come up under old brush. In scattered places with favorable moisture conditions, these species can outlive, outgrow, and shade out the xeric species; however, the mesic species are decimated in drought periods.
In the mountain woodlands and forests, the potential life spans of the great dominant conifers are much longer than the fire frequency. All the species can live for centuries, Pinus lambertiana to a millennium, and Sequoia gigantea for several millennia. Lightning-set fires are common; under natural conditions they are generally mild surface fires that remove dead fuel and undergrowth, including tree seedlings and saplings, but do not kill older trees. Occasionally, vagaries of burning in irregular topography leave areas unburned for a few decades, allowing growth of dense stands of the more shade-tolerant species, especially Abies concolor . In such stands ground fires escalate into crown fires, resulting in total kill of patches of forest. None of the dominant conifers are capable of resprouting from the roots nor do they store seed in cones or in the soil. After severe crown fires, recolonization depends on immigration. Seed of all the dominant conifers is dispersed by
wind, but usually for very short distances. The combination of erratic burn patterns and local seed dispersal results in a patchy mosaic of different-aged stands with varying species composition. In contrast to the trees, some more ephemeral herb and shrub colonists of fresh burns, such as fireweed, Epilobium angustifolium , are adapted for long-range dispersal.
Below the montane conifer forests on the desert side of the mountains, the low-growing pinyon, Pinus monophylla , has a different pattern of local migration due to fire. This pinyon species grows over much of the great semidesert of western North America in an open scrub woodland that is normally fire free. However, at the upper margin of its range in southern California, pinyon forms denser woodlands; an old, dense pinyon stand is highly flammable. After burning, the trees do not resprout or establish seedlings in fresh burns. Recolonization depends on immigration, probably primarily by bird dispersal. Pinyons have a symbiotic relationship with several species of jays and nutcrackers, which harvest the heavy, wingless seeds and transport them to storage sites, commonly over 10 miles away. The birds bury the pine seed in the soil in open sites, often caching far more than they can consume. However, the vagaries of pinyon seed planting by birds makes recolonization of burns erratic. On the edge of the Mojave Desert, Minnich observed burned pinyon stands in which no pine seedlings have appeared 10 years after the fire; in another, young trees were abundant 55 years after a fire. It may take centuries for one of these stands to again become vulnerable to fire.
Forest—Savanna Borders, Central America
(Kellman 1979; Kellman and Miyanashi 1982; Parsons 1955)
In Central America, many borders between forest or woodland and savanna appear to be in equilibrium; some are evidently controlled by topography or geology. On other borders no such controls are evident, and the pattern may be changing through time. It has often been suggested that in the humid tropics, soils deteriorate through time by leaching and laterization. This could cause a long drawn-out succession from forest on younger, more fertile soil through open woodland to savanna on senile soil. Frequent grass fires, which generally die out under the forest, would accentuate the nutrient, humus, and moisture deficiency of the savanna soils and thus sharpen the vegetational border. Conversely, cessation of burning might allow advance of the trees.
The Miskito Coast has islands of hardwood forest on the same kind of quartz sand and gravel that underlies surrounding savanna. Parsons (1955) suggested that these forests may be remnants of more extensive forest cover that burned during exceptional drought years or after being blown down by
hurricanes. Woodsmen working in the area believe the hardwood forest is in the process of expanding and recolonizing where savanna fires are suppressed.
Kellman (1979) suggested similar recurring fluctuations in woodland—savanna borders in Belize. Once converted to savanna and repeatedly burned, the soils are too infertile to support establishment of tall forest trees, even if fires are suppressed. Savannas can, however, be colonized by scattered populations of small, fire-tolerant trees and shrubs, such as nanche, Byrsonima crassifolia . These create microsites enriched in soil nutrients, which may provide nuclei for a second-stage invasion of savannas by forest trees.
Intercontinental Invasions, America and Australia
(Capeheart et al. 1977; Myers 1983; Westman et al. 1975)
The paperbark tea tree, Melaleuca quinquenervia , is native to the margins of freshwater swamps on the sandy coastal plain of Queensland and New South Wales. The trees are usually inundated by as much as 1 m of water during the wet season. In a dry season fire, the inner layers of spongy, moist bark insulate the trunk, but the loose, dry outer bark is flammable and carries fire into the canopy. After a fire, the serotinous capsules in the scorched crown open and release seed; a single tree can release over 20 million tiny, wind-dispersed seeds. The crown later resprouts.
This Melaleuca was introduced in the early 1900s to both the east and west coasts of southern Florida. It soon escaped from cultivation to become the dominant weed tree of extensive areas disturbed by logging and other human activities. It invades natural vegetation only in the kind of habitat it occupies in Australia, namely, the sandy margins of swamps that are flooded in one season and burned in another. Following a late dry season fire, massive amounts of seed are put on the ground at the most opportune time for germination. Thus, the Melaleuca develops dense stands along the swamp margins, excluding seedlings of pines and other native fire followers.
Baccharis halimifolia is a shrub native to the Atlantic and Gulf coasts of North America, where it grows on coastal foredunes and in freshwater swamps. It is abundant in Florida in swamps now dominated by Melaleuca and along sandy shores where another Australian tree, Casuarina equisetifolia , is naturalized. This Baccharis became naturalized in Queensland about 1900; by 1970 it had spread along the coastal plain of Queensland and New South Wales from about 23° to 31°S latitude. Much of its spread was as a weed of artificial habitats, but it is also invading Melaleuca swamps. A single Baccharis shrub may produce over a million seeds, which are widely dispersed by wind and water. Like Melaleuca, Baccharis is well adapted to survive seasonal flooding and drought; it is able to resprout rapidly after fire.
Comment
The extensive literature on fire and vegetation contains remarkably little evidence that natural fire regimes involve dynamic species distributions. Except in extreme climates—desert, tundra, and rain forest—recurrent fire has generally been a normal part of terrestrial ecosystems throughout their evolution. Adaptations allowing plants or their seeds to survive in burns have obviously been strongly favored by natural selection. They have evolved over the whole spectrum of growth forms, from tiny annuals to the largest trees. Many plant species do not merely tolerate fires; they exploit fires to build up their populations. For example, various hardwood and conifer trees follow the Melaleuca pattern. They retain seed for years in capsules or cones that open after the fire. Such plants that can stake the first claim to burns clearly have an advantage over those that reinvade burns from outside.
The few exceptional cases of reinvasion noted above all involve marginal populations: trees at timberlines, on savanna borders, or in the case of pinyon, on the humid margin of its primarily desert range. The ecological adaptations of all these species are primarily shaped elsewhere.
In the cases of California chaparral and montane conifers, it was suggested that a few species do not reproduce in fresh burns but rather in later successional vegetation. These are rather weak examples of a relay floristics model, in contrast to the initial floristics model more characteristic of fire-perturbed systems.
The recent cases of intercontinental migrations simply demonstrate again, as has been noted for other habitat types, that species may be barred from parts of their potential areas by dispersal limits.
Treefalls And Windthrows
Central American Rainforests
(H. Baker 1983; Brokaw 1985; Estrada et al. 1984; Hartshorn 1980, 1983; Herwitz 1981; Lang and Knight 1983; J. Whitmore 1983; T. Whitmore 1982; Williamson 1983)
Treefalls have been studied in two areas of Costa Rica and one in Panama in regions that have no history of hurricanes or forest fires. Gaps were generally initiated by fall of individual trees, often during torrential rains; whether the trees were senile, diseased, or overloaded with epiphytes is unknown. Some large trees took neighbors down with them or left weakened
neighbors that fell later; gaps resulting from these group falls sometimes exceeded 0.1 ha in area.
In the Atlantic lowlands of Costa Rica, the Organization for Tropical Studies field station, La Selva, contains a large stand of mature forest. The canopy is strongly dominated by a single species, Pentaclethra macroloba , a slow-growing legume tree. Its toxic seeds are scattered as far as about 10 m from the tree by explosive dehiscence of the pods; they must occasionally be carried farther by flowing water. Pentaclethra needs no gaps in the canopy for seed germination or for growth to maturity. However, in Hartshorn's study area, Pentaclethra shares the canopy with over 100 other tree species, about 75% of which Hartshorn classified as shade-intolerant, gap-requiring species. The study area also contains about 200 subcanopy species, of which about 30% are classified as shade-intolerant, gap-requiring species. Unlike Pentaclethra , nearly all the other shade-tolerant trees at all levels—canopy, subcanopy, and understory—are adapted for dispersal by birds, bats, and other animals. Most of the shade-intolerant species are also animal dispersed, but about 15% are wind dispersed.
Riverbanks and clearings in the region of La Selva have abundant populations of wide-ranging neotropical pioneer trees, for example, Ochroma lagopus, Trema micrantha, Cecropia obtusifolia , and Ceiba pentandra . All produce abundant seed that is carried long distances by birds, bats, or the wind and lies dormant in the soil awaiting disturbance to trigger germination. None are important in treefalls in the study area. Cecropia seedlings develop only in unusually large gaps, and an average of only two or three Cecropia trees per hectare are present in the forest; being dioecious, their reproductive success must be low. Cecropia, Ochroma , and Trema do not grow very tall or live very long. Ceiba develops into a towering emergent that can survive indefinitely above the canopy; nevertheless, it is uncommon in mature forests at La Selva.
Treefalls at La Selva are colonized mainly by forest trees that specialize in colonizing small openings with insufficient sun and bare mineral soil for extreme pioneers. The chronology of development of the gap trees is not well known. Some may have been present before the treefall as shade-suppressed juveniles or as dormant seed; others may seed in after the gap opens.
Corcovado, a Costa Rican national park on the Peninsula de Osa on the Pacific coast, has a huge, floristically complex rainforest. Here, also, treefalls in a local study area are colonized by forest species, not by Ochroma, Trema, Cecropia , or Ceiba , although these are common in the region on riverbanks, on earthflows, and in clearings. One of the gap species here is Cryosophila guargara , an understory palm; suppressed trunkless juveniles are abundant under undisturbed forest canopy. After treefalls the palms shoot up slender trunks about 10 m tall and begin to flower. They continue to produce seed for many years after the canopy has closed over them.
The Smithsonian Institution's field station on Barro Colorado Island in Gatun Lake, Panama, has mainly secondary forest on land that had been cleared before the canal was built and the lake formed. Succession has eliminated most of the pioneer trees; some of their successors, mostly about 60 years old, are senescent. Treefalls occur at the rate of about one per hectare per year. Strong pioneers, including Cecropia, Ochroma, Trema , and Ceiba are present locally in riparian sites and clearings. Their seedlings appear in some treefall gaps on the island, but are successful only in the larger gaps. Recruitment in small gaps is dominated by species found in the adjacent forest as suppressed seedlings before treefalls. In other words, succession does not start over at the pioneer stage with individual treefalls.
The Universidad Nacional Autónoma de México's field station at Los Tuxtlas, Veracruz, includes a mature rainforest. Here Cecropia obtusifolia is abundant on the edges of the forest; over 30 species of birds and various other animals have been observed feeding on Cecropia fruits throughout the year. They continuously inundate the forest with seeds, which remain dormant until the soil is disturbed. Cecropia seedlings grow as fast as 3 m/year in a large gap, but rarely live more than 30 years before being shaded out. Large gaps at Los Tuxtlas are mainly produced by strong northerly winds during winter outbreaks of North American air masses. These nortes reach Costa Rica in a weaker form. However, the number of Cecropia trees at Los Tuxtlas and La Selva averages about the same, two or three per hectare. Evidently large windthrows at Los Tuxtlas are too infrequent to maintain populations of short-lived pioneer trees.
Maple—Beench—Hemlock Forests, Eastern North America
Brown and Curtis 1952; Canham and Loucks 1984; Curtis 1959; Spurr 1956)
Mature forests are rare relics in this region today. Before they were cleared, forests in the Great Lakes—St. Lawrence—New England region were kaleidoscopic associations of many hardwood and conifer species; composition varied greatly with site character and disturbance history. Mesic sites protected from fires by lakes or other topography sometimes escaped burning for a few hundred years, long enough for shade-tolerant trees to become dominant. The most important of these was sugar maple, Acer saccharum , followed by beech, Fagus grandifolia , and hemlock, Tsuga canadensis . Less shade-tolerant and less abundant but still widespread associates were yellow birch, Betula lutea ; basswood, Tilia americana ; and elm, Ulmus americana . In the absence of disturbance, sugar maple reproduces more prolifically than any of its associates; the theoretical climax forest would be a pure stand of
sugar maples. Succession is prolonged, however, because all the associates are very long-lived, and their shade-suppressed seedlings or root sprouts occasionally inherit a treefall gap. Also, treefalls provide microhabitats favoring hemlock and yellow birch reproduction; their seedlings and saplings grow best on old logs, stumps, and tip-up root mounds. Hemlock and yellow birch are concentrated in relatively wet mesic sites, where they are shallow rooted and liable to windthrow, which may initiate another reproductive cycle.
Scattered through these mature forests are occasional old individuals or groves of species not normally associated with sugar maple and lacking any representation in the smaller size classes. The most conspicuous of these is the white pine, Pinus strobus , which towers above the maple canopy like Ceiba above the tropical forest. Like Ceiba, Pinus strobus probably evolved primarily in riparian habitats, but is able to colonize other areas of massive disturbance. Other pioneer trees found as relics in mature forests include paper birch, Betula papyrifera ; large-toothed aspen, Populus grandidentata ; and black cherry, Prunus serotina . Their establishment probably dated from catastrophic but very local forest destruction by ice storms and windthrows, with spot fires common in the debris left by the storms. Colonization of local openings in the maple forest implies seed dispersal from beyond the forest.
The trees of all successional stages discussed above, whether shade tolerant or pioneer, are a mixture of species dispersed by the wind, birds, or other animals. None of them except Populus grandidentata , which has very light wind-borne seeds, appear to be adapted for long-distance dispersal and able to colonize openings in the maple forest.
In northern Wisconsin, the original land survey records from mid-nineteenth century report many large areas of complete canopy destruction by windthrow in the maple—hemlock forest region. Those recorded ranged from less than 1 ha to several thousand hectares. A few were evidently tornado tracks, but most were probably due to squall line thunderstorms. (A single such storm system recently destroyed thousands of acres of secondary forest in this region.) Assuming random distribution, the average interval between windthrows at a given site was about 1200 years, several times the life span of late successional trees. About 20% of the presettlement landscape would have been in forest less than 200 years old, in which late successional species were still present. The larger windthrows must have been susceptible to fire.
In 1938 in New England, a hurricane destroyed a tract of primeval forest at Pisgah, New Hampshire, owned by Harvard University. What ensued was unusual in that the site was not burned, logged, or cleaned up. Four years after the storm, the blowdown was still a tangled mass of crisscrossed heavy timber, mostly suspended several feet above the ground. Most of the soil was shaded and moist beneath the fallen boles. Tip-up root mounds exposed
mineral soil on about 8% of the area. After four growing seasons, lower parts of the area were dominated by hemlock and beech seedlings, suppressed under the canopy before the storm, which were already 3 or 4 m tall. There were also many new hemlock seedlings, but few of beech. Higher parts of the area were dominated by posthurricane seedlings, mostly yellow birch, paper birch, black cherry, and two species not mentioned above, red maple, Acer rubrum , and black birch, Betula lenta . No aspen seedlings were found. Exposed mineral soil was colonized mainly by paper birch, black birch, and black cherry. Although old, emergent white pines had been present before the blowdown, pine seedlings were uncommon in the blowdown and not likely to survive; they evidently require a more open site.
Comment
In very low latitudes where strong pressure gradients and cyclones do not develop, rainforest canopy openings are simply a normal part of forest biology. A rich flora of fast-growing, shade-intolerant trees has evolved that fills these small gaps; usually they are recruited from nearby in the surrounding forest. Extreme pioneers arriving from outside the forest occasionally colonize exceptionally large gaps, but they are mostly short lived and unable to maintain forest populations. An exception in this group is the huge, emergent Ceiba , which may sometimes reproduce within a forest by successive colonization of scattered large gaps.
In tropical areas subject to hurricanes, forest dynamics might be different, but studies of stands before and after storms are not available. In Mauritius, the hurricanes that severely damaged some beach vegetation (discussed in Chapter 1) damaged but did not blow down virgin montane rainforests. It seems likely that great hurricanes open some sites for pioneer colonization, but that their return intervals may be too long to sustain a forest pioneer flora.
In some ways, mesic forests of eastern North America are similar to tropical rainforests in dynamics. Canopy gaps maintain a special flora of forest trees less shade tolerant and more diverse than mature forest dominants. Pioneers from streambanks and other open habitats play minor roles in unusually large gaps, but their populations are maintained outside the forest.
3
Invasion And
Elimination In
Established
Vegetation
Competitive Invasion
Naturalized Herbs, California Grasslands and Deserts
(Bartolome and Gemmill 1981; Dwire 1984; Robbins 1940, Twisselmann 1967; Wester 1975, 1981)
Upland vegetation in California varies strikingly in vulnerability to exotic invasion. Chaparral and forest communities are remarkably impervious to invasion, even after fire. Innumerable shrub and tree species have been introduced to California from other Mediterranean climatic regions without naturalizing. For example, European natives widely planted in California gardens include Pinus halepensis, Quercus suber, Ceratonia siliqua, Spartium junceum, Myrtus communis , and Rosmarinus officinalis . Some of these escape in artificially disturbed habitats, but they do not displace native woody species. By contrast, California grasslands are now dominated by exotic grasses and dicot herbs that began immigrating with the beginning of Spanish colonization. Anderson (1952) aptly called California grasslands "transported landscapes." California also has extensive hybrid landscapes in which exotic
grasses and herbs grow beneath open native woodland and desert shrubs.
The popular explanation for this invasion is that the native grasses and other herbs evolved under light grazing and decreased or died out after introduction of European livestock; Eurasian species that had evolved under millennia of grazing pressure then moved into the vacuum. This may not be the whole story. The possibility deserves consideration that some of the invaders could have naturalized with or without change in grazing pressure. The impact of grazing is hard to evaluate because livestock ranching spread very widely before much was recorded about the vegetation. Also, interpretation is complicated by the fact that increased grazing reduces burning. Grassland burning was widely practiced by California Indians before the establishment of missions; if it had continued, it might have barred invading annuals that are not adapted to annual fires.
Wester (1975, 1981) presented evidence that in the southern San Joaquin Valley the plant invaders arrived before the livestock. Livestock ranching developed there after 1870. Some feral horses were present earlier, but probably did not have any general impact on the vegetation. Early nineteenth-century accounts by Spanish expeditions and mid-nineteenth-century accounts by John C. Fremont and others give a consistent picture. Away from marshes and riparian habitats, the country was normally very barren, with only scattered shrubs and little grass. In wet years, however, there were masses of spring wildflowers and other annuals, including filaree, Erodium cicutarium . This species is native to coastal sands and other open habitats in Europe; in the San Joaquin Valley, Fremont repeatedly noted it covering the ground like a sward, as it still does. At about the same time, George S. Torrey reported it as common in Sonora, New Mexico, and California. Filaree seeds are easily carried in fleece and probably arrived with the first flocks of sheep. Filaree seeds have been found in adobe bricks of some of the eighteenth-century California mission structures. They were probably rapidly dispersed away from the missions by the native fauna. Filaree seeds have become a leading food source for many native birds, kangaroo rats, other rodents, and harvester ants. Filaree commonly grows beneath native shrubs, not only in the San Joaquin Valley but in the Mojave Desert and other regions of California.
Some other European natives evidently had similar histories. Seeds of wild oat, Avena fatua , and burr clover, Medicago polymorpha , have been found in early mission adobe bricks. The species were noted in wilderness areas of the upper San Joaquin Valley before livestock ranching began there. Both are well equipped for dispersal in fleece and in fur of native fauna. Subsequent invaders from Europe include red brome grass, Bromus rubens , which arrived in the late nineteenth century and has displaced natives on light, sandy soils even where they are not grazed at all. Arabian grass, Schismus arabicus , was
first recorded in the San Joaquin Valley in 1935; it has since spread over hundreds of thousands of acres, mainly during drought years, when very little competition was offered by native herbs. Wester (1975, 1981) noted that, because of drought and fluctuations in rainfall, soils of this region are especially immature and subject to erosion. They provide naturally open habitats similar to disturbed habitats in moister regions.
In the Mojave Desert, Erodium cicutarium and Bromus rubens are extremely common. In drought years they grow mainly under Larrea and other native shrubs, but in wet years they carpet the open ground between shrubs. Their presence is not correlated with grazing by domestic livestock.
In moister parts of California, invasion of ungrazed grasslands cannot be documented because introduced livestock were ubiquitous before the flora was recorded. However, there are many once-grazed areas that have since become reserves from which livestock are excluded. The introduced grasses have generally remained abundant in these and in some cases have continued to displace native grasses. For example, the University of California's Hopland field station has a 500-acre biological study area from which livestock but not native fauna have been excluded for 20 years; permanent transects were set up to monitor changes in grass cover. Native perennial bunch grasses, particularly Stipa pulchra , were abundant at the outset; subsequently, they increased in density on some transects with protection from grazing. On other transects, the formerly abundant Stipa has been replaced by two exotic annuals, Medusahead grass, Elymus caput-medusae , and Italian thistle, Carduus pycnocephalus , both very recently introduced to California from the Mediterranean. Southwest of Hopland on the Sonoma County coast, livestock was removed from the prairie-covered terraces 20 years ago, when Sea Ranch became a private residential development. Over 90% of the extensive terraces along 10 miles of coast has been reserved as permanent open space; beginning 10 years ago, research has been conducted on response of grass species to cessation of grazing. The grassland community is a rich and complex mixture of native and exotic species, varying greatly in different kinds of sites. The most common native grass when the study began was hairgrass, Deschampsia caespitosa , a large bunchgrass that is much more tolerant of grazing than most natives. During the last ten years, hairgrass has been losing ground to two European perennials introduced to California in the nineteenth century: sweet vernal grass, Anthoxanthum odoratum , and velvet-grass or Yorkshire fog, Holcus lanatus .
Alpine meadows in the Sierra Nevada are commonly sprinkled with dandelions, Taraxacum officinale , native to similar habitats in the Alps. Dandelions were introduced to California as weeds in the late nineteenth century and have spread mainly in artificially disturbed habitats. In Sierra meadows, however, their presence does not appear to depend on disturbance.
Naturalized Annuals, Nevada and Montana Woodlands and Deserts
(Beatley 1976; Bond and Wallace 1941; Forcella and Harvey 1983)
Pyramid Lake, Nevada, has an island about 100 ha in size, which has never been grazed. During the Last Glacial, the lake level was higher and the island smaller. Its upper part is ancient, weathered volcanic rock; the lower part is mantled with recent, unweathered calcareous tufa deposited by the glacial lake. On the upper part, Eurasian annuals, Erodium cicutarium, Bromus rubens, and cheatgrass, B. tectorum , have moved in with an abundant native bunchgrass, Stipa speciosa . On the lower tufa-covered area, the same three exotics have been joined by other Eurasian annuals, Salsola tenuifolia and Bassia hyssopifolia , both chenopods that have migrated widely in North America, mainly as weeds. Here they are growing among native halophytes: saltbushes, Atriplex spp., greasewood, Sarcobatus vermicularis , and saltgrass, Distichlis spicata .
In deserts of south central Nevada, Beatley (1976) reported that Bromus rubens has become so integrated into undisturbed natural communities over so large an area that, were its history of introduction not known, it would be taken for a native. She noted that only a few other exotics, all winter annuals, have successfully invaded relatively undisturbed communities: Erodium cicutarium, Schismus arabicus, Bromus trinii, and B. tectorum ; the last is common in disturbed sagebrush stands but also occurs sparingly at higher elevations in undisturbed pinyon–juniper woodland.
In mountain forest regions of western Montana, Eurasian weeds, such as Bromus tectorum, Cirsium arvense, Melilotus alba, and Verbascum thapsus , are restricted to such places as roadsides and clearcuts. In lower elevation grasslands and open Pinus ponderosa woodlands, the same exotics move out into areas dominated by native grasses, including Festuca spp. and Agropyron spicatum . Grazing by livestock is believed to favor this invasion but not be fully responsible for it.
Reestablishment of Rhododendron Ponticum, Northern European Forests
(Cross 1975, 1981)
This evergreen shrub had a wide distribution in Europe before the Last Glacial. It is known as a fossil from Ireland to Austria and northern Italy to Greece. It survived the Last Glacial only on the Iberian Peninsula and in the Black Sea region. In the latter it grows, along with Ilex aquifolium , as an understory in deciduous hardwood forests dominated by Quercus, Fagus,
Castanea, and Tilia . It is not clear why Rhododendron did not reinvade northern Europe along with its associates in postglacial time.
The species was reintroduced to Britain as an ornamental in 1763 and has since been widely planted. It has escaped from cultivation in many places, including Ireland, the Scottish islands and highlands, Norway, Belgium, the Channel Islands, and France. A single bush can produce more than 1 million of the extremely tiny seeds a year. Seedlings commonly establish in heaths and other open sites and also under deciduous forest canopies. Although they do not succeed on level litter-covered microsites, they get started on windthrown logs, rock outcrops, or places disturbed by deer. The seedlings are very shade tolerant and compete successfully in the forest understory with Ilex aquifolium , which returned to northwestern Europe on its own. While Ilex is palatable to browsing mammals, Rhododendron is toxic and unbrowsed. In places, it forms dense, almost impenetrable thickets that shade out the native ground flora and seedlings of the canopy trees.
Forest Invasions by Pittosporum Undulatum , Australia and Abroad
(Gleadow 1982; Gleadow and Ashton 1981; Grubb and Tanner 1976; Pickard 1984)
This evergreen broadleaf tree has attractive white flowers, orange fruits, and sticky seeds that adhere to almost anything; they are probably dispersed by any vertebrate animal that contacts them. The native range extended over a wide expanse of southeastern Australia's coastal lowlands, from Brisbane nearly to Melbourne. The species tolerates a wide range of climatic and edaphic conditions; it grows as an understory in tall, open eucalypt forests and on sea cliffs and dunes.
The species recently began moving westward in Victoria, spreading about 200 km in a few decades. It is invading coastal bluffs and also wet and dry eucalypt forests, where it is reproducing under its own canopy and suppressing Acacia and other understory species. In places Pittosporum is accompanied by two small evergreen European trees, holly, Ilex europaeus , and cherry laurel, Prunus laurocerasus . The main vector is apparently the European blackbird, but it is unlikely that the native fauna was incapable of spreading it. Possibly fire suppression rather than a change in dispersal is responsible.
Pittosporum undulatum was introduced to Lord Howe Island in 1898, escaped from cultivation, and is still spreading in undisturbed forest there. It is also naturalized on Norfolk Island, Hawaii, and Bermuda and in South Africa. In the Blue Mountains of Jamaica, it escaped from the botanical garden at Cinchona and is spreading in apparently undisturbed montane forests. Two other invaders of Blue Mountain forests are Cinchona officinalis
and C. pubescens , natives of Andean cloud forests. They were brought to the Jamaican garden that bears their name as sources of quinine in the nineteenth century.
Exotic Invaders, Tristan da Cunha Grasslands and Heaths
(Groves 1981; Wace and Holdgate 1976)
Tristan da Cunha is a group of rugged volcanic islands that lies in the South Atlantic about midway between Africa and South America. The climate is cold, wet, and windy; the native vegetation is mostly tussock grasses, heath thickets, and dwarf tree ferns. The native flora included about 60 seed plant species, mostly endemic. Discovered by the Portuguese in 1506, the islands were occasionally visited by sealers and whalers but were not settled until 1810. Potato farming, sheep pasturing, and other human activities have altered the vegetation on parts of the islands, but none of the native flora are believed to be extinct. About 120 alien species have been added to the flora, mostly established as weeds in fields, pastures, and settlements. A few species are naturalized on lava flows, scree, and beaches, and a few have invaded natural grassland and heath.
Nightingale Island is the least affected by human activities of the Tristan da Cunha island group. It lacks potable water and has been only briefly visited, usually to exploit penguins and other seabirds. No alien mammals or birds have ever established themselves there, but several alien plants are naturalized, including weedy European herbs, such as Holcus lanatus, Rumex obtusifolius, and Sonchus oleraceus . A more aggressive invader is New Zealand flax, Phormium tenax , a coarse monocot. In New Zealand it is a common pioneer of seacliffs and coastal dunes and also grows inland in a variety of heath, bog, and rocky sites. Phormium was introduced to the Tristan da Cunha islands about 1910 as a fiber source and windbreak. On Nightingale Island it has spread widely in heath and tussock grassland. Starting in 1968, attempts have been made to eradicate it because it was threatening to alter the nesting ground of great shearwaters; Nightingale Island has the world's greatest breeding population of these birds.
Exotic Invaders, Mauritius and Seychelles Forests
(Lorence and Sussman 1986; Sauer 1967; Vaughan and Wiehe 1937, 1941)
The native biotas of isolated oceanic islands have long been recognized as impoverished and disharmonious because many potential colonizing species
are screened out by lack of natural dispersal. These biotas are thus expected to be vulnerable to human-introduced invaders. Cases are well known of massive replacement of island endemics by aggressive introduced species. However, it is usually hard to tell how successful the exotic plants would have been in competition with the natives if there had been no disturbance of the habitat either by direct human agency or by introduced livestock. This question is especially hard to answer when islands were colonized by prehistoric human settlers, as in the Pacific Ocean from New Zealand to Hawaii.
Indian Ocean islands that were first settled in modern times offer less problematical cases of competitive invasion. In Mauritius, some rugged and remote regions have remained crown land through the colonial period and were never cleared or pastured. In the southwestern highlands above the Black River gorges, one of these areas was set aside in 1944 as the Maccabe Forest nature preserve. It is an evergreen rainforest dominated by a mixture of endemic trees as peculiar as the dodo. It is of only medium height because of the acid, wet soil and cool temperature, but the tree canopy is dense and appears to be a completely fully stocked stand. However, it has been massively invaded by exotics, mainly guavas (Psidium spp.). In their native home in tropical America, guavas are weedy pioneers in open habitats. Both the common guava, Psidium guajava , and the strawberry guava, P. littorale , were introduced to Mauritius gardens in the eighteenth century. The seeds were widely dispersed by birds. During the nineteenth century the species became widespread on the island. Vaughan and Wiehe (1937, 1941) were unable to find sites in the Maccabe Forest that had not been invaded by guavas. During the 1960s, the government attempted to control guava invasion of this forest by cutting and removing saplings and seedlings individually. However, Lorence and Sussman (1986) found heavy guava infestations in native forests in the same region of the island as the Maccabe Forest.
The Seychelles archipelago was first colonized by the French, who arrived from Mauritius in 1770. Two years later a royal spice garden was established on Mahe Island, and clove, nutmeg, black pepper, and cinnamon trees were planted. The French were hoping to break the Dutch spice monopoly, and the Seychelles garden was intended to be secret. The young trees were destroyed in 1780 to prevent discovery by an anticipated English raid, which never took place. By then, however, birds had spread seeds of Cinnamomun zeylanicum from the garden into natural habitats on the rugged granitic islands. The species thrived and multiplied, its high commercial value not being recognized until after 1900, when heavy exploitation of the stands began for export. However, it resprouts vigorously after cutting and remains a common permanent member of the flora in both artificially modified and remote natural habitats.
Comment
As expected, the clearest cases of competitive invasion of apparently closed stands of natural vegetation are on oceanic islands. Continents have some cases of invasion of undisturbed upland vegetation, but mainly confined to open types such as semiarid grasslands and deserts. However, the invulnerability of apparently closed communities may not have been thoroughly tested. Both natural and human dispersal have inoculated the world far more thoroughly with seed of pioneer species than of late successional and climax species.
A case of forest invasion that does not fit stereotypes of succession involves Monterey pine, Pinus radiata . In its native region on the California coast, it is a narrow endemic, but it behaves as a pioneer in both primary succession on dunes and secondary succession in clearings and burns. In Australia, where it has been extensively planted as a timber tree, it has invaded native forest. For example, in the Brindabella Ranges in the Australian Capital Territory, Burdon and Chivers (1977) studied the contact zone between a 43-year-old pine plantation and an adjacent mixed Eucalyptus forest with an Acacia understory. The native forest was ancient and apparently quite undisturbed, but had an understory of volunteer pines for a distance of over 150 m from the forest edge. The understory pines had begun reproducing by the age of 12 years and were surrounded by clusters of second-generation seedlings, which were competing successfully with the Eucalyptus seedlings and accelerating the invasion.
Selective Elimination
Castanea and Ulmus , North American Forests
(Anagnostakis 1982; McCormick and Platt 1980; Strobel and Lanier 1981)
As in many genera of northern hemisphere trees, Castanea and Ulmus are represented by different species in eastern North America, eastern Asia, and Europe. The Chinese chestnut, C. mollisima , and the Chinese and Siberian elms, U. parvifolia and U. pumila , evidently coevolved with parasitic Ascomycetes, the chestnut blight, Endothia parasitica , and the Dutch elm disease, Ceratocystis ulmi . Both fungi invade wounds in the bark and their mycelia grow through the living vascular tissues. On the Asiatic host trees,
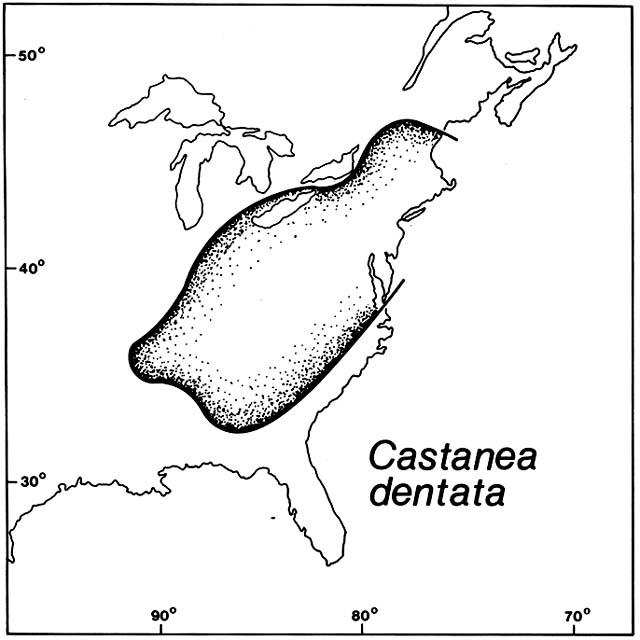
Figure 5. Castanea dentata . The former range of the North American Castanea is shown here before it was
eliminated from the forests by the chestnut blight (adapted from Stevens, 1917). This fungus evolved as a mild
parasite of the Chinese chestnut, following the rule that a successful parasite does not exterminate its host. It
did not follow this rule in North America where it arrived in 1904 with imported Chinese chestnut trees. Its
windborn spores rapidly infected the whole American chestnut population.
the symptoms are mild; on congeneric hosts elsewhere, infection with the fungi is catastrophic.
Chestnut blight arrived in New York in 1904 on Chinese chestnut trees imported as ornamentals for planting in the Bronx Zoological Garden. The spores of the fungus are wind-borne and soon infected the native Castanea dentata , an important member of the hardwood forests over several million hectares of the eastern United States (fig. 5). The tree was valued as a fine hardwood timber, for tanbark, and for the edible nuts. When the trees began dying back on a grand scale, expensive efforts to control the disease were begun. It soon became obvious that there was no hope of stopping the spread of the fungus throughout the range of the host. Attempts to transfer resistance from the Chinese to the American chestnut by standard hybridization and breeding methods were frustrated by the fact that resistant hybrids were ecologically different from their American parent. If introduced to the forests, they would have competed with other native hardwoods rather than reoccupying the chestnut's niche.
Castanea dentata is not extinct in eastern North America because the root systems of trees that have died back continue to send up sprouts, but these are reinfected and killed back again before reaching tree size or reproducing.
Chestnut blight arrived in northern Italy in 1938 and soon spread widely on the European species, Castanea sativa . The situation differed from that in North America in that European chestnut trees are generally planted, commonly as regular orchards, rather than being members of a natural forest. The species was evidently introduced westward from its southeastern European homeland during classic Roman times.
Beginning in 1951, some of the European chestnut trees that had been diseased began to recover; evidently the fungus was being attacked by a disease of its own that reduced its virulence. Research is now aimed at practical methods of spreading this viruslike disease of Endothia and perhaps saving both Castanea sativa and C. dentata .
Dutch elm disease caused by the fungus Ceratocystis , arrived in Europe, by unknown means, before it reached North America. There are several susceptible Ulmus species in both regions. Ceratocystis was first noted in the Netherlands in 1919, soon after in France and Belgium, and by 1927 in England. The fungus is spread by bark beetles of various kinds and thus moved more slowly than the wind-borne Endothia .
Ceratocystis arrived in the eastern United States in the 1930s on elm logs imported from Europe. The native elms are important dominants in floodplain and other moist forests over a huge area. Also, Ulmus americana had been planted over an even wider area as the favorite of all street trees. By 1950 rural and urban elms were dying wholesale from Quebec and Vermont
west to Illinois and south to Virginia and Tennessee. Spread of the fungus was slowed in some urban areas by expensive removal of diseased trees and constant spraying of beetles, but nothing could stop the mortality of forest elms. During the 1950s and 1960s, the fungus spread northeastward into Maine, southeastward into the Deep South and westward to the range limits of wild elms in the High Plains. Beyond there, it spread in town elms through the northern Rockies, reaching Oregon in 1973, northern California in 1975, and southern California in 1978, the end of the trail of elms planted by nostalgic emigrants from the east.
There is some hope of developing biological control of Ceratocystis by injecting elms with cultures of a bacterium antagonistic to the fungus, but this is more likely to save street trees than to bring back the natural stands; their roots are dead, not resprouting like chestnuts.
Panax , Eastern Asia and Eastern North America
(Emboden 1972; Price 1960)
Ginseng, Panax schinseng , has been a legendary plant in Chinese herbal medicine for millennia. Many wonderful powers have been attributed to its root, and it has generally commanded higher prices than any other herb in earth history. The species is native to the shady floor of mature hardwood forests of temperate eastern Asia. Even where uncleared relics of such forests survive, the ginseng is extinct in the wild over much of its native range. However, it has been taken into cultivation, particularly in South Korea, where tens of thousands of persons are currently employed in its production.
A related species, Panax quinquefolia , is native to undisturbed hardwood forests of eastern North America. Early in the eighteenth century, French Jesuits with experience in China organized exports of the American ginseng to China from Canada. Later, ginseng from New England became one of the most valuable cargoes the Yankee clippers had to trade with China. The roots were originally dug mainly by frontiersmen on the fringe of settlements. Later, they were dug from remnant forests, especially in the Appalachians. Not in law but in fact, wild forest ginseng became the property of the finder, regardless of whether it grew on private land, in national parks, or in nature reserves. Some root diggers left young plants and seeds for future harvest, but the species is extinct in much of its former range. Prices have continued to rise, recently to nearly $150/lb. In 1978, the U.S. Endangered Species Authority barred exports of wild ginseng from all states except Michigan, where digging was effectively controlled. Since then other states have started rigorous controls and resumed licensed exports. In 1979, U.S. exports of wild ginseng were valued at about $5 million. Meanwhile cultivation has
begun, mainly based on the Chinese species, which is reported to have been planted in natural forest habitats in the Blue Ridge Mountains once occupied by the American species. U.S. exports of cultivated ginseng in 1979 were valued at about $15 million.
Comment
There are other plants like ginseng that people have sought out individually because of their high value and have exterminated from parts of the original ranges. For example, for centuries extravagant prices have been paid for wood of two unrelated tropical trees, lignum vitae or gayac, Guaiacum officinale , and false gayac, Intsia bijuga . Their woods are among the strongest and hardest known and are also used as a cure for syphilis. Lignum vitae has been eliminated from some of its native dry West Indian forests. False gayac has been eliminated from some Indo-Pacific coastal forests. For example, in the Seychelles it is apparently extinct, and efforts at reintroduction have failed.
When a species is known to be rare, it is especially attractive to plant collectors, including academic botanists. In the Atlas of the British Flora, dots showing locations of very rare species are slightly displaced to frustrate collecting.
Conceivably, introduced insects might selectively eliminate plant species in their native regions in the same fashion that introduced fungi eliminated chestnuts and elms. However, no clear cases are known. The gypsy moth, introduced to Massachusetts in 1869, is still spreading in the United States. It prefers some host trees, for example, Quercus and Prunus , but it is not as specialized a parasite as some fungi. In 1981, the gypsy moth was credited with defoliating 10 million acres of North American forest, but the trees generally recovered and the ranges are apparently unaffected. Cases of elimination of introduced weeds by introduced insects are given in Chapter 4.
4
Artificially Modified
Habitats
Ruderal Vegetation
In the original Latin meaning, ruderals are plants that grow on ruins and rubble. The word will be used here in a broader sense to include weeds of dump heaps, urban wasteland, docks, pathways, railroads, roadsides, and other places heavily impacted by human habitation, industry, and commerce. The term is sometimes defined even more broadly to include all plant followers of humans or colonizers of artificially disturbed sites (Frenkel 1977). Agricultural and pastoral weeds, however, will be considered under other headings below.
Abandoned Villages, Aleutians and Greenland
(Bank 1953, Griffin and Rowlett 1981)
Archaeological sites can commonly be recognized, even in aerial photographs, by distinctive vegetation patterns. A clear example is found in Aleutian villages abandoned early in the nineteenth century when the Aleuts were casualties of Russian fur trade operations. The Aleuts had been primarily marine hunters and fishers with little impact on the tundra of the islands except in their coastal settlements. They built pit houses with stone and earth walls and sod roofs. Today the ruins bear a conspicuously different vegetation than the neighboring tundra. The dominant ruderals are lyme grass, Elymus arenarius , which is native to local beaches, and cow parnsip, Heracleum lanatum , which is native to streambanks.
In Greenland, also, there has been a micromigration of lyme grass from beaches to Viking and Eskimo ruins.
Maya Ruins, Yucatan
(Lambert and Aranson 1982)
Two useful trees, ramon, Brosimum alicastrum , and copal, Protium copal , are so conspicuous on Maya ruins that they have been suspected of being relics from Maya planting. The individual trees, however, are not that old, and it seems likely that the species colonized the sites after abandonment. They are native to nearby dry limestone outcrops; in the archaeological sites, they are concentrated on the steep sides of the highest ruins, built of the local limestone. Their roots often do extensive damage to the masonry.
Roads and Railroads, North America
(Anderson 1952; Baker 1972; Berthoud 1892; Blake 1956; Frenkel 1977; Gill 1973c ; Heiser 1978b ; Mühlenbach 1979; Robbins 1940; Semple 1983; Widrlechner 1983)
In North America, also, various native pioneers were evidently preadapted to exploit sites disturbed by human activities. For example, several annual sunflowers, Helianthus spp., grow wild in coastal sands of Texas, alkali sinks in the California and Nevada deserts, and sandy washes in the High Plains. Berthoud (1892) found sunflower achenes tangled in the coats of bison and the plants growing along bison trails. Some sunflowers spread prehistorically as Indian camp followers. One of these, H. annuus , has a story too complex to discuss here; it involved evolution both as a ruderal and as a domesticate. Helianthus petiolaris has a simpler and more recent migrational history. Until the nineteenth century, it was probably restricted to the High Plains in Colorado. Modern roads opened a pathway through the previously impenetrable vegetation of Kansas and Missouri. From Kansas eastward, H. petiolaris commonly grows in a nearly continuous file along roadsides, not straying into adjacent grasslands or cultivated fields. By 1900, it had crossed the Mississippi and reached Indiana, and by 1960, it had reached the eastern seaboard. Along the way, it became an urban ruderal.
Until 1925, another coarse herbaceous composite, Heterotheca camporum , was confined to prairies and open rocky glades of Illinois and neighboring states. Since then it has spread along roadsides, moving southeastward into Virginia after 1960.
An annual amaranth, Froelichia gracilis , grows wild in open, sandy grasslands
from Colorado and Nebraska south to Chihuahua, and Texas. Until 1920 it was unknown east of the Mississippi; it has since spread, mainly along railroad tracks, into eight eastern states, including New York.
Railroads have been responsible for rapid, often leapfrogging spread of alien ruderals across North America. A dwarf snapdragon, Chaenorhinum minus , which grows wild on European coasts, has long been a widespread weed along European railroads. In North America, it was first recorded at two ports, where it probably arrived in ships' ballast: Camden, New Jersey, in 1874, and St. John, New Brunswick, in 1881. By 1900 it was appearing in scattered places inland, and it has since crossed the continent to both the Pacific and Gulf coasts, being established in hundreds of localities. In each locality, the first record was almost invariably along a railroad; later records have often been along streams that intersect railroads.
The common tumbleweed or Russian thistle, Salsola kali s.l., grows wild on the coasts of Europe and is also a common weed there. It was first recorded in North America in 1886 in South Dakota where flax seed imported from Russia had been sown. (More than one Salsola species may have been introduced to North America, but the species can only be distinguished by specialists and their migrational histories await untangling.) By 1895, Salsola has spread through 16 states and several Canadian provinces and had reached the Pacific coast. The initial spread was mainly along railroads, which simultaneously offered an open habitat and rapid dispersal. The plants are commonly caught in the trucks of the cars and tumble along behind the trains, scattering seed as they go. Salsola is now a familiar ruderal along highways over much of the continent, most abundantly in the Southwest. The California Division of Highways spends over $1 million a year to remove the tumbleweeds from roadsides and drains. Biological control with Eurasian insects is currently being attempted. In 1977 a Pakistani moth was released that is not supposed to feed on beets or native chenopods but only on Salsola and Halogeton , another Eurasian chenopod. The role of Salsola and Halogeton in overgrazed western rangelands is discussed below.
The total ruderal flora of the great railroad yard system of St. Louis, Missouri, has been intensively studied over a 20-year period by Mühlenbach (1979). He found more than 100 species that apparently arrived by train; these had not previously been found within Missouri, although the state is botanically one of the best known in the country. Prominent among the species found were grasses and composites that are common elsewhere in North America, both natives and Eurasian weeds. Most of the species were ephemeral, often disappearing for no apparent reason after successfully flowering and fruiting. The rarest ones, found as single individuals, ended up as pressed herbarium specimens.
Along northern California highways, Frenkel (1977) found that road shoulders had a very simple flora of cosmopolitan herbs, mostly annuals. The
nine most frequent species were all naturalized from Eurasia: Bromus mollis, B. rigidus, Lolium multiflorum, Poa annua, Polygonum aviculare, Spergularia rubra, Medicago polymorpha, Erodium cicutarium , and Plantago lanceolata . Most of these arrived in California during the Spanish and Mexican periods. Only the Spergularia is believed to have immigrated during the twentieth century. Frenkel noted that the limited number of temperate zone species adapted to road shoulders and pathways have spread to form a cosmopolitan ruderal flora in such widely separate regions as North America, Europe, Japan, and Chile. About half of these species have small, hard seeds that are tracked around in soil on feet and tires; most of the rest are adapted for wind dispersal or attachment to animals. None of the common ones have fleshy fruits suggesting internal transport of seeds by animals.
Intercontinental Migration of Pineapple Weed
(Baker 1972; Gill 1973c ; Hegi 1954; Salisbury 1961)
Pineapple weed, an annual composite, is apparently native to both northeastern Asia and western North America. Because of a problem in nomenclature, the species appears in botanical literature under the two names Matricaria matricarioides and Chamomilla suaveolens . Like Helianthus and unlike most composites, pineapple weed lacks adaptation for wind dispersal; the seeds are evidently carried in animal fur and on animal and human feet. Perhaps the species came from Siberia through Beringia to America with prehistoric people and their dogs. It was collected in Idaho by the Lewis and Clark expedition when the region was a wilderness. In the Mackenzie Delta of the Canadian Arctic, pineapple weed grows mainly on riverbank point bars. These are naturally open areas of accreting sandy beaches dotted through the general matrix of bog and forest. These point bars were the traditional campgrounds of Eskimo and Indian canoemen. It is not certain that presence of pineapple weed in such sites depends on human activity because the species is still present 30 years after the sites were last camped in.
Most of the pineapple weed population in western North America, from Alaska to Baja California, is concentrated along pathways and roadsides. On northern California roadsides, Frenkel (1977) found it to be the tenth most abundant species after the nine Eurasian exotics noted above. Its seeds are probably dispersed in mud on tires.
Pineapple weed was introduced to Europe through botanical gardens. It escaped from the Berlin garden about 1850 and was widespread on continental pathways and roadsides by the 1860s. It was introduced to Britain through Kew Gardens in 1869 and escaped within 2 years. Both on the continent and in the British Isles, it continued to spread during the first
quarter of the twentieth century until it became one of the most ubiquitous of all ruderals.
Salted Roads, Mine Dumps, and Bombed Ruins, Britain
(Bradshaw 1983; Matthews and Davison 1976; Salisbury 1943)
A bizarre micromigration was initiated by use of rock salt to melt ice on British roadways. Starting in 1973, halophytes from coastal salt marshes began spreading inland along roadsides, some moving 13 km within 3 years. Species included Suaeda maritima, Spergularia maritima, Plantago maritima , and Aster tripolium ; some are flowering and fruiting in their new sites.
Colonization of British mine and industrial waste dumps is partly by local ruderals but partly by immigrants from a distance. Some plants that pioneer on alkaline lime and soda waste dumps in northwestern England come from calcareous coastal dunes over 40 km away. Some are wind dispersed, such as a gentian, Blackstonia perfoliata , and an orchid, Anacampsis pyramidalis , and some are bird dispersed, such as restharrow, Ononis repens . Some kinds of waste dumps remain barren for centuries. On china clay wastes, there is gradual, orderly development of vegetation and soil, with increasing soil nitrogen evidently controlling the sequence of colonization.
Ruins of British towns bombed during World War II opened sites for population explosions of some ruderals, for example, the circumboreal fireweed or rose bay, Epilobium angustifolium ; the Eurasian groundsel, Senecio vulgaris ; and the Oxford ragwort, S. squalidus . All three have seeds dispersed long distances by the wind and are capable of producing astronomical numbers of seeds. Senecio squalidus is native to rocky places in the mountains of central and southern Europe. It was named by Linnaeus in 1753 from plants cultivated in the Oxford Botanical Garden, where it had been grown since 1699. By 1800 it began volunteering on walls in Oxford. As the railroad network developed in the mid-nineteenth century, the Oxford ragwort began moving along the tracks in clinker ash and stone ballast. By 1930, it was fairly widespread in southern England and some parts of Ireland. Since World War II, it has become one of the commonest ruderals in the British Isles, including the Channel Islands. Curiously, it is only locally present as a ruderal on the continent. The long delay in naturalization and gradual acceleration of its spread suggest evolution of an ecotype adapted to the new region. Also, S. squalidus is highly self-sterile, and the initial introduction may have been incapable of seed production.
The gradual spread of Senecio vernalis on the continent is in some ways a similar case. Senecio vernalis , like its congeners, has light, plumed achenes dispersed by wind. It is an annual, native to the steppes of central and
southern Russia. It moved westward into Lithuania in the eighteenth century, Poland in the early nineteenth, Germany in the late nineteenth, and France in the twentieth century. Its spread has been chiefly on rubble, embankments, and other so-called wasteland (Szafer 1966).
Weeds Imported with Wool and Grain, France and Finland
(Suominen 1979; Thellung 1912)
European botanists have long been drawn to places where imported wool, cotton, and grain were cleaned as good places to find novel exotic plants, sometimes new to science. A classic locale in the study of wool adventives is the French department of Hérault, in which Montpellier lies. Probably no other region of the world has been so carefully studied by plant taxonomists for so long. Since about 1600, a formidable roster of them has worked at the University and Botanical Garden of Montpellier. Thellung, who synthesized the records of adventive plants in this region, was unexcelled as a taxonomist of difficult weedy species.
Thellung (1912) listed 769 exotic species that had been found growing spontaneously in the region since the seventeenth century; many of these were described and named at Montpellier before they were discovered by botanists in their homelands. The richest single source was a wool yard at the Juvenal Gate, where imported wool had been washed for 200 years, starting in 1686. Thellung attributed the introduction of 526 species to seed cleaned out of the fleece. The homelands of the plants matched the source regions of the wool in different periods. Before 1830, nearly all the wool and the associated seeds came from the Near East and other Mediterranean regions. Starting in 1830, the source shifted to the Plata region of South America. Shortly before the wool yard closed, Australian wool and flora were imported. Following closing of the wool yard, the immigrants gradually died out until only 10 species survived there 25 years later. Only four of the wool immigrants had escaped the yard and established weed populations in the surrounding country. Thellung attributed the low rate of survival to climatic incompatibility, which does not seem to be a completely satisfactory explanation.
Of the total of 769 exotic species, 243 were not imported in wool, according to Thellung. He attributed their immigration to escape from cultivation, largely from the botanical garden, imported grain and fodder, ships' ballast, and railroad traffic. Over 100 of these species persisted as reproductive populations, mainly as weeds of artificially disturbed habitats. A disproportionate number of these were North American natives, for example, Phytolacca americana and Amaranthus retroflexus , discussed in Chapter 1.
In Finland, Suominen identified a total of 370 weed species as probably
introduced with imported grain. The study covers the period from 1851 to 1975, so it does not include the many weeds that arrived with grain from the Neolithic to 1850. A massive array of herbarium collections has been correlated, by 5-year intervals, with customs and other records of grain imports.
For a long time after 1850, new weed immigrants were mainly imported with rye and oats from northern Russia. The number of species rose sharply after each Finnish crop failure, when grain imports rose. The adventive weeds grew mainly in the vicinity of flour mills or mill dumps; some grew along railroads and almost none in seaports. Changes in geographic distribution of new ruderals during World War I were correlated with new rail connections and Russian troop movements. During World War II, German troops in Finland were supplied through certain harbors, which temporarily sprouted new immigrant weeds native to central Europe. After World War II, a new set of weeds arrived from the Soviet Union as wheat became the main grain imported; these weeds came from more southern and drier regions than the oats and rye weeds. At the same time, another new set of weeds arrived with large maize and soybean imports from the United States. Most of these were North American natives, such as Datura stramonium, Iva xanthifolia , and Ambrosia artemisiifolia , and some were European weeds naturalized in America, such as Abutilon theophrasti . Imports of Argentinian maize and Australian wheat brought mainly naturalized European weeds, not natives of the southern hemisphere. A local outburst of southern European weeds was tied to a particular shipment of Turkish oats. After 1953, imports of Chinese soybeans brought Setaria faberi , a weedy grass common in fields in China.
The vast majority of the colonies were ephemeral and confined to the mill dumps and other spots where the imported seeds landed. None of the immigrants from southern Europe or overseas were able to reproduce under Finnish climate and day lengths. The minority of new immigrants that did reproduce were almost all from Russia. These did not generally become field weeds but rather remained strictly ruderal, commonly in urban sites with warm, dry microclimates and nonacid soils. Among these precarious immigrants are species that are less demanding weeds in lower latitudes, such as Solannum nigrum, Hyoscyamus niger , and Sonchus asper .
Comment
By definition, ruderal habitats are human creations, but the importance of human seed dispersal in migrations of ruderals is highly variable. It was probably irrelevant in occupation of Aleutian, Mayan, and British ruins. In
the cases of roadside and railroad weeds, human dispersal may have merely accelerated the rate of migration. It was crucial for overseas migrations. Such long-range dispersal has often resulted in ruderal colonies that are unable to maintain themselves. It is commonly considered a trivial pursuit for botanists to record such ephemeral colonies. If the roles of dispersal and environmental selection in plant migration are to be properly evaluated, however, a balanced record of failures and successes is needed. If only successful colonization is considered, it is easy to forget the role of environmental selection and to assume that species are absent from distant regions because they have been unable to get there. Moreover, when a botanist finds a new immigrant, whether it is at a dead end or the founder of a great invasion may be unpredictable.
Ruderal populations have sometimes served as migrational bridges between other habitats, including natural seashore and riparian habitats where many ruderal species originated. For example, Amaranthus rudis , native to stream banks of the south-central United States, began appearing in European ports and railway yards as a trivial ruderal about 1950. It has since moved from Italian railways into natural riparian habitats along the Po River.
Weeds Of Cultivated Ground
Transcontinental and Intercontinental Migrations of Amaranthus
(Sauer 1967a )
Amaranthus is a cosmopolitan genus of annual herbs that includes about 50 species native to stream banks and seacoasts of many temperate and tropical regions. Some of the New World species became especially successful and widespread as agricultural weeds; the migrations of three of these will be sketched here. These three species are native to regions where maize was cultivated before Columbus arrived in America. In traditional American Indian agriculture, maize was interplanted with other crops in milpas, which were treated more like gardens than like Old World small grain fields. No weed seeds rode along on maize ears selected individually for seed grain, which was hand planted in hills that were hoed and hand weeded. Nevertheless, amaranths thrived and spread as milpa weeds. Farmers tolerated them as favorite sources of potherbs and edible seeds and eventually domesticated some amaranths as ancient grain crops, a story that is not relevant here. A healthy amaranth plant produces thousands of seeds, which in the weed
species, germinate gradually over decades whenever the soil is disturbed.
Amaranthus hybridus s.l. (including A. quitensis ) grows wild on riverbanks from eastern North America through the highlands of tropical America to temperate South America. It very likely became a milpa weed in Guatemala or Mexico when maize was first domesticated. It did not accompany maize to Europe in 1493, but finally arrived by means unknown in the early eighteenth century. During the nineteenth century, it became an abundant weed in southern Europe and northern Africa mainly in agricultural land, including maize fields; occasionally it escaped to river banks. North of the Alps, it has appeared repeatedly as an ephemeral ruderal but does not reproduce there. About 1900, A. hybridus appeared in California, South Africa, the Far East, and Australia and has continued to spread as an agricultural weed. In New South Wales, it has become much more abundant since 1960.
Amaranthus retroflexus grows wild on streambanks in eastern North America, as noted in Chapter 1. It is also a common weed in floodplain maize fields. In the mid-eighteenth century, Peter Kalm sent seeds from Pennsylvania to Linnaeus; a specimen grown at Uppsala, the type for the binomial, is still preserved in the Linnaean Herbarium in London. Linnaeus may have been responsible for inoculating Europe with the species by sending seeds to various botanical gardens. Lamarck noted it had escaped at Paris by 1783. By 1800, it was a common agricultural weed throughout much of the continent. Collections in major European herbaria show that during the nineteenth century, A. retroflexus spread as far as Norway, Portugal, the Azores, Algeria, Greece, Turkey, Syria, Astrakhan, and Afghanistan. Recently, Conard and Radosevich (1979) noted that maize yields in Italy and Austria were being seriously reduced by infestations of A. retroflexus that had developed atrazine resistance due to overuse of the herbicide. About 1900, A. retroflexus appeared in California and since then has arrived in China, Japan, and Australia. In New South Wales, it has become widespread since 1950.
Amaranthus powellii (including A. bouchonii ) grows wild in arroyos and desert washes in the Cordilleran system from western North America to the Andes. It was also a prehistoric milpa weed. In California and other far western states, as agriculture expanded in the nineteenth century, A. powellii became the most common weed amaranth in the region. It began to spread east of the Rockies about 1900 and has become a troublesome weed of maize and soybeans in the U.S. corn belt since 1940. Overseas, it showed up sporadically from the 1890s through the 1920s in Europe at scattered places from Scotland to Spain and from Sweden to Austria. For a long time, it remained a ruderal around docks, wool waste dumps, tanneries, grain elevators, and railroad yards. Since 1930, it has become a common weed in maize, potato, and other crops in the British Isles and on the continent; it reached Turkey after 1960. Meanwhile, A. powellii appeared in Australia, New Zealand,
and southern Africa in the late nineteenth century; only recently has it become abundant there. In East Africa, it first appeared in 1949 as a maize weed in Kenya. It invaded southern India after 1960.
Comigration of Cereal Crops and Weeds to California
(Barrett 1983; Robbins 1940)
Old World small grain agriculture, since its Neolithic origins, has depended on mass harvesting and sowing of seed from a mixed population of more or less domesticated cereals and associated weeds. Spanish wheat fields contain such mixtures today. During the colonial period, Spanish weeds inevitably went along with wheat to the New World. Eventually, perhaps via both Mexico and Chile, Spanish wheat and weeds even reached California. The eighteenth-century adobe bricks of California missions contain seeds of black mustard, Brassica nigra , and other European weeds that probably were accidentally carried in the seed wheat. Curiously, the red poppy, Papaver rhoeas , one of the most common Spanish grainfield weeds, has not naturalized in California wheat fields, although the species is grown as an ornamental.
Rice cultivation began in California with seed introduced from Japan in 1912–1915. Various Japanese rice weeds still grow around the Sacramento Valley experiment station where rice was first planted. Some of these weeds have not spread from there. Two species of barnyard grass, Echinochloa oryzoides and E. phyllopogon , which evidently came with the imported rice seed, have spread as major weeds throughout California rice fields. A close relative, E. crus-galli , also native to Asia, arrived in California earlier; it has been a common weed there since the mid-nineteenth century in various crops. Echinochloa oryzoides and E. phyllopogon are almost entirely confined to flooded rice fields. They are believed to have evolved as weed mimics of rice in ancient paddies. Hand weeding caused any genetic changes that made the weed harder to eliminate from the crop to be favored by unintentional selection.
Both species now have seedlings that look almost identical to rice seedlings; both flower and mature their grain synchronously with rice. The seeds are not as heavy as rice but are two to three times as heavy as ordinary E. crus-galli seed. They resemble rice closely enough to pass inspection in certified seed. Both species produce seed with weak dormancy that germinates fully along with planted rice, unlike E. crus-galli . Also like rice and unlike E. crus-galli , the weed mimics are adapted to conduct O2 from the air down to flooded root systems. In retrospect, immigration of these weeds could have been barred by meticulous seed sorting, but they passed routine screening.
Sequential Migration of Glycine and Setaria from China
to North America
(Fairbrothers 1959; Pohl 1951)
A species of bristlegrass, Setaria faberi , noted above as having arrived in Finland with soybeans imported from China, arrived in North America long after the crop. Soybeans were introduced repeatedly from 1765 to 1900, but did not become an important crop until World War I when a phenomenal expansion began that culminated with their regular rotation with maize throughout the U.S. corn belt.
The first record of Setaria faberi in North America was on Long Island, New York, in 1925. During the next 20 years, it spread east of the Appalachians along railroads and roads and in scattered urban sites from New England to the Carolinas. For a long time, it was not recognized as distinct from a related weed, Setaria viridis , which had arrived long before.
Setaria faberi ceased to be a minor ruderal and became a major agricultural weed when it finally reached the corn belt. It was first recorded in Illinois in 1941; by 1950 it was exceedingly abundant across Illinois and Indiana, frequently blanketing soybean fields so densely that the crop could hardly be seen. It was first recognized in Iowa in 1949, and by 1950 it was in 36 Iowa counties and had spread to neighboring states. It grows as an annual in both phases of the standard crop rotation, but is more of a problem in soybeans than in the taller maize.
Comment
Human dispersal of weed seeds may be irrelevant in some situations, as where riparian species are preadapted to colonize cultivated ground within their natural seed shadows. Human dispersal was obviously crucial in cases of weed migration overseas, as in the case of Setaria faberi , and weeds have commonly gone abroad as stowaways in shipments of grain or cotton. Another pattern, not discussed above, is escape of a deliberately introduced crop to become a noxious weed. For example, Tientsin jute or velvetleaf, Abutilon theophrastii , was a fiber crop, domesticated in Asia, which was introduced to the British colonies in North America. Before American cultivation was abandoned in the late nineteenth century, Abutilon had been widely planted by corn belt farmers. It survives there as a major weed of both maize and soybeans, resistant to herbicides and causing losses of hundreds of millions of dollars annually (Spencer 1984). Similarly Johnson grass, a variety of Sorghum halepense , has been widely planted in North America as a fodder crop and has escaped to become a troublesome weed in other crops.
In Central America in 1960, a Mennonite colony struggling to establish itself in Belize planted Johnson grass for the dairy cattle, setting in train a permanent weed problem. The seeds are spread by birds and cattle and invade new land as fast as the forest is cleared (Sawatzky 1971).
Regrowth On Abandoned Fields
Piedmont, North Carolina
(Billings 1938; Christensen and Peet 1981; Egler 1954; Finegan 1984; Keever 1950)
By the mid-nineteenth century, most of the Piedmont region east of the Appalachians was under cultivation for row crops and the rest was exploited for timber and grazing. The native pines and hardwoods retained their former gross ranges but only as patchy remnants. Thereafter, farms were progressively abandoned due to soil exhaustion and economic depression. Because of the drawn out chronology of abandonment, Billings (1938) and other workers were able to compare different ages of regrowth on otherwise similar sites to develop a model of secondary succession that has become a textbook classic.
The native flora and its seed bank in the soil had evidently been exterminated from the fields by long continued cultivation. The dominant species in the study area the first year after abandonment was European crabgrass, Digitaria sanguinalis , which had been present as an agricultural weed. Native pioneer herbs and trees, all with primary populations in riparian and other naturally open habitats, began to invade when their first seed ripened after the last cultivation. Native composites with wind-borne seeds built up populations quickly, first with annuals, such as Conyza canadensis and Ambrosia artemisiifolia , and next with perennials, such as Aster pilosus . By the third year, seedlings of a perennial grass, Andropogon virginicus , began to crowd out the composites. Meanwhile, yellow pines, Pinus echinata and P. taeda , rapidly seeded in, and by the fifth year the dense stand of pine seedlings began to shade out the pioneer herbs. Thus far, the sequence of invasion was evidently controlled by availability of seed rather than by a relay in which early arrivals prepared the site for following species. It was believed, however, that invasion by hardwood trees was delayed until about 20 years after abandonment, when the accumulation of pine litter and humus finally permitted Quercus seedlings to survive. Further development of canopy and soil were supposed to be prerequisite to entry of other hardwoods.
Later workers have suggested modification in the scheme of hardwood succession. In some places, the pines were accompanied from the start by wind-dispersed hardwoods, for example, Liquidambar styraciflua, Liriodendron tulipifera , and Acer rubrum . Various species of Quercus and Carya may also have colonized promptly if there was a nearby seed source. They may be absent from most young stands because of lack of dispersal. Transport of their heavy acorns and hickory nuts may await buildup of animal populations with forest development. Christensen and Peet (1981) suggested that abandoned fields are so fully stocked by rapidly arriving wind-dispersed trees, mainly pines, that more slowly dispersed species cannot invade until the pioneer forest begins to degenerate and thin out.
The process of hardwood forest regrowth in the Piedmont is becoming of mainly academic interest because of a general trend to plantation pine monoculture for pulpwood production.
Cat Island, Bahamas
(Byrne 1980)
Shifting cultivation has been practiced intermittently on Cat Island for about 1,000 years, with a hiatus for about 200 years after the original Arawak inhabitants had been exterminated by the Spanish. The present pattern developed during the British colonial period in the eighteenth century. Fields averaging about 0.1 ha in area are partly cleared in the evergreen scrub woodland covering most of the island; a few shade trees are left. After the slash is burned, many of the stumps resprout and much time is spent the first year removing the sprouts. More effort is spent on herbaceous weeds during the second and third years, after which the field is abandoned. Regrowth is usually allowed to develop over 15 years before reclearing, often over 30 years.
Byrne (1980) conducted an elegant study of shrub and tree regrowth on 300 former fields. The age of the vegetation was determined from successive aerial photographs and groundwork with local informants. The farmers distinguish three kinds of terrain: whiteland, blackland, and flatland, which Byrne identified, respectively, as Holocene beach ridges and dunes, Pleistocene beach ridges and dunes, and Pleistocene marine plains.
Within the first 15 years after abandonment, whiteland fields were invaded by about 15 woody species and the blackland and flatland by over 80 each. Nearly all the species are native to coastal and other naturally open habitats on the island. The only important old field pioneer that is an exotic is Leucaena latisiliqua , a mimosa-like shrub or small tree that was introduced from the tropical American mainland; on Cat Island it is common along
roadsides. A few of the early regrowth species probably survived clearance and cultivation, either vegetatively or as buried seed, but most evidently seeded in. All species are well adapted for local dispersal, mostly by wind, such as Gundlachia corymbosa , and a few by birds, such as Lantana involucrata .
From about 15 to 30 years after abandonment, some of the pioneer shrub species were replaced by taller shrub and tree species. Most of these were present in the early regrowth phase and simply increased in cover. Other tree species invaded only after the vegetation was more than 15 years old, including Metopium toxiferum on blackland and flatland and Coccoloba uvifera on whiteland. In Chapter 1 it was noted that the Coccoloba was slow to recolonize Belize cays where it had been dominant before being wiped out by a hurricane. These later invaders are bird dispersed.
Byrne (1980) pointed out the remarkable contrast between the very limited role of exotic plants here and their overwhelming invasions of disturbed habitats on some other islands. The Bahamas had a rich flora adapted to droughty, sandy, and rocky terrain that is geologically young and naturally disturbed by hurricanes, lightning fires, and dune formation. Evidently this flora was preadapted to exploit temporary human clearings.
Upper Amazon Basin, Peru
(Scott 1978)
Traditional Campa Indian agriculture continues in the Gran Pajonal region of eastern Peru. Unlike so much of the humid tropics, this region is not being converted to pasture for beef cattle. Campa Indian population is not increasing, and about 97% of their region is currently covered with semievergreen forest. Shifting cultivation involves small clearings in mature forest, which are burned during the short dry season and planted with maize, manioc, and a variety of other plants. Most of the forest species are eliminated from the milpas, if not by cutting and burning, then by pulling resprouts and seedlings. Some weeds germinate even before the rains, probably from seed that was dormant in the forest soil, for example, Phytolacca rivinoides and Passiflora coccinea . Both of the species are bird dispersed, and the Phytolacca is known to be capable of long dormancy until released by soil disturbance. Other weed species, largely wind dispersed, appear within a few months. Very abundant are bracken fern (Pteridium aquilinum ); grasses, including Imperata brasiliensis ; shrubby composites, including Baccharis floribunda ; and fast-growing trees, including Cecropia spp. All these pioneers have local populations on stream banks, anthills, or other naturally open sites and are not dependent on seed from other milpas.
After 2 to 3 years, the milpa is usually abandoned to the weeds, and Cecropia trees soon dominate with an understory of shrubs, mainly melastomes. The bracken and grasses are gradually shaded out. After 25 or 30 years, the Cecropia trees die out, and a few saplings of primary forest trees recolonize the area.
This cycle can be sidetracked and stalled if the abandoned field is burned annually. The Campa sometimes deliberately create new grassland patches on former fields by firing them each dry season. This initially favors the Imperata over other weeds and forest regrowth, but as the years go by, the stoloniferous Imperata is gradually replaced by Andropogon spp. and other bunch grasses. The new savanna is then invaded by fire-tolerant shrubs, particularly Byrsonima crassifolia , which is dispersed by birds. The Campa grassland—forest borders are extremely abrupt; the fires normally go out without penetrating the forest. Some bird-dispersed shrubs that are not adapted to either frequent burning or shade survive along the forest margin, including Lantana camara, Rubus urticaefolia , and various melastomes. The Campa do not keep cattle, and they value grassland patches mainly as settlement sites. Settlements migrate every few years, usually rotating among several sites on grassy ridges that offer a view, exposure to sun and wind, and escape from mosquitoes and snakes.
Where non-Indian settlers with livestock enter the region, the Campacreated grasslands are overgrazed and fire frequency and intensity are reduced. The old field succession then resumes; Baccharis floribunda and other wind-dispersed composites return, along with bird-dispersed shrubs and small trees, especially Lantana camara, Psidium guajava , and melastomes.
Tall Forest Regions, Philippines
(Brown and Mathews 1914; Conklin 1957; Holm et al. 1977; Kellman 1970)
Traditional swidden or shifting agriculture in the dipterocarp rainforests and tall seasonal forests of the Philippines relies on a long fallow period during which the forests substantially recover. Even in small, temporary clearings, the primary forest species are usually completely killed out by burning and weeding. During cropping, the weed flora consists mainly of grasses and other herbs. By the second year after abandonment, pioneer trees, mainly bird dispersed, are seeding in. The species are not those that colonize treefalls in mature forest. The seed must come either from wild stands on coastal, riparian, or landslide sites or from other abandoned swiddens. Common pioneer trees include Trema orientalis in the elm family and various species of Homalanthus, Macaranga , and Mallotus , all in the euphorb family.
All are soft wooded and rapid growing; they retain dominance for about 25 years before the reinvading primary forest trees take over.
Here again, a typical cycle can be shunted onto a sidetrack by repeated burning of the regrowth. This commonly begins in swiddens that have been invaded by cogon grass, Imperata cylindrica , and wild sugarcane, Saccharum spontaneum , species discussed above as common on Krakatau and in riparian sites in Malaysia. The abundant plumose seeds of these grasses may blow in the wind for long distances over land and sea, but they drop out of the still air under a forest and seldom find their way to isolated clearings. They spread easily between nearby clearings along paths and roads. Their aggressive growth and spread by tough rhizomes are among the main reasons for field abandonment. If ignited, these grasses burn fiercely even when green; their rhizomes survive while tree seedlings that would otherwise eventually shade the grasses out are incinerated. The fires normally go out right at the forest margin, even in regions with a dry season, so the burning itself does not cause advance of these artificial savannas into undisturbed forest. Imperata and Saccharum are often fired deliberately to maintain them as fresh forage for deer or cattle. Also, as human population pressure has risen and the swidden cycle has been shortened, accidental burning has become common and self-reinforcing. The spread of these artificial savannas has been partly channeled by topography; they are expanding most rapidly on ridges and hillcrests most exposed to fire.
Comment
Finegan (1984) noted that theories of forest succession, particularly on old fields, have suffered from a pendulum swing from one extreme to another, with positions based mainly on deductive logic rather than field observation. Rather than choosing between polar positions that the succession is controlled by either autogenic changes in the environment or by vagaries of seed dispersal, he suggests that a less inflexible theory would allow for the possibility of control by both. Both certainly need to be considered in accounting for the sequence of species invasion in the few cases discussd above.
Whatever factors control the sequence of colonization, there is no doubt that local migration is involved. Abandoned fields are invaded from outside rather than reclaimed by species surviving in situ from a previous cycle; this appears to be true of both the weedy pioneers and the late stages of forest succession. However, in long fallow-shifting cultivation where only a small percentage of the forest is cleared at a time, the pioneers may have to immigrate from farther away than the forest regrowth species.
Ecology textbooks usually lump old field regrowth under secondary succession together with regrowth after blowdowns, fires, and other natural
disturbances or perturbations. This makes secondary succession a messy concept because old field succession approaches a primary succession model in starting with a clean slate and requiring invasion, while the other natural kinds of secondary succession approach an initial floristics model with all the species present on the site from the outset.
Altered Rangelands
Cattle and Sheep, Great Basin of Western North America
(Burkhardt and Tisdale 1976; Christensen and Johnson 1964; Lanner 1981; Mack 1984; Madany and West 1983; Robertson 1971; Rogers 1982; Vale 1974, 1975; West 1983; Yensen 1981; Young and Budy 1979; Young et al. 1972, 1976)
The high, arid intermountain region of western North America has cold, snowy winters and hot, dry summers. Under original pristine conditions, salt flats in the valley floors, where they were not completely barren, had scattered greasewood, Sarcobatus vermiculatus , and other chenopod shrubs. Vast areas on lower slopes around the basins were dominated by less extremely halophytic chenopod shrubs, for example, shadscale, Atriplex confertifolia ; wing-scale, A. canescens ; and winterfat, Ceratoides lanata . At slightly higher levels, big sagebrush, Artemisia tridentata , dominated tens of millions of hectares; it was commonly accompanied by rubber rabbitbrush, Chrysothamnus nauseosus , and other shrubs. At still higher elevations, big sagebush was joined by junipers, and still higher, by pinyons. Juniperus osteosperma and Pinus monophylla were the most widespread of these scrub conifers. Above the scrub conifer woodland in the highest mountains were forests of tall conifers.
The region also had native grasses. Meadows along streams carrying snowmelt from the mountains had a tall perennial wild rye, Elymus cinereus . Among the scattered sagebrush and the scrub conifers grew sparse perennial grasses, such as Agropyron spicatum, Poa secunda, Sitanion spp., and Stipa spp. The grasses aestivated during summer and the dried grass sometimes burned. Most grasses were not killed by fire, but sagebrush, junipers, and pinyons were. Fires did not generally spread far before being stopped by lack of fuel, especially on steep slopes and rocky ridges. Thus, burns created a patchwork of different-aged stands of sagebrush and woodland.
The vegetation was probably originally in near equilibrium with the native herbivores. Bison were few, in contrast to the great herds east of the Rockies. Other ruminants—mule deer, pronghorn antelope, and bighorn sheep—were present in limited numbers. Their facultative browsing and
grazing put little pressure on the vegetation. Rabbits and rodents were generally more important plant consumers.
The Indian inhabitants of the region were hunters and gatherers. They harvested plentiful crops of wild rye and pinyon nuts. There is no evidence that they had a significant effect on the vegetation.
Mormon settlements, starting at Great Salt Lake in 1847, rapidly fanned out through the region seeking irrigable land for intensive subsistence farming. Mormon livestock culture initially followed the North European pattern: community milk and meat cattle, draft animals, and sheep were kept in the settlement at night and herded nearby during the day. The result was severe but very localized overgrazing.
Extensive commercial livestock production developed in the Great Basin from different roots, that is, the Spanish American pattern of open range without fences or stored feed. It was stimulated by the mining booms and demand for meat in California after 1849, in Nevada's Comstock Lode after 1859, and by subsequent mining strikes. The areas of old Spanish settlement in California and New Mexico provided a source of cheap, abundant cattle and sheep to found the Great Basin herds. The range was free and belonged to those who got there first. Except during annual roundups, cattle and reserve horses were essentially free-ranging feral animals. Sheep were herded in large flocks, initially often without a home ranch. Itinerant sheepherders claimed as much right to free grass as anyone and drove their flocks wherever the grazing was best.
Initially, there was enough grass so that livestock could survive normal winters on the open range without unacceptable losses. However, overstocking and recurring droughts during the 1870s and 1880s greatly depleted the grass. In the severe winter of 1889–1890, over 250,000 head of livestock died on the ranges of northern Nevada alone. A new pattern then developed based on home ranches on streams with meadows supplying hay for winter feed for cattle and some sheep. Ranchers who had homesteaded the valley meadows inherited use of the surrounding open rangelands, theoretically federal property. The natural meadows were expanded by irrigation. The Indians commonly became hired hands on the ranches. Instead of harvesting wild rye and pinyon nuts, they fed hay from their ancestral meadows to cattle and cut pinyon and juniper trees for ranch firewood and fence posts. Some itinerant sheep operations still owned no home ranches and wintered the flocks in the lower elevation shadscale deserts.
In spite of occasional setbacks, livestock numbers continued to build up until early in the present century. Between 1900 and 1920, Nevada had an average of over 1,200,000 sheep, 400,000 cattle, and 70,000 horses. Feral horses kept grazing pressure on open ranges in winter, when ranches were feeding cattle at home. Since then, cattle numbers have fluctuated around the same level, while sheep and horse numbers have dropped precipitously to about 200,000 and 20,000, respectively. Droughts and depression in the
early 1930s wiped out most of the large sheep operations. Finally, in 1934 Congress passed the Taylor Grazing Act, under which 70 million ha of federal land in the western United States, including 71% of Nevada, were withdrawn from unrestricted open range and organized into controlled grazing districts. By then much of it had been reduced to nearly pure stands of unpalatable brush. Much of it was so degraded that even where grazing had been completely excluded for over 30 years, little recovery of forage production has taken place. It took less than 90 years for ranching and sheepherding to irreversibly alter the region's plant geography by unintentionally caused local and long-range plant migrations.
The most catastrophic retreats have been by native grasses, while the greatest advances have been by exotic grasses and weedy dicots. The wild rye, Elymus cinereus , in meadows along streams did not survive well under either grazing or mowing for hay and has been largely replaced by irrigated alfalfa, Medicago sativa , and Kentucky bluegrass, Poa pratensis , both natives of Eurasia. On the open range, whatever grasses are now present are predominantly Eurasian annuals with a long history of survival under intense grazing. The most abundant of these is cheatgrass or downy brome, Bromus tectorum ; it was first observed in the region about 1900 in western Nevada. The seed evidently was brought in the coats of sheep driven from California, where cheat had arrived a few years earlier. Unpalatable when mature, cheat has spread to fill the vacuum left on millions of hectares by removal of the native perennial grasses. This substitution appears to be permanent. Even in stands where remnants of the native grasses survive and livestock have been excluded for over 50 years, the natives do not displace cheatgrass. Another unpalatable Eurasian annual grass, Medusahead, Elymus caput-medusae , was also apparently brought from the Pacific coast in the coats of sheep. Arriving in the Great Basin in the 1930s, it spread rapidly in heavier soils than cheatgrass. It is still spreading in overgrazed areas, but in some areas where grazing has ceased, it has been replaced by a native perennial grass, Sitanion .
Various Eurasian annual dicots have also invaded the region. As noted above in Chapter 4, Salsola spread throughout the western states as a railroad ruderal. Accompanied by another Eurasian tumbleweed, Sisymbrium altissimum, Salsola fanned out into rangelands. Salsola obviously benefits from soil disturbance and removal of competition by grazing. A related chenopod, Halogeton glomeratus , was an obscure wild species in deserts east of the Caspian Sea until it showed up as a ruderal near Wells, Nevada, in 1934. It had spread widely along roads and in rangelands before it was recognized as toxic to sheep; it has killed thousands of sheep in the Great Basin. Halogeton now grows on millions of hectares of overgrazed Atriplex confertifolia and Artemisia tridentata rangeland. Halogeton leaf litter enriches the soil surface with salt, which favors its own seedlings over competitors.
Some of the palatable native shrubs have stories parallel to the native grasses. Formerly abundant species of Atriplex and Ceratoides that once supported
ported many sheep are now gone from large areas. Other native shrubs have had more complex changes in distributions, as have pinyon and juniper. They have been buffeted by changes in the fire regime more than by direct effects of browsing. When domestic livestock were first brought into the region, fire frequency may have been increased deliberately, especially by sheepherders, to stimulate grass; the woody vegetation may then have lost ground. Also, the Comstock Lode and later mining operations within the region consumed vast quantities of pinyon and juniper wood for mine timbers, fuel to power machinery, and charcoal for smelting. By the time mining collapsed late in the nineteenth century, trees had been stripped from country around mining centers up to an 80-km radius. How much effect this exploitation had on pinyon and juniper geography is uncertain; seedlings and saplings must have survived.
Meanwhile, as grass was lost from overstocked rangelands, fire could no longer spread. Unrestricted by fire and unbrowsed, big sagebrush spread where more palatable shrubs had been removed. So did junipers, pinyons, and other conifers where they were not being cut.
With the arrival of cheatgrass, the situation in the area it invaded was again reversed. The ungrazed mature grass was commonly dense enough to carry fires that killed patches of sagebrush, pinyon, and juniper. Also, after World War II, extensive areas of sagebrush and pinyon—juniper woodland were killed with herbicides or crushed and uprooted with heavy equipment to destroy the woody vegetation in an effort to increase grass production. Between 1960 and 1972, about 150,000 ha of woodland in Nevada and Utah were destroyed with heavy chains dragged between tractors by the U.S. Forest Service and the Bureau of Land Management. There was no hope of bringing back the native grasses, so the chained areas were generally seeded with Eurasian perennial grasses. Management of federal lands in the Great Basin has become very sensitive politically, with cattlemen and environmentalists in polar positions on continuation of grazing. The cattlemen have a great asset in the popular view of cattle grazing as part of the romantic western tradition. Few people except range scientists are aware of the impact of grazing on the Old West.
Feral Livestock, California Channel Islands
(Brumbaugh 1980; Coblentz 1978; Dunkle 1950; Goeden and Ricker 1981; Goeden et al. 1967; Hobbs 1980; Johnson 1980; Minnich 1980, 1982; Philbrick 1972, 1980; Raven 1963; Thorne 1969; Westman 1983)
After the prehistoric extinction of pygmy mammoths, the vegetation of the islands off the southern California coast was untouched by large herbivores before the introduction of livestock in the nineteenth century. The only
terrestrial mammals were rodents, skunks, and diminutive foxes, all of which may have ridden in the seagoing canoes of the Chumash Indians who frequented the islands. The islands were never connected with the mainland, but during glacial low sea stands, the channel separating some of them from the mainland was only a few kilometers wide and presumably easily swum by the elephants.
The rich native flora included many endemic species, some of which are believed to have originated on the islands by adaptive radiation. Others are survivors of once widespread species known only as fossils on the mainland, such as island ironwood, Lyonothamnus floribundus , and island oak, Quercus tomentella . Many of the native island species are conspecific with those on the nearby mainland, although it has been suggested that shared chaparral shrubs evolved more arborescent forms on the islands. This so-called island gigantism may be an artifact of grazing and browsing by feral sheep and goats. Their voracious consumption of plant material reduced fuel and prevented chaparral burning, which was probably always less frequent than on the mainland because of the maritime climate. The chaparral shrubs thus have prolonged lives while being trimmed up to tree form by browsing.
Each of the eight major islands has had a different history of livestock introduction. Four will be sketched here.
San Miguel is the westernmost and most windswept of the islands. It is rather low and most of its 37-km2 surface is covered with Pleistocene dunes. In Holocene time, these had been stabilized by a cover of coastal sage scrub and grass. In the mid-nineteenth century, the island was stocked with over 6,000 sheep and some cattle and horses. During 3 years of drought in the 1860s, nearly all of these starved to death. Overstocking continued, and during subsequent droughts over half of the island became a waste of drifting sand. Regrowth during wet years was mainly by grass and other herbs. The number of sheep was finally reduced to about 3,000 in the 1930s and most were removed in 1950. After the National Park Service took over the island, the remaining sheep were shot in 1968 and feral burros were removed in the 1970s. Successive aerial photographs show gradual revegetation of some of the dunes since 1960. However, the original coastal sage scrub and grass vegetation has not recovered. Some recolonization is by a native morning glory vine, Calystegia macrostegia , found on coastal bluffs on the islands and mainland. Much of the colonization is by the perennial Australian ice plant, Mesembryanthemum aequilaterum , discussed in Chapter 1.
Santa Cruz is the largest and most rugged of the islands, with an area of 250 km2 and peaks up to 750 m high. Cattle, horses, sheep, and pigs were introduced in the mid-nineteenth century. Cattle and horses have remained under control, mainly in valley grasslands and on gentle slopes. Vegetational changes there are much like those that began earlier in mainland grasslands. The native perennial bunchgrasses have declined under grazing and have been mostly replaced by annuals. Some of the annuals are unpalatable natives,
such as doveweed or turkey mullein, Eremocarpus setigerus , probably originally confined to intermittent watercourses, and a tarweed, Hemizonia fasciculata , which may have been a member of the original grassland. The cattle and horse pastures are now dominated by European annuals, among the most common being a wild oat, Avena barbata ; brome grasses, such as Bromus mollis, B. rubens , and B. diandrus ; filaree, Erodium cicutarium ; burr clover, Medicago polymorpha ; cat's ear, Hypochaeris glabra ; and milk thistle, Silybum marianum . The pastures have also been heavily invaded by a European perennial, Foeniculum vulgare (fennel), which was introduced to California as a culinary herb and has spread widely as a ruderal. On Santa Cruz Island, it dominates pastures near the boat harbor and ranch house. Although eaten by cattle when young, it is evidently unpalatable when flowering. It is currently spreading rapidly, not only in pastures but under scrub oak woodland that appears undisturbed. As on the mainland, cattle have had relatively little impact on the native scrub and woodland vegetation.
Sheep have had far more impact on Santa Cruz Island scrub, both coastal sage and chaparral, and on woodland, both hardwood and pine. By 1870, the island had about 45,000 sheep and by 1875, about 60,000. They were allowed to run free and only about half could be rounded up for shearing annually. Tens of thousands were slaughtered during drought years to prevent total desertification and starvation. Feral pigs also became abundant during the late nineteenth century and prevented reproduction of both scrub oaks and woodland oak trees by rooting for acorns. Late nineteenth century accounts and photographs record progressive destruction of coastal sage and other vegetation and increasing erosion. In the bare areas, a cactus native to coastal bluffs, Opuntia littoralis s.l., spread inland. Most of the denudation took place during the late nineteenth century. Since aerial photographic coverage began in 1929, there has been little further attribution of woody vegetation except near the eastern tip of the island, which continues to be operated as a sheep ranch.
Starting in 1939, the Stanton Ranch, which had acquired all but the eastern tip of the island, began trying to eliminate the sheep. Tens of thousands were shipped to market and tens of thousands of others that could not be caught were shot, along with innumerable pigs. During the 1950s, the Stanton Ranch built a huge network of fences to control the cattle and exclude sheep and pigs from large portions of the island. The fences are often broken through by pigs and by floods in stream channels, so the feral sheep and pigs are seldom completely absent for long. However, the vegetation contrast across some fence lines is dramatic. In wet years, the contrast is less striking because of temporary cover by European annuals in the sheep country. Generally the sheep side of the fence has much more bare ground, browsed shrubs, and no young trees.
Since the 1929 photographs were taken, a stand of bishop pine, Pinus muricata , in sheep country on the north side of the island has decreased
greatly in density. The trees are all 40 to 70 years old with no seedlings or saplings; the average life span of a bishop pine is about 65 years. Two other bishop pine stands on the island had no seedlings and also faced extinction before sheep were fenced out in the 1950s. Pine reproduction is now abundant in various age classes. Reproduction of other native trees and shrubs is virtually nil in sheep country, with a few exceptions, including an unpalatable bush groundsel, Senecio douglasii .
The Nature Conservancy is in the process of taking over the Stanton Ranch and faces the formidable task of eliminating feral livestock. It is probably already too late to save some species, for example, an endemic monkey flower, Mimulus brandegei , first seen in 1888 and last seen in 1932.
The invasion of the interior of Santa Cruz Island by the coastal Opuntia littoralis has been reversed by a biological control program, the oldest one in North America and the only one anywhere to successfully control a native weed with purposely introduced insects. In 1939, an estimated 40% of the rangeland was considered useless because of dense Opuntia infestations. In 1940, entomologists from the University of California, Riverside, and the Stanton Ranch began working to establish insects that fed on Opuntia littoralis on the mainland coast but that were not present on the island. The most successful was a cochineal bug, Dactylopius opuntiae , which since 1951 has destroyed most of the Opuntia populations of the island. At present the situation appears to be equilibrating, partly because the cacti are less dense and more resistant kinds have survived, but mainly because the cochineal bug was followed to the island by some of its own predators, particularly a moth, Laetilia coccidivora , which arrived in the early 1970s. Areas of dead cactus have commonly been recolonized by native coyote brush, Baccharis pilularis .
Santa Barbara Island has a surface area of only 2.5 km2 , mostly on a low mesa that emerged from the sea in Pleistocene time. In spite of its recent origin, the island has a native flora of about 70 species, all but one shared with other islands or the mainland; a species of live-forever, Dudleya traskiae , is endemic to the island. Vegetation on the mesa top was largely destroyed by cultivation of crops, a topic outside the scope of this chapter. However, the peripheral bluffs and sea cliffs are relevant here because they were invaded by feral livestock in the form of domesticated European rabbits. These were introduced by military personnel stationed on the island during World War II, after the island had been declared a national monument and farming was excluded. The rabbit population peaked at about 2,600 in 1955, destroying most of the native coastal bluff vegetation, which had been dominated by the arborescent sea dahlia, Coreopsis gigantea , and a morning glory vine, Calystegia macrostegia . An annual South African ice plant, Mesembryanthemum crystallinum , took over much of the denuded area. The National Park Service began shooting rabbits in 1954, and by 1958 so few rabbits were left that the native species began to regenerate from sprouts and seedlings. Even the endemic Dudleya traskiae , which had been considered extinct, reappeared in 1974 in former rabbit territory.
Santa Catalina is almost as large and rugged as Santa Cruz and has similar natural vegetation, except that Catalina has no pine forests. Feral goats were probably present by 1840. The island had commercial sheep and cattle ranching from about 1850 to 1950, when domestic livestock other than some bison were removed. Late nineteenth-century photographs of Catalina show that overgrazing was already widespread and severe: coastal sage scrub was nearly gone, chaparral had been opened up to a scrub savannah, and the coastal Opuntia littoralis had invaded bare inland areas. Pigs were introduced in the 1930s. Today feral goats and pigs are abundant on much of the island. Goat browsing has selectively eliminated most chaparral and coastal sage species, leaving unpalatable Salvia apiana, S. mellifera, Rhus integrifolia , and R. laurina . In the worst goat-occupied wastelands, the only perennials are Opuntia littoralis s.l. and the South American Nicotiana glauca . The Catalina Island Company chose not to introduce cactus-feeding insects, preferring cactus cover to bare ground.
Development of the resort town of Avalon in the 1890s was followed by dramatic reinvasion of surrounding parts of Catalina by native shrubs. Slopes within sight of the town are shunned by the goats. What was in the 1880s an overgrazed landscape of sparse grass, cactus, and scattered browsed shrubs had by 1900 become covered by coastal sage scrub. Since 1940 the area has been invaded by chaparral species, notably the bird-dispersed Rhus integrifolia and Heteromeles arbutifolia . The same two species are invading stands of Quercus dumosa in goat-free areas where pig rooting has completely stifled oak reproduction. The Island Company has carried out goat removal and fencing in parts of the islands. This work may be expanded because most of Catalina has been placed in a conservation easement with the Los Angeles County Department of Parks and Recreation. In areas where the goats are gone, native shrubs are recolonizing rapidly. One of the most successful of these is the endemic St. Catherine's lace, Eriogonum giganteum . Of about 200 species in the original native flora, about 40 have not been seen in the last 25 to 30 years. Some of these survive on neighboring goat-free islets. Others are shared with other major Channel Islands or the mainland and may return to Catalina some day. Others are believed extinct, such as Mimulus traskiae , known only from Catalina and not seen for 50 years.
Rabbits, Lisianski Island, Hawaii
(Clapp and Wirtz 1975)
Lisianski is a low, coral sand island, about 200 ha in area, located about 1600 km northwest of Oahu. In the early nineteenth century, it was described as having a few patches of coarse grass and shrubs (the species not named), abundant elephant seals and green turtles, and teeming seabird rookeries. Between 1904 and 1909, the island was occupied several times by Japanese
landing parties, who killed several hundred thousand seabirds for sale of feathers to the French millinery trade. In 1909, Theodore Roosevelt declared the island a bird sanctuary. When the Japanese left, they did not take their domestic rabbits. By 1914, the rabbits had eaten all the vegetation on the island except for some tobacco plants near the abandoned houses and two vines of an Ipomoea sp., perhaps I. pes-caprae , the pantropical beach morning glory; most of the rabbits had starved to death. By 1916, all the rabbits were dead and there were no traces of plant life except some algae.
A scientific expedition in 1923 found four plant species on Lisianski: Eragrostis variabilis and Nama sandwicensis , which are Hawaiian Island endemics that are probably bird dispersed, and Portulaca lutea and Sesuvium portulacatrum , which are pantropical, sea-dispersed beach plants. They also found an unidentified Ipomoea seed and planted Barringtonia asiatica , an Indo-Pacific beach tree, which did not survive. The vegetation in 1923 was restricted to tiny areas. By 1943, much of the island had sparse vegetation, and thickets of Scaevola taccada had developed along some beaches. This Scaevola is a wide-ranging Indo-Pacific seashore shrub, dispersed by both ocean currents and birds. By 1969, the vegetation was thick and included 13 species. Two of these had been deliberately planted: Casuarina equisetifolia and Chenopodium oahuense .
Pigs, Clipperton Island
(Sachet 1962)
Clipperton lies in the tropical eastern Pacific about 1,000 km off the coast of Mexico. This island consists mostly of a ring of coral sand and rubble surrounding a brackish lagoon. In the lagoon is a small outcrop of the volcanic rock on which the atoll is founded. When the island was first described in 1711, it had some low bushes on it, but by 1858 the vegetation had disappeared. The island then teemed with nesting seabirds and swarmed with land crabs.
Clipperton was occupied by guano diggers between about 1897 and 1917. Pigs were introduced by 1897 and about a dozen were left behind when the island was abandoned in 1917. The pigs survived on the abundant crabs and bird eggs.
A scientific expedition to Clipperton in 1958 found the island biota very different from that reported a century before. There were about 30 species of seed plants, mostly weedy grasses and dicot herbs, probably introduced by the guano diggers or later visitors. There were also masses of Ipomoea pes-caprae vines, probably derived from drift seeds. Various species of sedges had colonized the marshy lagoon edge, and various submerged aquatics, including Najas marina, Potamogeton pectinatus , and Ruppia maritima , grew
in the lagoon. Birds were much less abundant than in 1858, but various species of seabirds and shore birds were present. American coots were swimming in the lagoon with chicks; Sachet (1962) suggested that coots had brought seed of the sedges and aquatics. She also suggested that the feral pigs had been responsible for reducing the bird and crab populations and, in so doing, had permitted plant species to become established that had previously been barred from the island.
On Canton Atoll in the South Pacific, Degener and Degener (1974) noted that drift seeds of many species not present on the atoll commonly wash up on the beaches and germinate, only to be eaten by crabs; Ipomoea pes-caprae was specifically cited as an example. Clipperton has undoubtedly always been within the seed shadow of this beach morning glory, but could not escape the crabs until pigs entered the system. On Clipperton, pigs, functioning as carnivorous predators, played a role in vegetation change opposite to their role on many islands.
The 1958 expedition to Clipperton found 58 pigs hiding in the masses of beach morning glory; all 58 were killed on charges of molesting the nesting birds. The effects of their removal await study.
Cattle Rangeland, New Caledonia
(Barrau 1980)
In New Caledonia, traditional Melanesian shifting cultivation was displaced in the mid-nineteenth century by French colonial cattle ranches. In this seasonally dry tropical island, prehistoric slash and burn agriculture had probably been responsible for some expansion of savanna woodland and grassland at the expense of forest, but the Melanesians had no grazing animals and no incentive to convert forest to grassland. Savannas were more difficult to prepare for cultivation than forest fallow. Their irrigated terraces and yam mounds were permanent structures, but were periodically allowed to grow up to forest for later clearance by burning. The precolonial landscape had villages and gardens interspersed with stands of forest and patches of savanna woodland and grassland. The French could not see such a landscape without thinking of cattle ranching. By 1859, 1,000 head were imported from Australia, and by 1883, New Caledonia had 88,000 cattle. The Melanesians were driven out of large areas and their gardens converted to open range. Very quickly the vegetation became simplified, with overgrazing and spectacular erosion in some areas. There were die-offs of cattle during drought, and burning was extensively practiced to induce palatable regrowth. Though perennial grasses, particularly Imperata cylindria and Heteropogon contortus became dominant. A fire-resistant tree, Melaleuca leucadendron , originally confined to marsh margins, invaded more and more savanna areas. It was joined by
weedy shrubs from the American tropics, especially Lantana camara and Psidium guajava .
Desertification, Sahel
(Ibrahim 1980)
Between the Sahara and mesic Subsaharan Africa, the Sahel is a huge, semiarid savanna, a seasonally green grassland with scattered thorny acacias and other shrubs and trees. Since antiquity, most of it has been the domain of nomadic pastoral peoples moving northward during the rains, leaving the mud and disease of the south for the temporary grass and watering places of the Sahel. The nomads shared parts of the region with seminomadic or sedentary agriculturists, who planted millet, sesame, and other crops that required only brief rains. The economy and the natural vegetation were perhaps roughly in equilibrium, although under stress in drought years.
In the present century, with the advance of modern human and veterinary medicine and the drilling of water wells, this relative stability was changed. In North Darfur between 1917 and 1977, for example, human populations increased sixfold; sheep, goats, and camels more than ten-fold; and cattle twenty-fold. Starting in 1965, there was a 12-year period of below average rainfall. Similar droughts had often happened before, but not with the same grazing pressure. Unprecedented deterioration of the vegetation was widely evident by 1977, less in areas where traditional nomadic grazing had continued than where newly sedentary population had become concentrated around modern pumped wells. In a wide ring around these, all palatable grasses, including Cenchrus biflorus, Eragrostis tremula , and Aristida pallida , disappeared. Browsing coupled with fuel wood cutting eventually destroyed the acacias. In some places, removal of vegetation reactivated fossil dunes that had formed under an ancient, more arid climate. The newly bared areas were invaded by some native pioneer shrubs, such as Capparis decidua and Ziziphus spina-cristi , that had formerly been restricted to dunes and wadis. Sheep burr, Acanthospermum hispidum , an unpalatable annual composite native to tropical and subtropical America, has invaded overgrazed areas. The burr is carried in the coats of livestock. It is eaten by starving camels, donkeys, and goats where nothing else grows.
Overstocked Game Reserves, East and South Africa
(Barnes 1983; Cumming 1981; Owen-Smith 1981)
A serious problem has developed in the famous African wild animal reserves as large herbivore populations have increased above the carrying
capacity of the vegetation. Formerly free-ranging herds have been compressed into small remnants of their former territories.
In the Imfolozi Game Reserve in Natal, rhino-proof fences have confined a population of white rhinos, which has increased since 1930 from a few hundreds to thousands, reaching a density of over five per square kilometer. The reserve is shared with other ungulates, and their combined grazing has far exceeded the carrying capacity of the grassland. The formerly dominant tall grass, Themeda triandra , is gone from much of the area; it was partly replaced by creeping grasses, but much ground is bare and eroding. Because the sparse grass no longer carries fire, unpalatable woody plants have invaded it.
The problem is different for elephants because woody vegetation suffers most. Elephants not only break foliage and branches off trees for feed, but also damage or destroy trees for amusement. A single elephant may push over 1500 trees a year. After being decimated by ivory hunters for centuries, with the kill peaking in the mid-nineteenth century, elephant populations protected in reserves have rebounded dramatically and are now destroying their resources. In a study area in Zimbabwe, a woodland dominated by miombo, Brachystegia boehmii , lost 50% of its biomass in 4 years; in another study area, a miombo woodland was destroyed in 6 years. In a study area in Tanzania, during 5 years, a Commiphora ugogensis woodland lost 67% of its trees and a woodland of Acacia albida lost 40%; there was no tree regeneration in either area. In Kenya, elephants have been eliminating baobab, Adansonia digitata , from Tsavo National Park. Under primeval conditions, before people and their livestock had taken over most of the land, elephants would have moved on to greener browse after destroying a stand of trees. The open area could have become a grassland, maintained by fire and supporting herds of antelope and other grazing animals. In the absence of fire, trees would have recolonized until the elephants returned, in an endless cycle. The elephants have no place to go now; game managers have the horrible choice of letting them destroy their food supply and starve or shooting them wholesale.
Rise and Fall of Opuntia, Australia
(Hosking and Deighton 1979; Mann 1970; Osmond and Monro 1981)
About 30 species of cacti native to various regions of North, Central, and South America have become naturalized at least locally in Australia. Nine of these have become pests over considerable areas of southeastern Queensland and northern New South Wales. Australian botanists generally identify the worst pest cacti as belonging to two species, Opuntia stricta and O. inermis . American botanists generally consider O. inermis to be a synonym of O. stricta . What is called O. inermis in Australia may be called O. dillenii in the Americas. Whatever they are called, these prickly pears are native to
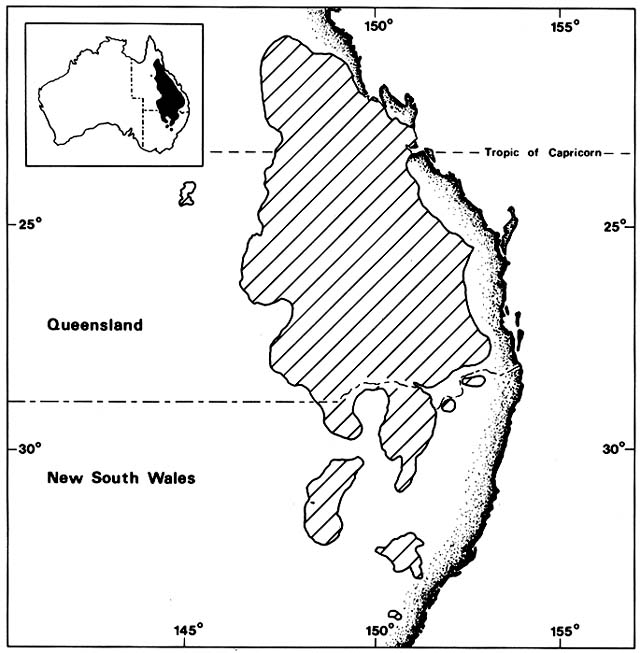
Figure 6. Maximum Range of Two Introduced Cacti (Opuntia stricta and O. inermis ) in Australia. Many plants
have become aggressive invaders when dispersed to regions free from their former parasites and herbivores.
Australia had no cacti until the nineteenth century when various New World species were introduced in cultivation.
Several of these escaped and spread rapidly in spite of control efforts. The map shows the combined ranges of the
two worst pest species in the early 1920s, before they were eliminated by insects introduced from South America.
(Adapted from Mann, 1970)
seashores of the Gulf of Mexico and Caribbean. How and when they reached Australia is unknown, but by the mid-nineteenth century they were being widely planted for hedges by homesteaders in eastern Australia. Both spread on their own on the cattle and sheep ranges and were recognized as a nuisance by the 1880s. Dispersal was partly by vegetative propagation from pads adhering to livestock and partly by seed. The fruits were attractive to feral pigs, emus, and many other mammals and birds.
How successful the cacti would have been if the region had been ungrazed is uncertain. They spread mainly in open savanna woodlands, largely dominated by brigalow, Acacia harpophylla , and Casuarina spp., vegetation types in which grass fires were a normal part of the system. Hotter fires in ungrazed grass would presumably have been harder on the cacti than on the native plants. Although the two Opuntia species did naturalize in some coastal sites similar to their home habitats, their main infestations were inland, on both sides of the Great Dividing Range, up to elevations of about 800 m. The western limit was about 650 km from the coast. At their peak in the early 1920s, the two Opuntias infested about 25 million ha of land between 21° and 33°S latitude (fig. 6). Mechanical removal and chemical control cost more than the value of the land. Many sheep and cattle holdings were abandoned as useless.
In 1924, the Queensland government paid bounties for the slaughter of 335,000 emus and other birds blamed for spread of cactus seeds. The following year it was estimated that the cacti spread at an average rate of 100 ha/hr.
The tide was turned by biological control, an approach pioneered by Australian entomologists. Work had begun in 1912, when the Queensland government sent an expedition abroad to find cactus-feeding insects. They brought back a moth species native to Argentina, Cactoblastis cactorum , which unfortunately did not survive. They also imported cochineal bugs, Dactylopius spp., which were successfully reared, but work was suspended for the duration of World War I. In 1919, the Commonwealth Prickly Pear Board was set up. New expeditions to the Americas collected many kinds of cactus-feeding insects, including about 50 new to science. These were reared in quarantine and screened by starvation tests on economic plants. The first insects to be released were cochineal bugs, Dactylopius opuntiae , native to the southwestern United States. The bugs were successfully established on pest cacti in several Australian localities in 1921–1923; by 1924 patches of the cacti began to die. The government, enthusiastically helped by private initiative, spread the bugs along all the roads in the cactus-infested territory. The effect, however, was somewhat disappointing as cactus mortality was highly variable.
The catastrophic wiping out of the cacti was not accomplished by Dactylopius but by Cactoblastis cactorum . This moth was reimported from Argentina and finally released throughout the cactus-infested territory in 1926–1927. Moth eggs were supplied by the hundreds of millions free of charge
to landholders. The larvae fed on the cactus pads, riddling them with tunnels; most of the cacti promptly collapsed and died. By 1933, 90% of the Queensland cacti were gone. The crash of the cacti was followed by a crash of the insect population; then there was some cactus regeneration and resurgence of the insects. The cycles continued in constantly damping waves. At present, only a few cacti and their obligate parasites survive in scattered colonies, carrying on a stable hide and seek, host—predator relationship.
The story is not over. The tiger pear, Opuntia aurantiaca , native to Argentina and Uruguay, became a relatively minor pest in eastern Australia after 1911. It is currently spreading in east-central New South Wales in spite of large sums spent on attempted control. Cactoblastis larvae feed on the tiger pear, just as they do in their joint homeland, but they are not lethal to the cactus and do not stop its spread.
Comment
Pastoral peoples have commonly introduced domesticated pasture plants, both grasses and legumes, along with their livestock. This kind of comigration of symbiotically associated plants and animals occurred during European colonization of eastern North America and New Zealand, for instance. Since crop migrations are outside the scope of this book, the cases considered here follow other patterns.
Many cases have involved turning domestic animals out to shift for themselves on wild vegetation composed of species they had never met before. When this was done on islands that had previously lacked large herbivores, the effect on vegetation was catastrophic. There are many cases other than those discussed above; three additional examples on uninhabited islands can be cited. Ilha da Trindade in the tropical South Atlantic had forests that were destroyed by goats and pigs liberated by the famous scientist, Edmond Halley, in 1700 (Eyde and Olson 1983). Macauley Island in the Kermadec group in the temperate South Pacific had several dominant forest tree species that were exterminated by goats and pigs liberated in the early nineteenth century (Sykes 1969). Lastly, Rabbit Island, located 3 km off the Australian mainland in Bass Strait, acquired its rabbits in the mid-nineteenth century. The exterminated most of the flora. After the rabbits were in turn exterminated in 1960, plant recolonization from the mainland was rapid. The number of native species in the flora increased from 20 in 1960 to 49 in 1980 (Norman and Harris 1981). The losses of plants in these three cases may be partly attributable to island plants being poorly adapted to cope with herbivory, but they can probably be mainly blamed on uncontrolled growth of animal populations. Feral livestock on islands have commonly been free
from diseases as well as predation. The ultimate control on their populations is starvation.
Recent introduction of domestic herbivores to new continents, specifically Australia and the Americas, has also had catastrophic effects. Ecosystems with rough equilibrium between native plants, herbivores, and predators were replaced with new systems in which the predators were eliminated and grazing pressure increased by orders of magnitude. Although the native floras had coevolved with herbivores, the plants were unprepared for the escalated consumption, and the more palatable species generally retreated from large areas. In southwestern North America, various wild plants that were formerly gathered in quantity for food by the Indians are now extinct over large regions; some survive only where protected from grazing (Bohrer 1978).
The other side of the coin is the advance of plant species that benefit, in one way or another, from increased herbivory. Some of these are native pioneers from nearby riparian, coastal, and other open sites that invade areas formerly closed to them. With overgrazing, opening up of bare ground and reduction of burning are so tightly correlated that it is often hard to sort out their roles in allowing these advances. This question arises in many cases in addition to those cited above, for example, in advances of mesquite (Prosopis spp.) in overgrazed grasslands in southwestern North America (Fisher 1977; Gilbert and Moore 1985) and in Argentina (D'Antoni and Solbrig 1977).
Along with the native pioneers, exotic invaders usually thrive with increased grazing. Occasionally, some species arrive in the coats of the introduced livestock, but usually they arrive later by accidental or deliberate human dispersal. It is commonly assumed that this is because they had to await habitat disturbance, but this is not always proven. In western North America, for example, Bromus spp. and other Eurasian annuals have spread on both overgrazed and undisturbed ranges. These annuals grow so fast and abundantly in the spring that they are only partly consumed by livestock. Unpalatable when mature and dry, they accumulate as dead fuel. Commonly this has resulted in reversal of the advance of fire-sensitive native species after grazing removed the original fire-carrying native grasses. Furthermore, in the Mojave Desert of California, these Eurasian annuals have invaded ungrazed regions dominated by creosote bush, Larrea divaricata , and cacti, Opuntia spp., which were originally fireproof because of sparse herb cover. Repeated burns decimate and may eventually eliminate native shrubs.
Drastic effects of increased herbivory are not confined to islands and continents where herbivores are invaders. The African examples discussed above show that even where plant and animal species have lived together since prehistoric time, recent human-controlled buildup of animal populations has also resulted in local retreats and advances of native species and invasion of exotic, unpalatable species.
Altered Forest, Woodland, And Brushland
Fire Regimes
Conifer and Hardwood Forests, Great Lakes Region
(Curtis 1959; Flader 1983; Heinselman 1981; Loucks 1983)
Finley (1976) and other workers have made magnificent maps of vegetation of the Great Lakes region from the original land survey records preceding white settlement. These showed an intricate forest mosaic with kaleidoscopic associations of dominant tree species. The patterns included a great climatically controlled gradient between boreal conifer forest and temperate deciduous hardwood forest. Other patterns resulted from edaphic variables, largely due to recent glaciation. Superimposed on these were dynamic patterns resulting from natural and artificial disturbance. Windthrow, lightning-set fires, and Indian burning were pervasive factors in the region. Only small parts of it were in the maple—beech—hemlock forest type discussed in Chapter 2, which had gone long enough without disturbance for fire-dependent species to have been eliminated by succession.
Fire can probably be treated more realistically as a partial function of vegetation than as an independent variable. Allowing for some chance variation in ignition and spread due to weather, fire regimes and vegetation may covary as roughly predictable feedback systems.
The original boreal conifer forests were subject to large, intense crown fires in a cycle of roughly 50 to 100 years. Of the dominant conifers, black spruce, Picea mariana , and jack pine, Pinus banksiana , reoccupied burns immediately from seed that survived on killed trees in unburned cones. White spruce, Picea glauca , and balsam fir, Abies balsamea , did not survive on burns but recolonized more slowly from wind-borne seed from outside the fire perimeter. The conifers were often joined on new burns by Populus tremuloides and Betula papyrifera . Both resprouted vigorously after burning and could persist indefinitely on sites where fires recurred often enough to prevent takeover by the longer lived conifers. Dry sandy regions had grassy jack pine barrens kept open by frequent ground fires. The southern margin of the boreal forest had scattered groves of white and red pine, Pinus strobus and P. resinosa . These pioneer pines were incapable of invading undisturbed forest, and the groves may have been initiated by windthrow followed by hot fires. These pines grew taller and lived longer than other trees in the region; mature groves survived recurrent ground fires.
South of the boreal forest was a wide belt of mixed conifer—hardwood forest with very complex, patchy species patterns. All the trees mentioned above ranged down into this zone, with fire regimes similar to those in the north. The importance of these northern species decreased gradually southward,
and they became increasingly restricted to marginal sites, for example, black spruce in swamps and pines on cliffs, while various oaks and other deciduous hardwoods became increasingly dominant. The hardwood forests, even those that had not reached the maple—beech—hemlock stage, commonly were too shady to have much undergrowth, and crown fires were unlikely. Perhaps some occurred during extreme droughts at intervals of centuries.
The transition from hardwood forest to prairie in the southwestern Great Lakes region was a patchy mosaic of forest, oak savanna, and open prairie. The prairies had a rich flora of perennial grasses and dicot herbs, all adapted to frequent, even annual, fires. The parklike oak savannas were generally dominated by burr oaks, Quercus macrocarpa , mostly huge, old fireproof trees. The savannas also contained various species of oaks that were killed back to the ground by grass fires but resprouted repeatedly; these developed massive root systems, locally known as grubs, but had only low, bushy shoot systems.
Throughout the region, fires were commonly set by lightning and by Indians. Most of the Indian groups in the Great Lakes region were hunters, fishers, and gatherers. In the southern part of the region, sedentary agricultural groups opened clearings in the hardwood forests for milpas to produce crops of Mexican origin. The abandoned fields were colonized by white pines and other pioneer trees.
This whole fabric, from boreal forest to prairie, was torn apart and rewoven into a different pattern in less than 100 years. Boom and bust logging moved westward through the region in the late nineteenth century, concentrating on old growth white and red pines. Groves were clear-cut and hardwood forests were scavenged for scattered pines, even if there were only one or two per acre. By itself, this selective logging would have been a brief perturbation with no great effect on the forest in the long run because the pine species were not eliminated. However, the logging was merely the beginning of general deforestation. In part, this was due to accidental conflagrations, commonly started in logging slash. In the drought year of 1871, crown fires starting in slash burned 1.5 million ha of Michigan and Wisconsin forests. Much more forest was deliberately cut and burned by settlers, largely land-hungry immigrants from northern Europe who regarded hardwood forests as indicators of good soil. Many worked part-time as loggers or miners while gradually clearing a farmstead. The region's copper and iron mines also consumed hardwood as charcoal for smelting. Later, there was some commercial cutting of hardwoods for flooring and other lumber. However, by and large, the Great Lakes forests were destroyed through persistent hard work by would-be farmers trying to execute a national policy: the plow follows the axe.
By the 1920s and 1930s, it became obvious that much of this region, now known as the Cutover, was submarginal for agriculture. Farm abandonment and population exodus began. By then, seed sources for most of the
conifers and nonsprouting hardwoods had been so reduced that direct recovery of former species distributions was impossible. Huge expanses of country became blanketed with nearly pure stands of scrubby aspen and birch, persistent sprouters with wind-borne seed.
Pulpwood exploitation of the boreal forest conifers had begun in the region about 1900. As the stands of these trees were depleted, the paper companies switched to aspen and birch. Formerly considered useless weed trees, these are now a major resource of the region, with stands deliberately maintained by clear-cutting on a short rotation. Aspen and birch, formerly minor pioneers, now dominate far more of the Great Lakes forests than any other species.
Where the Cutover is not being exploited for pulpwood, fire suppression is permitting gradual spread of later successional trees. However, this does not mean that forests are returning to their former patterns. Modern fire suppression programs have been so effective that most of the Great Lakes forests can now be expected to die of old age before experiencing a fire. As compared with presettlement fire regimes of burns every 50 to 300 years, the current fire rotations are mostly in thousands of years. If maintained, only a few extremely shade-tolerant species will reproduce and survive.
South of the Cutover, in the regions formerly in a mosaic of hardwood forest, savanna, and prairie, permanent agriculture and pasture were successfully established in the nineteenth century. Even before they were plowed, the prairies and oak savannas were generally converted to pasture for livestock. Burning was promptly stopped. The consequences for the patterns of species distribution would have been profound, but the point is moot because invention of the moldboard plow in the mid-nineteenth century made it possible to completely destroy prairie vegetation and open up the rich black earth beneath for crops. Like the bison, prairie plant species survive only in a few protected reserves. There are a few exceptions; some prairie species of low palatibility, such as Solidago rigida and Artemisia caudata , survive on farms on pastured slopes too steep and dry to plow. They grow mixed with immigrant Eurasian species, such as Poa pratensis and Taraxacum officinale . In the oak savannas, with end of burning, oak grubs and seedlings rapidly grew up to young forests. Remnants of these and of the older hardwood forests of the region that have not been cleared are now generally used as farm woodlots; they also serve as shady refuges for cattle in the summer. Browsing eliminates tree seedlings, and trampling changes the herb layer composition, with Plantago major and other Eurasian weeds now common.
Conifer Forests and Woodlands, Western United States
(Arno 1980; Gibbens and Heady 1964; Habeck 1976; Harvey et al. 1980; Houston 1973; Johannessen et al. 1971; Kilgore 1981; Kilgore and Taylor
1979; Lockmann 1981; Lunan and Habeck 1973; McBride and Jacobs 1980; Minnich 1977, in press; Rundel 1972; Vankat 1977; Zedler 1977)
For millennia, uncontrolled wildfires were a normal part of the coniferous forest ecosystems of western North America. The spatial pattern of species and the pattern of burning were interrelated in a feedback system, each being partly dependent on the other. Different kinds of conifer stands tended to generate and be a consequence of different fire regimes. For example, at higher elevations in the Rocky Mountains, dense Picea engelmannii forests were typically fire free for about a century in spite of occasional lightning strikes before the inevitable crown fire during extreme drought. On north slopes, spruce seedlings would generally reoccupy the burn at once; on south slopes, there was often a simple succession, with Populus tremuloides and other pioneer trees dominating the site for about 50 years before the spruce regained dominance.
Douglas fir, Pseudotsuga menziesii , had varying fire regimes in different parts of its range. In the normally humid maritime climate of the Pacific Northwest, Douglas fir typically grew in huge, approximately even-aged stands initiated by catastrophic crown fires. A Douglas fir can live for over 1,000 years, but most stands in the Northwest burned within 500 years, probably after rare lightning strikes during abnormal summer droughts. Dominance of the species was maintained by these fires that had long return intervals and replaced entire stands. Otherwise the species would have eventually been displaced by more shade-tolerant conifers, for example, red cedar, Thuja plicata ; hemlock, Tsuga heterophylla ; and grand fir, Abies grandis . In the drier interior east of the Cascades and in the Rockies, Douglas fir commonly grew in an open grassy park landscape, where small surface fires set by lightning were very frequent. Occasionally a stand was fire free for 30 years or more, long enough for reproduction to build up a dense stand of saplings vulnerable to crown fire.
At slightly lower elevations than Douglas fir, Pinus ponderosa also grew in open grassy park landcapes over huge areas of the interior. Most of the old trees but few seedlings survived frequent fires; some old ponderosa pines survived repeated lightning strikes that ignited grass beneath them. The Sierra bigtree, Sequoiadendron gigantea , grew in scattered groves surrounded by a sea of other conifers. Mature and old trees invariably have scars of repeated fires on their trunks; the fires burned under the trees without crowning. Sequoiadendron cones open when heated by fire, releasing seeds that find optimum conditions for establishment on a fresh burn.
These generalizations about fire regimes are, of course, oversimplified. Fire frequencies and intensities were highly variable from time to time and place to place within a given type of forest. Vagaries of firekill and postfire recolonization produced a dynamic patchwork of stands differing in age
structure and species composition. The scale of the patches varied with topography, tending to be finer in more rugged terrain.
Incursions of sheepherders, ranchers, and loggers and disruption of the Indian populations undoubtedly had some effects on fire regimes, but it is unclear what those effects were. Later on, as settlement proceeded and concern about uncontrolled fires grew, it became customary to blame human ignitions by Indians, sheepherders, or whomever for catastrophic destruction for forests. Few people recognized that wildland fires might be natural and inevitable. In southern California, for example, it was widely believed that fires caused by humans had destroyed formerly continuous stands of conifer forests, leaving only degraded chaparral. Widely scattered groves of bigcone spruce, Pseudotsuga macrocarpa , obviously scarred and damaged by fire, were taken to be relics of former great forests, although the species was probably always confined to little habitat islands in a sea of chaparral. It is the only conifer in the region that can survive having its crown scorched and defoliated.
During the early part of the twentieth century, fighting forest fires became a moral crusade, and during the 1920s and 1930s enough technology and manpower was thrown into the fight that suppression became extremely effective. Foresters eventually realized, however, that they had grabbed a tiger by the tail. The longer the fire was postponed, the denser the forest became, the more fuel accumulated, and the harder fire was to control. When one finally got away, usually under the worst possible fire weather conditions, the larger and hotter the burn. By the time policy shifted from indiscriminate fire suppression to controlled burning, it had become extremely difficult to make headway against the huge areas of aging forests with heavy fuel loads. As a temporary expedient, most fires in western forests are still being suppressed.
Fire suppression has had very uneven effects on distributions of different species. Effects have probably been least in very high elevation forests of Pinus contorta and Abies lasiocarpa and other subalpine species, where fire suppression generally began only about 50 years ago, recovery from past long-interval crown fires is slow, and the mosaic pattern of different-aged stands still persists. Changes have been most marked at the other extreme, namely, in lower elevation ponderosa pine woodlands and savannas. There, the end of ignition by Indians and the onset of livestock grazing may have ended burning long before effective government intervention in fire suppression. Also, grazing favors pine reproduction by reducing competition with pine seedlings by the grass. As a result, former pine woodlands and savannas now commonly have a dense understory of young pines. Toward their upper limits, some formerly pure ponderosa pine stands are being invaded by Douglas fir.
In the Sierra Nevada, Sequoiadendron groves that were kept open by
surface fires have been strongly invaded by young white fir, Abies concolor , not only preventing bigtree reproduction but threatening the old trees with immolation.
Conifers also invaded areas formerly dominated by oaks. In Oregon's Willamette Valley, extensive park savannas with scattered Quercus garryana and Q. kelloggii have been invaded by dense stands of young Douglas fir. The namesake of the tree, David Douglas, recorded Indian burning of these savannas in 1826. Similarly, when Indian fires were stopped in Yosemite Valley, meadows with scattered Q. kelloggii trees were soon invaded by dense stands of ponderosa pine and other conifers. Overgrazing of the meadows by livestock probably accelerated this invasion, but it continues today even where meadows are not grazed; the National Park Service currently uses frequent prescribed burning to prevent conversion of the meadows to conifer forest.
The other side of the coin has been retreat of pioneer species. In several national parks in the Rockies, Populus tremuloides groves are senile and dying out. They were formerly regenerated by hot fires and had a headstart on conifers by root sprouting, but in the absence of fire, the clones have a short life. In Sierra Nevada bigtree groves, shade-intolerant shrubs, such as bitter cherry, Prunus emarginata , snowbush, Ceanothus cordulatus , and manzanita, Arctostaphylos patula , have died out under dense canopies of young Abies concolor . Fire suppression has not lasted long enough to cause retreat of fire-dependent conifer species. Although their reproduction has been suspended, most are so tall and long-lived that loss of territory by successional replacement would require centuries. it is now recognized that postponement of fires that long is unlikely.
A more immediate concern is possible conifer mortality due to abnormal fuel accumulation. Replacement of a mosaic of largely immature stands by a homogenized blanket of aging forests or brush may make formerly tolerable fire so intense as to be lethal. The most vulnerable species would be those with poor seed dispersal that depend on survival of old trees for subsequent reproduction. It is ironic that Pseudotsuga macrocarpa , which fire suppression was expected to restore to former imaginary abundance, may have lost ground because of fire suppression. Minnich (1977, in press) has evidence from sequential aerial photography and ground study that P. macrocarpa groves that recovered from earlier, smaller fires have been wiped out by hotter fires.
A quite different road to extinction may be traveled by Tecate cypress, Cupressus forbesii . As with Pseudotsuga macrocarpa , its natural pattern consists of small groves scattered throughout a sea of southern California chaparral. Unlike bigcone spruce, however, Tecate cypress trees are killed by fire but recolonize the burns with seed stored in closed cones until scorched. However, the trees do not produce any cones until they are about 10 years old.
Zedler (1977) believes that the natural return interval of fire in the Tecate cypress habitat is more than 40 years. Recently, human set fires have burned some of the groves repeatedly at much shorter intervals than that; some groves are unlikely to survive continued unnaturally frequent fire.
Retreat of Proteaceae, South Africa
(Vogts, 1982)
In the Cape of Good Hope region, the native sclerophyll scrub or fynbos had a rich endemic flora including many Proteaceae. The shrub species characteristically were strong colonizers of burns, seed being released from dormancy by the fires. Originally, the brush probably burned at intervals of a decade or a few decades, long enough for an accumulation of stored seed but within the life span of the shrubs. Presumably, vagaries of fire patterns caused some micromigrations, but species patterns were in rough equilibrium.
In the early colonial period, European settlers commonly increased the frequency of burning so greatly that the fynbos species lost much ground to grasses and other herbaceous vegetation. More recently, efforts to conserve remnants of the fynbos have involved fire suppression. Often fire frequency was pushed to the other extreme, being delayed beyond the normal life span of the shrubs, which thus lost more ground. For example, the spectacularly beautiful blushing bride (Serruria florida ) had declined to a single population in the Franschhoek Mountains. When in 1930 the number of plants declined, the cause was attributed to frequent fires and the area was rigidly protected. In 1962, not a single plant could be found in the protected area and only a few outside it. The species appeared to be about to become extinct in nature, although it was being cultivated in gardens. Then, an accidental fire swept the protected area, and seed stored underground germinated and new plants were established. The so-called marsh rose (Orothamnus zeyheri ) also became nearly extinct until discovery that reproduction of the few surviving plants was favored by burning.
The South African ecologists are studying proper fire intervals to encourage regeneration of different species of Proteaceae. Not only individual Proteaceae species but whole communities are adversely affected by the total absence of burning. In the Marloth Reserve at Swellendam, an area dominated by Proteaceae was fenced off and protected from fire and alien vegetation for 50 years. By then, Proteaceae were becoming senile and the area was being taken over by other fynbos species.
Eucalyptus Forest Dieback, Western Australia
(Shea 1979, 1982)
More than 1.5 million ha of lateritic uplands in southwestern Australia originally had a tall forest of jarrah, Eucalyptus marginata . The forest was rather open, with Acacia spp. dominating the understory, and was subject to occasional medium-intensity fires that did not kill the jarrah. Since early in the nineteenth century, jarrah forests have been logged for their fine timber. Fire was uncontrolled until World War I, when a policy of complete fire suppression was begun. After 35 years of suppression, it was decided that the policy was a failure because the forest was adapted to fire. For the next 25 years, prescribed low-intensity burning of the whole jarrah area was instituted on a 5- to 7-year cycle. This tended to eliminate the Acacia understory, which needs hotter fires to stimulate seed germination. The understory became dominated by Banksia spp., formerly minor components.
Jarrah dieback began in small areas soon after 1900, expanding slowly until the 1950s, when it accelerated. In 1966, the cause of the disease was finally identified as Phytophthora cinnamomi , a saprophytic soil fungus and root parasite. The fungus is believed to be native to southeastern Asia, Indonesia, and Queensland, but it is common in much of the world on many host plants. Why it did not reach Western Australia and attack the jarrah earlier is a puzzle. Possibly it had to wait for logging and disruption of the original fire regime. There is evidence that the fungus is spread by logging trucks. Also, the replacement of Acacia by Banksia in the understory evidently favored the fungus. Acacias are not susceptible to Phytophthora and emit soluble compounds that suppress it. Banksias are highly susceptible, their roots acting as pathways for spread of the fungus. Death of banksias and jarrahs raises soil temperature and favors fungal infection. In patches of the forest, all jarrahs of all ages have died, but mortality varies greatly with local edaphic conditions. Whether the dieback can be controlled by changes in fire regime remains to be seen.
Comment
Prehistoric human effects on forest fire regimes are outside the scope of this section. Also excluded are historical cases where native forests have been replaced by forestry nurseries and tree plantations. In the cases dealt with here, recent human intervention in fire regimes has had relatively subtle effects on tree species distributions.
Artificially increased frequency of burning, if continued longer, might
eventually cause more drastic retreat and even extinction of species too slow to mature and reproduce, like the Tecate cypress.
In the United States, the policy of complete forest fire suppression ran its course in far too short a time to eliminate most fire-dependent tree species. It would probably have been impossible to postpone fires beyond the life span of the tall fire-dependent conifers. In some places, fire has been postponed long enough to convert the former mosaic of different-aged forest patches to a homogeneous blanket of old growth where any wildfire would be extremely intense. It is not clear how much effect this would have on species distributions. Fire suppression has caused local retreats of some short-lived pioneer trees, such as the trembling aspen, but these species also have populations in other kinds of habitats, and there is no threat of their becoming regionally extinct.
The vagaries of natural fire regimes were evidently such that trees did not become rigidly adapted to a precise timetable. Moreover, many individual species have such broad ranges and occupy such diverse sites within those ranges that they had to have flexibility in surviving and exploiting fires.
Some South African fynbos endemic shrubs were so restricted geographically that extinctions by changes in fire regimes are conceivable. However, the gradual disappearance of species observed in old brush when fire is artificially excluded does not prove they are becoming extinct. Perhaps they can survive on unburned sites for long intervals as dormant seed.
Altered River Flood Regimes
High Plains, North Dakota and Nebraska
(Johnson et al. 1976; Williams 1978)
In North Dakota, the formerly wild Missouri River has been converted to a series of reservoirs and stabilized channels with controlled flow. Dam construction, primarily for flood control downstream, was completed mainly in the 1950s and 1960s. The extensive floodplain formerly had a dynamic pattern of deciduous hardwood forests. During the great spring floods with snowmelt in the Rocky Mountains, the meandering channel swept away patches of established forest and deposited fresh alluvium to be colonized by pioneer trees. The first of these were seedling willows and cottonwoods, particularly Salix amygdaloides and Populus deltoides . If these were left undisturbed by the river in later years, they were invaded by ash, Fraxinus lanceolata ; box elder, Acer negundo ; elm, Ulmus americana ; and finally, burr oak,
Quercus macrocarpa . Since damming, river meandering has all but ceased and no young willows or cottonwoods are establishing. Flooding has been eliminated on areas more than 2 m above mean river level, thus decreasing moisture and nutrients on most of the former floodplain. Elm and box elder reproduction are declining, and there has been a significant decrease in growth rates of all the floodplain forests. It is expected that in the future, all the species will retreat to fragmented remnants of their former distributions.
Change in riparian vegetation has been quite different along the Platte River system in western and central Nebraska. Explorers and travelers in the 1800s reported that islands in the river were heavily wooded with willows and cottonwoods but that the riverbanks had hardly any trees, perhaps because of prairie fires. At that time, the river flooded wildly in spring and was commonly completely dry in late summer. Dam construction began in 1970, primarily for irrigation rather than downstream flood control. Comparisons of channels in 1865 and 1969 over a 480 km reach show a reduction in channel width by 90% in the upper reaches and 30% in the lower reaches. Peak discharges and annual discharges have been greatly reduced, but return seepage from irrigation keeps the river flowing through the summer in the lower reaches. Time-lapse photographs show a huge increase in vegetation in the former channels and along the banks. Sandbars in former channels were colonized by sedges and grasses, followed by willow, cottonwood, elm, ash, box elder, and hackberry, Celtis occidentalis .
Similar dramatic time-lapse changes below dams have been recorded on several other High Plains rivers (Williams and Wolman 1984).
Dammed Rivers, Arizona
(Graf 1982; Turner and Karpiscak 1980)
Before Glen Canyon Dam was completed in 1963, the discharge and water level of the Colorado River running through the Grand Canyon varied vastly from season to season and year to year. Since the dam was built, these variations have been almost eliminated in normal years; discharge has varied mainly on a daily cycle with demand for electrical power from the generators.
Predam vegetation was recorded by a long series of river-running expeditions; starting in 1871, excellent photographs were taken. Ten years after Glen Canyon Dam was built, the National Park Service began rephotographing the riparian vegetation from the same camera stations as the old pictures. These matched pairs show two different effects of the dam on plant distribution.
First, species that were locally present above the former floodline have colonized the zone below the line that was formerly scoured clean of plants.
For example, Acacia greggii, Prosopis glandulosa , and Brickellia longifolia , which grow on stabilized talus and sand above the former floodline, now have abundant seedlings below it. These are not strictly riparian species.
Second, a different pattern of migration has been the spread of truly riparian species along the new daily high waterline into reaches where they were previously unable to withstand the scouring action of floods. Some of these are natives of the general region, for example, cattails, Typha latifolia and T. dominguensis ; common reed, Phragmites australis ; willows, Salix exigua and S. goodingii ; cottonwood, Populus fremontii ; arrowweed, Pluchea sericea ; and seep willows, Baccharis glutinosa and B. emoryi . Seeds of all these may have blown and floated along the main river; some probably also invaded from tributary canyons. The cottonwood, for example, was present before the dam only in tributary canyons in the Grand Canyon region.
Three recently introduced Eurasian shrubs also spread along the new waterline: camelthorn, Alhagi camelorum ; Russian olive, Eleagnus angustifolia ; and tamarisk or salt cedar, Tamarix spp. Invasion by tamarisk of natural riparian habitats in the southwestern United States was discussed in Chapter 1. Tamarisk was present along much of the Colorado River, including the Grand Canyon, by the 1930s, but only in widely scattered sites. The Glen Canyon Dam allowed spectacular expansion of Tamarisks as a dense, almost continuous fringe along the daily high water mark.
After 20 years under control, in June 1983 the Colorado River, fed by phenomenal snowmelt, overwhelmed the storage capacity of Lake Powell behind Glen Canyon Dam. The Bureau of Reclamation opened the spillways on the dam. By June 25, 1983, the river was destroying vegetation and removing beaches up to the predam floodline. The effects await restudy.
Tamarisk has also benefited from controlled flow below dams on other Arizona rivers. Before the 1920s, the middle Gila River and its tributary, the Salt River, were perennial streams. Their banks were lined with willows and cottonwoods, and the sand bars temporarily colonized by seep willows and arrowweeds. After dam construction, water was diverted for irrigation rather than stored for power generation, and surface water flows below the dams were reduced to rare floods. Much of the former channel was occupied by tamarisk, forming dense and extensive thickets. Tamarisk was mapped over a 100-km reach of the river from aerial photographs taken at seven different times between 1937 and 1979. These show that the main spread was completed by the early 1940s; since then, there have been great fluctuations in the tamarisk populations. Some have died out during periods when the channel had a very low groundwater table; others have been ripped out in the rare floods, but colonization has been rapidly resumed.
Tamarisk has also benefited throughout the Southwest from sedimentation behind dams; it forms dense jungles on delta deposits at the heads of reservoirs.
Mississippi River Deltaic Plain
(Baumann et al. 1984; Becker 1981; Coleman 1966; Coleman and Gagliano 1964; Russell 1967)
Prior to construction of artificial levees, the Mississippi River behaved much like the uncontrolled Mexican rivers discussed in Chapter 1. Since the postglacial eustatic rise of the sea approached the present level about 5,000 years ago, the main river channel has wandered back and forth across the great deltaic floodplain over an east to west span of more than 250 km. During this time, the river built six different deltas, each containing several subdeltas where an active distributary was located for about a century. The total land area did not increase greatly during this time because, while sediment deposited during floods was building new natural levees and marshes at active river mouths, subsidence and coastal erosion caused loss of land in the rest of the region. This is because continued loading of sediment causes crustal downwarping and compaction of the thick, ancient sediments under the whole plain. Local plant colonization followed advancing natural levees, beach ridges, and freshwater marsh development. Cores from deep borings through sediment sequences show that when a distributary was abandoned and input of fresh river sediment was shut off, formation of organic peat by marsh plants sometimes kept pace with subsidence for a while, but eventually large open water bays developed. Wave erosion along their shorelines caused rapid retreat of plant species until the next cycle of deposition began.
Since the foundation of the city of New Orleans on the current main river channel, artificial levee construction has brought the river under increasing control; by 1920, it was considered to have been tamed. A phenomenal flood in 1927 led to even more massive engineering, and the river was walled in by great mainstream levees along its entire length. The one major opening above New Orleans has been the Atchafalaya River, which branches off the main river 80 km above Baton Rouge. The Atchafalaya has taken an increasing part of the Mississippi discharge every decade since 1860. By now it would have become the main mouth of the Mississippi if the U.S. Army Corps of Engineers had not restrained the diversion. They currently allow a maximum of 30% of Mississippi floodwater to be captured by the Atchafalaya. This supplies fresh sediment to the marshes and swamps of the great Atchafalaya basin, and a new subdelta is being built into the Gulf at the river mouth. The new mudflats are being colonized by Salicornia, Spartina , and other salt marsh plants. Eventually, the subdelta should build out about 50 km onto the shallow continental shelf and provide extensive new habitat for freshwater marshes and cypress swamps.
However, this local accretion of new land is not offsetting losses elsewhere.
Levee construction has kept the main flow of the river down the same old channel past New Orleans for so long that the active subdelta was extended out to the edge of the continental shelf. Every year since then, hundreds of millions of tons of alluvium have been wasted by being dumped into deep water instead of building new land. This weight still causes crustal downwarping and regional subsidence accompanied by expansion of open water and shoreline erosion. Loss of sinking marshland has been accelerated by digging of canals for navigation and oil exploration; these have helped expose marshes to severe damage during hurricane passages.
By 1960, net losses of Louisiana wetlands to open water were estimated at over 40 km2 /year and by 1980 at about 130 km2 /year; losses are accelerating. Bald cypress, Taxodium distichum , and freshwater marsh plants are the major losers. Salt marsh species have shifted landward. Also, beach plants often persist along a retreating coast, riding on sandy beach ridges that are pushed landward by the advancing sea.
Comment
Artificial levees on the Mississippi River deltaic plain have had profound and complex effects on the plant geography of the region. By contrast, case histories of dam building show rather simple, predictable effects on riparian plant patterns. Elimination of floods and evening out of flow through the year narrow the zone in which riparian species can live and at the same time make this narrow zone more continuous. However, if the dams are incompetent to handle great floods, the continuity may be only temporary, and there may be episodes of decimation and recolonization along the riverbanks that are longer in time scale than in the original, natural regimes.
The available case histories do not suggest that engineering works have been responsible for changes in gross species ranges. Rather, there has been local repatterning of species that were present in natural habitats within the region.
5
Discussion Of Modern
And Historical
Migrations
In the abstract model presented in the Introduction, geographic patterning of seed plant species is the product of interplay between opposing forces tending to expand or restrict the distribution. The main centrifugal force is usually seed dispersal; the main centripetal force is environmental selection. When dispersal and environment are sufficiently stable, fluctuating within normal limits, these forces eventually equilibrate and the species borders become static. Migration, whether advance or retreat, occurs when equilibrium is upset by changes in dispersal or environment or both. The case histories that have been discussed thus far show a variety of migration patterns resulting from such changes.
Changes In Dispersal
Changes in dispersal alone, with no change in environmental selection, can result in both local and long-range colonization. An example of local colonization is the arrival on Baltic islets of new plant species after a change in the foraging grounds of gulls nesting on the islets. An example of long-range colonization is the introduction from overseas of ruderal plants with wool imported to France. New colonies produced by such long-range dispersal are commonly ephemeral and nonreproductive. However, there are also a great
many cases of successful establishment of widely disjunct populations following long-range dispersal. Most of these are weeds of artificially disturbed habitats or wild pioneers of seashores, streambanks, and other naturally disturbed habitats, but there are also cases of introduced exotic plants invading undisturbed native vegetation, both on remote islands and on continents.
As would be expected, recent establishment of new, widely disjunct colonies is mainly due to human introduction, the major new agency of long-range dispersal. Only occasionally are new colonies attributed to natural long-range dispersal, for example, the arrival of an orchid, Spiranthes romanzoffianum , in the British Isles in the nineteenth century by wind-borne seed from North America. For two reasons, however, formation of disjunct colonies by natural long-range dispersal may be going on without being recognized. First, the mode of dispersal may be unrecognized. For example, if plant species were to arrive in Hawaii by ocean, wind, or bird dispersal in the present day, as they undoubtedly did many times prehistorically, they would inevitably be assumed to have been artificially introduced. Second, the recency of the colonization may be unrecognized. For example, Madroño: A West American Journal of Botany regularly has a section reporting new collections that extend the known ranges of native plant species. Some of these involve disjunctions of only a few tens of kilometers, but many report populations several hundred kilometers outside the formerly known ranges. These are normally assumed to have been simply newly discovered rather than newly established.
None of the case histories showed retreats of species due to decreased dispersal. Conceivably, extinction of animal species could have caused contraction of seed shadows of plant species, followed eventually by dying out of marginal colonies outside the reproductive core of the plant species. Perhaps this occurred with oaks and other trees that provided mast for the North American passenger pigeon or with aquatic plants with seeds carried in the fur of the European beaver, but no examples are known. A more extreme case, in which the whole population of a plant species may have ceased to reproduce after extinction of an animal, involves two species endemic to Mauritius. The dodo, a giant, flightless relative of the pigeon, became extinct about 1680, soon after the island was colonized by the Dutch. Temple (1977) suggested that a tree species, Calvaria major , was totally dependent on the dodo for reproduction. Calveria was originally common on the island and was cut for lumber in the early colonial period but is now nearly extinct. Only 13 trees survive, all believed to be over 300 years old. Temple fed some of the thick-shelled Calvaria seeds to domestic turkeys as stand-ins for dodos; the seeds germinated after passing through a turkey gizzard.
Changes In Environment
In a great many cases, especially but not exclusively local migrations, advances and retreats are evidently due to changes in the environment, while any dispersal changes appear irrelevant. A change in a single factor in the plant environment, such as introduction of a new herbivore, a new disease, or a new fire regime, commonly causes retreats of some species and advances of others. Such linked migrations are not necessarily on the same scale; the catastrophic retreat of elms in North America was accompanied only by micromigration of the species that replaced them. Sunflowers marched across the continent along roadways that had pushed previous plant occupants back only a few feet.
Disjunctions in species ranges can result from environmental change both by colonization of isolated new habitats and by fragmentation of formerly continuous distributions. Examples of the former include new islands emerging within the reach of sea-dispersed beach plants and artificial marshes formed under the flyways of migratory waterfowl. Many plant distributions have been fragmented by clearing and grazing. Dramatic recent changes in plant environments, like changes in dispersal, have been largely but not exclusively due to human activities.
Changes In Both Dispersal And Environment
Some cases of plant migration involve interconnected changes in dispersal and environment. For example, herds of sheep driven into the Great Basin of western North America evidently carried in their wool seeds of Eurasian grasses that invaded ranges overgrazed by the sheep. On Krakatau, the establishment of figs and other trees dispersed by birds and fruit bats evidently attracted more of those animals to the island and started a positive feedback between forest development and animal introduction of more tree species.
Part II
Prehistoric Migrations
There is a large body of literature in biogeography that attempts to deduce prehistoric migrations of taxa solely from their present distribution patterns. Strangely, such deductive reconstructions, by long tradition, have come to be called historical plant geography, although as Stott (1981) pointed out, they are not generally based on any genuine historical evidence. Some of these reconstructions assume that through geologic time plant ranges have simply expanded and contracted around centers of origin or refuge. Where present distributions of taxa are disjunct, there has been endless contention between proponents of two polar a priori explanations: long-range dispersal versus fragmentation of former continuous ranges. Less simplistic deductions based on consideration of the biology of the taxa and the known paleoecology of the region are more plausible and interesting. In the absence of any actual evidence of past distributions, however, there is no way to confirm the time or spatial scale of the postulated migrations. A scholar dealing with a predetermined cast of characters without a fossil record may have no alternative but to speculate about their migrations. Here, the cast of characters is not predetermined; as in Part I, case histories will be chosen for which there is actual time depth in the evidence.
The transition from written history to prehistory requires a change in approach. It is no longer feasible to organize cases under habitat types. In the fossil record, environment is not a matter of observation but of interpretation. Reconstruction of paleoenvironments is not a starting point but a goal of research. The starting point here will be fossil assemblages from particular geologic time spans and particular regions. Little attention will be given to inferred paleoenvironments. This is certainly a subject of profound interest in its own right. For purposes of tracing plant migrations, however,
it is more appropriate to deal with the plant taxa directly. Using paleoenvironments inferred from fossil distributions to explain fossil distributions is logically quite circular.
The chronology will be worked backward because the more recent periods have the most complete and least problematic fossil record. While moving forward from the origin of seed plants in the Paleozoic might appear more straightforward, it would not actually give a more coherent story, simply because the record is too discontinuous.
6
Last Glacial
And Holocene
This period offers the most readable chapter in all of paleobotany. Any fossil evidence, however, involves formidable problems not shared with modern historical evidence. These problems have been carefully examined by Davis (1978) and Watts (1978). Only a few of the more intractable ones will be briefly noted here.
Identification of Taxa . The basic units of the fossil record are not ordinarily whole plants or assemblages of organs, as on herbarium specimens, but disarticulated parts. The great bulk of the seed plant record consists of pollen grains, the domain of palynology. The most abundant macrofossils are usually individual leaves or leaflets. Plant fossils of this period are generally considered to belong to living species, but their pollen and leaves have often been identified only to genus or family. A great deal of talent and effort are currently being devoted to better identification, partly by use of scanning electron microscopy.
Dating . The pioneer palynologists made an assumption that is no longer considered tenable, namely, that during Late Glacial and Holocene time, major vegetation changes were synchronous over large regions, perhaps globally, due to changing climate. Before absolute 14 C dating was available, changes in fossil floras were used to define time levels and thus to correlate dating between different sites and regions. This led to circular reinforcement of the idea of synchronous climatic control of plant migration. Forunately, development of isotopic dating has opened the way for a true chronology not derived from changes in fossil floras.
Displacement . Transport of pollen and other plant parts by wind and water may leave fossil records far outside the range of the species. Water-worn fossils can sometimes be recognized, but wind-borne pollen is not altered by its journey. Thus, if fossil pollen of a taxon is present at a site in low frequency, it can be interpreted as either an abundant species far away or a rare one nearby. For example, in eastern North America, beech, Fagus grandifolia , is recorded during the Late Glacial by macrofossils from two sites in the lower Mississippi Valley and by pollen from several sites farther east. The pollen is present in extremely low frequencies and is usually interpreted as having come from a few distant, highly localized refugia in the lower Mississippi Valley or elsewhere. However, Bennett (1985) prefers to believe that beech was very widespread but very rare all across southeastern North America. There are not enough Late Glacial pollen sites yet known in the region to resolve this question. Discrimination between distance and abundance as controls of pollen frequency is possible when many sites in different parts of a region can be compared. Uniform low frequencies of a given pollen type suggest a background pollen rain from a distant source, while locally variable frequencies suggest presence within the region. This deduction is strengthened when formerly constantly low frequencies rise abruptly in a geographic sequence, which evidently documents local arrival of an advancing species border.
Palynologists face other difficult problems in attempting to translate pollen frequencies into vegetational reconstructions because of great differences between species in the quantity of pollen produced and preserved. In its naive early days, palynology seriously underestimated the difficulty in translating fossil pollen data into geographic patterning of plant species and their environments. Dawning awareness of this difficulty has led to admirable advances in research techniques and interpretation of data. Whatever its remaining imperfections, modern palynology is infinitely better for reconstructing prehistoric plant migrations than the untestable speculations that prevail in the absence of a fossil record.
Northwestern Europe
(Bell 1969; Bertsch 1953; Beug 1982; Butzer 1971; Edwards and Hirons 1984; Frenzel 1968; Godwin 1956; Hammen et al. 1971; Huntley and Birks 1983; Iversen 1949, 1960; 1964; Kral 1972; Markgraf 1972; Pennington 1969; Prentice 1983; Ralska-Jasiewiczowa 1983; Tallantine 1972)
The reconstruction of plant migrations in northwestern Europe during this time span forms a classic chapter in paleobotany. It is the work of a large
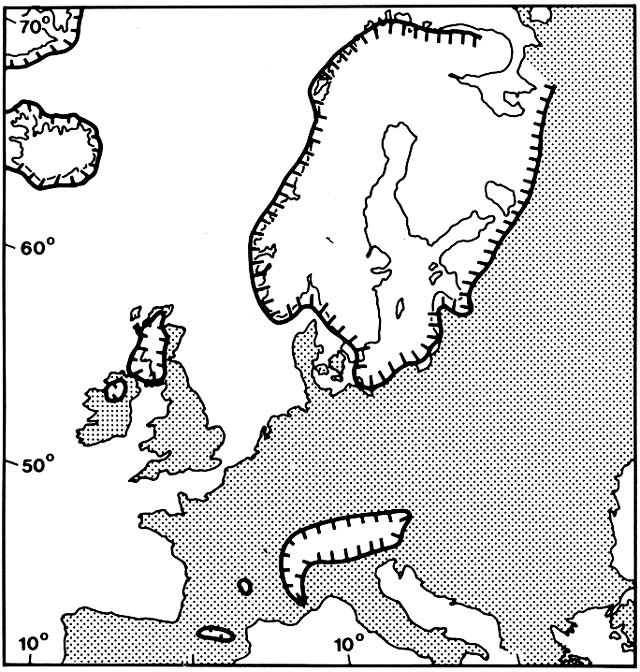
Figure 7. Areas of Northwestern Europe Covered by Glacier 12,500 Years Ago. Modern coastlines are shown
for orientation, but the sea then stood far below its present level. (Adapted from Huntley and Birks, 1983)
corps of scholars in various countries. They had a magnificent fossil record in the abundant bog and lake deposits of the region. Also, the simplicity of the forest flora of the region aided accurate identification of fossils and ecological interpretation. The same tree genera that are represented elsewhere in the northern hemisphere by multiple species are here represented by single or few species.
Late Glacial: Dryas I
The beginning of the Late Glacial, which terminated the Weichsel glaciation, is conventionally dated at about 14,000 B.P. (before the present) (Table 1). The great continental ice sheet that had formed about 60,000 B.P. began to retreat from its Full Glacial maximum. In the Late Glacial, it still covered almost all of Fennoscandia and much of the northern British Isles (fig. 7). Sea levels were still low; the North Sea and the English Channel were dry land. South of the continental ice sheet, mountain glaciers were extensive in all the ranges as far south as Spain, Italy, and Greece. Boreal forests were extensive in eastern Europe, but northwestern Europe had only scattered patches of sparse, open woodland. Barren outwash plains, flooded periodically during early summer, were extensive; they were sources of massive wind-borne loess deposits. Much of the region was dominated by grasslands where soil processes were similar to arctic tundras, with solifluction and frost heaving above permafrost.
The Dryas I vegetation, however, has no exact modern analogue, either in the arctic tundras or in the cold, dry steppes of central Eurasia. Its component species have since gone their separate ways to a variety of regions and habitats. The woodland trees, species of Picea, Larix, Pinus, Betula , and Populus , are now found in the boreal forest. Some shrub and herb species have retreated to arctic and alpine tundras, for example, Dryas octopetala, Betula nana, Salix herbacea , and Koenigia islandica . Many others are now pioneers of seashores, cliffs, stream gravels, and other naturally disturbed sites over a wide climatic range, for example, Juniperus communis, Ephedra distachya, Suaeda maritima, Triglochin maritima, Hippophae rhamnoides , and Helianthemum oelandicum . Many members of the Dryas I herb flora are now common weeds, for example, Rumex acetosella, Chenopodium album, Plantago major, P. lanceolata, Daucus carota , and Centaurea cyanus . During Dryas I, periglacial processes provided the disturbed, exposed ground needed by a heterogeneous assemblage of pioneer plants. The Dryas I vegetation supported a great mass of great herbivores, including woolly mammoths, woolly rhinos, muskoxen, reindeer, and the Paleolithic hunters that pursued them.
|
Allerød Interstadial
The brief warmer period during the Allerød Interstadial had rapid glacial melting. Sea level rose to within about 40 m below present level, but the North Sea area remained land and the Baltic region had a great freshwater lake. Many small lakes began accumulating organic sediments over the former inorganic deposits; they were colonized by aquatic plants, such as Myriophyllum alterniflorum , probably brought north by waterfowl. Dryas, Juniperus , and other pioneers advanced into deglaciated territory, but gained less territory than they lost to northward-advancing trees. Tree birches, Betula pubescens and B. pendula , spread over large, formerly treeless areas as far as Britain and Denmark. Woodlands of Populus tremula and Pinus sylvestris expanded in southern Germany and the Alps, where the tree line rose to only about
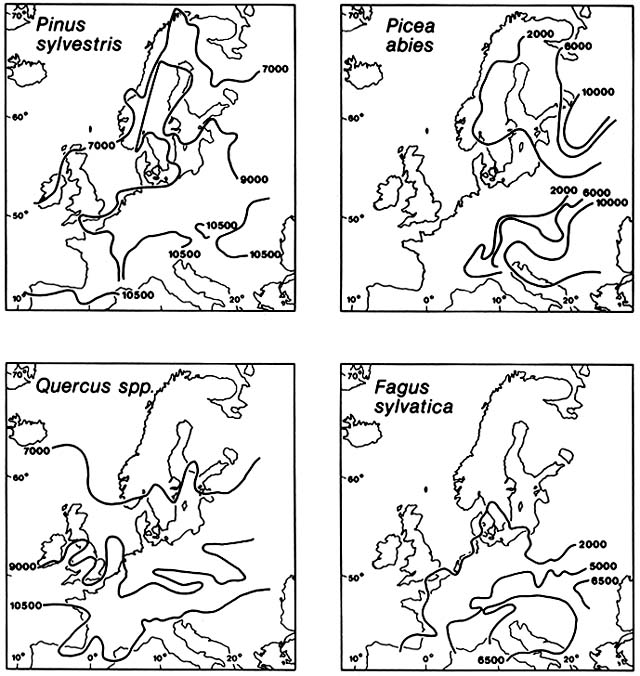
Figure 8. Postglacial Immigration of Selected Trees into Northwestern Europe. Advancing fronts are shown
at specified numbers of years before the present. Subsequent losses of territory are not shown. During the early
Postglacial, Britain was not yet an island. Each tree species migrated individually at its own rate and along a
peculiar pathway. Migration rates were commonly so fast that human inhabitants of the region must have been
well aware that the species borders were moving. (Adapted from Huntley and Birks, 1983)
500 m below its present level. In the Carpathians, Picea abies expanded its range. Woolly mammoths and rhinos became extinct during the Allerød, while muskoxen retreated to the far north. Some reindeer survived in the region and moose increased in abundance, often being the main game of the Paleolithic hunters.
In deposits of late Allerød age, charcoal horizons were associated with high frequency of pollen of fireweed, Epilobium angustifolium . Some scholars have suggested that extinction of the large herbivores and deliberate forest burning can be attributed to the Paleolithic Late Magdalenian peoples. Advance of the forests during the Allerød must have reduced the habitat of the large herbivores and made them vulnerable to overhunting. It is also possible that climatic cooling at the end of the Allerød led to death of the forests and the conflagrations recorded by charcoal horizons.
Dryas II
During the Dryas II glacial stadial, glaciers readvanced, sea level dropped, and forests were replaced by tundra and steppe over much of the region. This final Weichsel glacial stadial lasted about 1,000 years before its abrupt end.
Holocene: Preboreal
During the first subdivision of the Holocene Epoch, the Preboreal, the continental glacier melted back to separate residuals in northern Sweden and Norway. Glaciers in the Alps retreated to about their present extent. Lakes as far north as the Baltic region were rapidly colonized by Cladium mariscus and other warmth-requiring aquatics. Juniperus communis and other pioneer shrubs expanded rapidly north of the Alps, in the northern British Isles and northern Scandinavia. Juniper dominance was very brief before it was over-whelmed by advancing tree birches and poplars, such as Betula pubescens, B. pendula , and Populus tremula . From scattered Dryas II relic stands, these expanded to regional woodlands. Pinus sylvestris began moving northward from the Alps, but had not yet caught up with the birches. Picea abies began spreading slowly westward in the southeastern Alps and Carpathians, but it remained in the mountains (fig. 8), leaving the Danube and Pripet lowlands uninvaded. During the Preboreal, the tree line in the Alps rose to approximately its modern level.
Warmth-requiring deciduous hardwoods, which had probably become extinct north of the Alps during the Weichsel, began to reinvade. Of these, hazel, Corylus avellana , moved the fastest, advancing from Italy northwestward
across France to Britain, which was not yet an island. Hazel then looped eastward across the low countries to the Baltic. By 9,500 B.P. , hazel was approaching its present limits in northern Scotland and Scandinavia. In parts of northern Europe, hazel had outrun pine. During the same time span, deciduous Quercus spread from Spain and Italy across western France to southwestern England, while Alnus, Tilia , and Ulmus were still advancing through France. By the end of the Preboreal, the open steppe and tundra plants had been driven back by the advancing forests and woodlands to scattered patches. Reindeer were gone from most of the region.
Boreal
Pinus sylvestris caught up with the boreal tree birches and poplars, driving them back to scattered remnant patches, and was approaching its present northern limits in Britain and Scandinavia. At the same time, pine was losing ground over much of the region to advancing temperate hardwoods. Hazel continued to move eastward into Poland and also pushed northward into deglaciated parts of Fennoscandia. The oaks, Quercus , continued to move rapidly northward through Britain and Ireland and also up the Danube and across central Europe. Lindens, elms, and ash trees, Tilia, Ulmus , and Fraxinus spp. had moved past the Alps more slowly and were still advancing across France and Germany far south of their present limits at the end of the Boreal. Meanwhile, alders, Alnus spp., were invading poorly drained areas. An undetermined species of alder made a tentative advance into northwestern France early in the Boreal, but this migration stalled for unknown reasons. At the same time, far to the east both Alnus glutinosa and A. incana pushed north from southeastern Europe clear into Fennoscandia. Picea abies continued to expand slowly westward in the mountains of central Europe but did not move out onto the plains. Abies alba finally began moving northward into the Alps, presumably coming from a glacial refuge in the Appenines.
Atlantic
During the Atlantic period, deglaciation of Fennoscandia was completed and eustatic sea level approached the present level. Britain and Ireland became islands. Encroachment of the rising sea onto the continental shelf must have extended the mild, humid maritime climate eastward. Hornbeam, Carpinus betulus , was restricted to Italy and the Balkans at the beginning of the Atlantic. By the end of the period, hornbeam had spread northward through Poland to the Baltic, joining the mixed deciduous hardwood forest. Meanwhile, alders continued to spread into deglaciated territory, especially in swampy carrs and fens. During the Atlantic, alders spread northward through
Fennoscandia to the Arctic Ocean and westward from the Baltic Sea into the Low Countries and England, then northward to Scotland, Ireland, the Faroes, and Iceland.
Another deciduous hardwood, beech, Fagus sylvatica , invaded the region more slowly around both flanks of the Alps. Early in the Atlantic, beech advanced from Italy into southern France and from the Balkans up the Danube Valley. By late Atlantic time, the eastern invasion had rounded the Alps and was moving westward through the central European mountains. Beech advanced selectively, favoring chalk soils and leaving the mixed oak forest in mesic sites.
Meanwhile, pine, birches, and other pioneer trees continued to be squeezed into edaphically and climatically marginal sites. Of all the Holocene subdivisions, the Atlantic had the most continuous expanse of late successional hardwood forest. In certain places, however, such as the Pennine plateaux of southern Britain, extensive blanket peat bogs, with Sphagnum moss and Eriophorum sedge, began replacing former hardwood forest. As for fir and spruce, during the Atlantic, Abies alba reached its present northern limits along the Rhine and began moving into the eastern Alps, where it met and overlapped with Picea abies moving in the opposite direction. After 3,000 years in the eastern Alps, Picea finally crossed the Rhine into the western Alps during Atlantic time. At the same time, a separate migration of Picea abies , presumably from a glacial refuge in Russia, reached the Baltic from the east.
Subboreal
During the Subboreal, there was large-scale replacement of forest by blanket bogs, heathland, bracken, and grassland. At the same time, beech finally moved out of the central European mountains and advanced at a startling rate across Poland to the Baltic Sea and across Germany and France to England. Meanwhile, the border of hornbeam remained nearly stationary in southeastern Europe, but hornbeam advanced from northern Poland westward across northern Germany. Spruce, Picea abies , crossed the Baltic into Scandinavia, but remained rare and local there during the Subboreal. Fir, Abies alba , completed its migration in the central European mountains, even surpassing its present borders in places, including the Alps, where it grew higher than the present timberline.
Subatlantic
Blanket bogs have continued to spread to the present time at the expense of woodland, especially in wet coastal and cool mountain regions. In general, the fossil record suggests fluctuating, patchy, local vegetational changes,
largely attributable to human activities, rather than progressive changes in species ranges. There are a few exceptions. After a long history of relatively slow advance, hornbeam began advancing rapidly about 2,000 B.P. Within 500 years the species advanced from western Germany across all of France to the Bay of Biscay. During the first half of the Subatlantic, spruce essentially completed its immigration into Scandinavia.
Changing Interpretation of Postglacial Migrations
In the early days of palynology, it was taken for granted that regional vegetational changes were controlled by climatic changes. Early Postglacial advances of tree species were thought to have been restrained by gradually rising temperature, allowing cold-tolerant boreal species to precede the warmth-demanding species. The northern European climate was considered to have improved to a so-called optimum in the Atlantic period, followed in the Subboreal by deterioration, as evidenced by decline in abundance or retreat of certain species. Essentially, the whole sequence was interpreted as a series of different climax vegetation types, each in equilibrium with changing climate. The conceptual model did not allow for successional or migrational lag.
There is no doubt that drastic climatic changes during the Allerød and again at the beginning of the Holocene set off great plant migrations. However, the assumption that the moving species borders were constantly in equilibrium with climatic controls was inevitably challenged. Bertsch (1953) pointed out a possible spurious correlation between cold tolerance and sequence of immigration of the dominant tree species. The most cold-tolerant trees, such as Pinus, Betula , and Alnus , are quicker to mature and more widely dispersed than the most warmth-requiring ones, such as Ulmus, Tilia, Quercus , and Fagus . Iversen (1960) reiterated this point and also noted that, because the boreal species are cold tolerant, they would have survived the Weichsel glacial at higher latitudes and thus, other things being equal, would have arrived first in Postglacial time. Furthermore, the first Postglacial immigrants were pioneer species, capable of invading open habitats. The later arriving dominants, although slower to mature and less dispersible, are longer lived and more shade tolerant; even under a constant climate, they might be expected to eventually replace the pioneers. Iversen (1964) later suggested that some vegetational changes after Atlantic time, which had been attributed to climatic deterioration, were due to gradual leaching of immature soils derived from base-rich glacial drift and also to accumulation of acid peat. In northern Europe, the possibility that forests can be successionally replaced by blanket bogs and heaths is now taken seriously by many ecologists.
It is possible that in early Holocene time, climate had changed so abruptly
from the glacial climate that there was a period of free migration before species could fill their new potential areas. During this free migration, location of the moving borders would have been largely controlled by how far seed was dispersed and how long it took for new colonies to mature and produce the next wave of seed. Many of the advancing tree species spread at almost incredible rates; their advances must have been very obvious to people inhabiting the region. Almost all of the dominant trees accomplished much of their advance at rates of over 300 m/year, averaged over centuries and millennia. Pinus, Corylus, Alnus, Ulmus, Acer, and Carpinus advanced at rates of over 1 km/year, averaged over centuries. Thus, all of these advanced at rates of many kilometers per generation. Explaining location of their borders does not require invoking climatic or other environmental controls. Rather, it demands long-range dispersal agents. North-flowing rivers and birds were probably crucial. Human dispersal probably was also, particularly in species with heavy, edible seeds, such as Pinus, Corylus, Quercus, and Fagus . Early Postglacial Europe was inhabited by so-called Mesolithic peoples. Unlike the specialized Paleolithic big game hunters of the glacial steppes and tundras, the Mesolithic cultures had a more diversified food base, including fishing, hunting deer, and gathering nuts and other vegetable foods. Archaeological caches of hazel and other nuts show they were commonly transported by the people.
Later, with the arrival in northern Europe of Neolithic pastoral and agricultural peoples, evidence of anthropogenic effects on plant distribution becomes much clearer. This began in the Subboreal period. Before 14 C dating the end of the so-called Atlantic optimum and the beginning of the Subboreal was defined by a change in abundance of pollen of certain tree species, particularly an abrupt drop in Ulmus . This so-called elm fall was accompanied by subtler and less consistent changes in abundance of other species for which rather vague climatic causes were suggested. Subsequently, 14 C dating revealed that the elm fall and associated changes in pollen of other species were not synchronous throughout the region, as had been assumed, but varied by several hundred years between different places. Moreover, it was found that the pollen record did not show unidirectional changes but rather complex local fluctuations, with forest trees alternating with birches and other pioneer trees. Along with increases in pioneer trees came resurgence of pioneer herbs that had disappeared from the fossil record in early Postglacial time, such as Rumex spp., Chenopodium spp., Plantago lanceolata, and Centaurea cyanus . Presumably, these had survived in naturally open coastal and riparian habitats as Postglacial forests took over most of the region and were preadapted to become weeds when the forests were opened by Neolithic pastoral and agricultural clearings. Subboreal pollen diagrams commonly show the first appearance of domesticated cereal crops at the same levels as resurgence of native pioneer trees and weeds.
Causes of the elm fall are still something of a puzzle. It is questionable whether Neolithic shifting cultivation in early Subboreal time was sufficiently extensive to cause a decline of the magnitude recorded. Palynologists and archaeologists, particularly in Scandinavia and Great Britain, have proposed that lopping of elm branches for fodder for cattle was largely responsible for the elm fall. Arrival of the fungus responsible for Dutch elm disease, Ceratocystis ulmi , has also been suggested. Huntley and Birks (1983) have neatly combined all three hypotheses. Spread of agriculture and the fungus may have coincided because injury to elms by Neolithic felling and lopping would have made the trees more susceptible to attack by bark beetles that transmit the fungal spores.
The puzzling acceleration during the Subboreal of the advance of beech, Fagus sylvatica , has also been attributed to human agency. Unlike its North American congener, the European beech is not usually able to invade undisturbed forest. Its sudden expansion coincides in time with the elm fall and the beginning of shifting cultivation. Later in the Subboreal, with the beginning of the Iron Age when plow farming moved onto heavier soils, beech may have expanded on abandoned upland fields on chalk and limestone.
It is widely recognized that northern Europe has had very little truly natural vegetation, in which species distributions have been under direct climatic control, since the Atlantic period. For Subboreal and later time, sorting out any signals of climatic change from the other variables affecting plant geography is a baffling problem. Some scholars, including Beug (1982) and Prentice (1983), are optimistic that this can eventually be accomplished, but it has not yet been done.
A special problem with attributing plant migrations to climatic change arises with freshwater aquatics. Some of these were much more extensive and abundant in deglaciated territory during early Holocene time than now, for example, Cladium mariscus, Trapa natans, Najas marina, N. flexilis, Ceratophyllum demersum, and C. submersum . Their retreats and local extinctions have been cited as evidence of climatic deterioration since the Atlantic optimum. It is curious that such retreats should be concentrated among aquatics, which are relatively indifferent to climatic gradients. Also, their losses of territory have been due to thinning out of the density of sites rather than to general southward displacement of the border of the gross ranges. Some of the winking out of colonies may be due to limnological changes as new glacial lakes became older.
Eastern And Midwestern North America
(Black and Bliss 1980; Davis 1965, 1978, 1981a, b, 1983; Dean et al. 1984; H. Delcourt 1979; P. Delcourt 1980; Delcourt and Delcourt 1977, 1981,
1984; Delcourt et al. 1983; Grimm 1983; Ritchie 1976; Vesper and Stuckey 1977; Watts 1983; Wright 1971, 1976)
After early naive attempts to find parallels with the European Late Glacial and Holocene climatic and vegetational sequence, North American workers have developed a story that is in many ways different and more complex.
Full Glacial
During the Wisconsinan Full Glacial, from about 25,000 to 15,000 B.P. , the continental ice sheet covered half of the North American continent, extending from the northern Rocky Mountains across what later became the Great Lakes to the Atlantic Ocean, where the southern margin on Long Island reached the latitude of Madrid and Naples (fig. 9). Instead of the great European tundra or steppe grassland, there was a very narrow and probably discontinuous treeless belt along the ice margin. Scattered mountain glaciers and alpine tundra extended far south into the Appalachian Mountains. Unglaciated North America east of the Rockies was mostly dominated by conifers now found in the boreal forest, although not in the same associations. Picea and Larix were dominant over a great area west of the Appalachians, and Abies on both flanks of those mountains. Unlike the modern boreal forest, much of the interior conifer forest, especially in the north, had no pine component. Pinus banksiana was present in parts of the Midwest and was dominant in much of the southeast, especially on a coastal plain that was much broader than now because of lower sea level. Much of Florida had dry scrub, savannas, and active dunes. A few hardier genera of deciduous hardwoods—Populus, Alnus, Betula, Carya, and Quercus —were widely associated with the dominant conifers, but the complex of broadleaf trees that dominate mature, mesic forests today were generally absent. Only recently have fossils of these from the Full Glacial been identified in the lower Mississippi Valley and eastward; presumably the species survived in especially favorable microhabitats, such as warm, south-facing gorges.
Late Glacial
During the Late Glacial, from about 15,000 to 10,000 B.P. , ice retreat began and continued without any interruption comparable to the Dryas II Advance in Europe. The deglaciated area was evidently initially colonized by pioneers from the formerly narrow tundra beyond the ice margin: many sedges, a few grasses, and several shrubs, including Salix herbacea, Vaccinium uliginosum, and Dryas integrifolia, a nitrogen fixer. In New England, it was
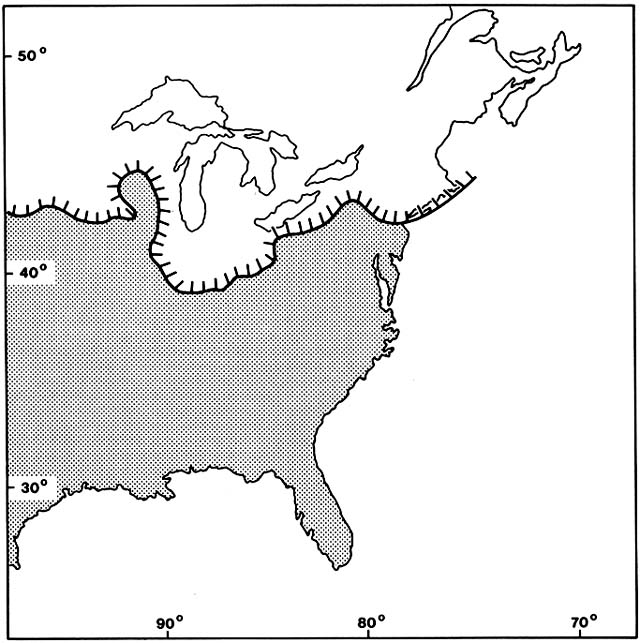
Figure 9. Areas of Eastern North America Covered by Ice During the Last Glacial. (Adapted from Davis, 1983)
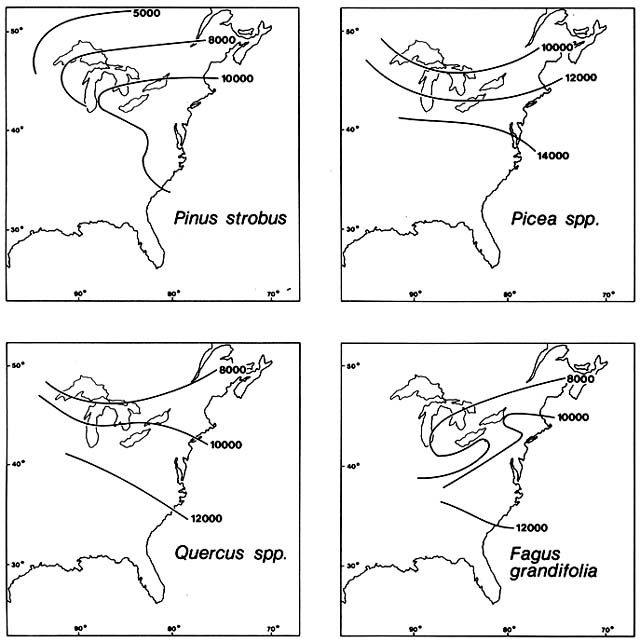
Figure 10. Postglacial Immigration of Selected Trees into Northeastern North America. Advancing fronts are
shown at specified numbers of years before the present. Subsequent losses of territory are not shown. As in Europe,
species migrated individually, not in fixed associations. (Adapted from Davis, 1983)
about 2,000 years before the deglaciated area was invaded by forest; in the Great Lakes region, the tundra phase was evidently much briefer. With the advance of the forest, Dryas and other pioneer colonists of the deglaciated territory retreated to arctic–alpine ranges, leaving isolated relics in the forest on scree and stream gravels.
The conifers that comprise the modern boreal forest migrated individually during the Late Glacial. Generally, spruce was the first to invade tundra on deglaciated territory, with Picea glauca usually ahead of P. mariana and P. rubens. Picea moved northward rapidly west of the Appalachians, reaching the Canadian prairie provinces, Lake Michigan, and Lake Erie by about 13,000 B.P. At the same time, Picea moved upward in elevation in the Appalachians, where the treeless tundra barrier to cross-mountain migration was broken about 12,500 B.P. Having crossed the mountains, Picea moved rapidly northeastward through New York and southern New England, reaching New Hampshire by 11,000 B.P. (fig. 10). Larix laricina , the tamarack, moved northward into the Great Lakes region close behind spruce; tamarack then looped northeastward around the northern flank of the Appalachians. Meanwhile, in the southeast, Pinus banksiana and Abies , probably A. balsamea , moved northward east of the Appalachians, reaching Chesapeake Bay by about 14,000 B.P. , southern New England by about 12,000 B.P. , and northern New England by about 10,000 B.P. By then, they had also branched northwestward through New York into the Great Lakes region, where Pinus banksiana populations from east and west of the Appalachians may have joined.
By the end of the Late Glacial, all these boreal conifers had fairly straight northern borders running west to east from Lake Superior to the St. Lawrence. By then, they had extensively overlapping ranges. Along with boreal hardwoods, Populus balsamifera, P. tremuloides, Betula papyrifera, and Alnus rugosa , they were in some areas forming associations like the modern boreal forest. As they advanced northward, the boreal trees began losing territory in the south.
Two nonboreal conifers, white pine, Pinus strobus , and hemlock, Tsuga canadensis , were moving northeastward and northwestward from glacial refuges in the southern Appalachian foothills or coastal plain. Before the end of the Late Glacial, both were in New England and the southern Great Lakes region.
Full Glacial refuges of many temperate deciduous hardwoods probably lay closer to the Gulf of Mexico. By 13,000 B.P. , various species of Quercus, Ulmus, Acer, Carya, Fraxinus, and Ostrya or Carpinus or both were moving rapidly northward along both sides of the Appalachians. By 10,000 B.P. , most of them were in the southern Great Lakes region and southern New England. Carya moved faster in the west, where it had reached nearly its modern limits by the end of the Late Glacial, while it had only reached the
Carolinas in the east. Fagus evidently remained east of the Appalachians and moved more slowly; by 13,000 B.P. it had reached Georgia, and by 10,000 B.P. it was in the Chesapeake Bay region. Castanea had not yet put in its appearance.
Deglaciated territory was colonized very rapidly by freshwater aquatic plants, probably due to dispersal by migratory waterfowl. Fossils of various species of Myriophyllum, Nuphar, Nymphaea, Potamogeton, Sagittaria, and Typha are known from Full Glacial time from a wide area of the southeastern United States. Fossils of most are also known from Full Glacial time from unglaciated regions in the southwestern United States. Fossils of all these taxa with 14 C dates between 15,000 and 10,000 B.P. are known from deglaciated territory between the Rockies and New England.
Holocene Epoch
By the beginning of the Holocene, about 10,000 B.P. , spruce was losing ground in the south faster than its northward advance. The spruce belt was being compressed into a narrow band close to the retreating ice and the glacial lakes; isolated mountain outposts were left behind at progressively higher elevations. By 8,000 B.P. the retreating southern and lower mountain borders stabilized near their present positions, while the northern advance accelerated, with the rapid final collapse of the ice sheet at about 7,000 B.P. In some arctic regions, the early Holocene advance of spruce carried it well beyond the present tree line. Postglacial fluctuations of the arctic tree line, the tundra—taiga border, have been dramatic in both Alaska and Canada; in places it has moved over 100 km north and south of the present tree line. Data on these displacements are very spotty in space and time; they do not appear to have been synchronous in different regions. There is also evidence of complex Postglacial fluctuations of the alpine timberline on mountains in New England. The other boreal forest trees generally lagged behind spruce in both advancing and retreating. Like spruce, they lost ground in the south to the general advance of temperate hardwoods, but held onto some relic patches in bogs, sand barrens, and burns and along streambanks and lakeshores.
After 10,000 B.P. , the nonboreal conifers, Pinus strobus and Tsuga canadensis , continued their northwestward advance through the Great Lakes region. By 7,000 B.P. , white pine was entering eastern Minnesota; by 5,000 B.P. it had advanced beyond its present limits in Ontario. Hemlock followed more slowly, reaching eastern Wisconsin about 5,000 B.P. By then, both species grew 350 m higher in the mountains of New England than now. At about 4,800 B.P. , hemlock pollen fell abruptly to about 10% of its former abundance all over eastern North America in an area of about 10 million km2 . After the
hemlock fall, there was an increase in pollen of birch and other successional species, indicating that many hemlock trees had died, not just ceased producing cones. After about 1,000 years of low abundance, hemlock began increasing and regained its approximate former abundance by 3,000 B.P.
During early Postglacial time, the temperate hardwoods continued their advances at varying rates. In eastern Canada, present northern borders of Quercus were approached by 8,000 B.P. , of Ulmus and Acer saccharum by about 6,000 B.P. , and of Carya and Fagus by about 4,000 B.P. While advancing northeastward through New England into eastern Canada, Fagus also advanced laterally westward, finally entering eastern Wisconsin after 4,000 B.P. Chestnut, Castanea dentata , appeared in the fossil record in the southern Appalachians by 8,000 B.P. and spread northward very slowly, reaching Pennsylvania by about 5,000 B.P. and southern New England by about 2,000 B.P. Its role in what is generally regarded as the regional forest primeval was thus brief.
In the Midwest and Canadian prairie provinces, some of the territory abandoned by the boreal forest was not wrested away by hardwood forest, but was instead taken over by a mosaic of prairie, oak savanna, and open woodland. The pattern of the mosaic may have been related to topography and fire frequency. The details are obscure because wind-pollinated prairie grasses cannot be separately identified and because most other prairie herbs are insect pollinated and rarely recorded as fossils. Much of the fossil evidence consists merely of changes in the proportion of tree and herb pollen. It seems, however, that prairie and savanna expanded rapidly in the region after 10,000 B.P. By 9,000 B.P. , the prairie—forest ecotone may have occupied about the same region as it did at the time of white settlement. In Minnesota, however, there was evidently a temporary eastward advance of the prairie after 9,000 B.P. for about 100 km. This advance ended about 5,000 B.P. , followed by an increase in savanna, with Quercus macrocarpa , and woodland. It is not clear how much of this middle Holocene change in Minnesota vegetation was due to changes in gross species ranges and how much was simply a change in local abundance.
Problems of Interpretation
As in Europe, pioneer North American palynologists generally assumed that changing Postglacial vegetation was controlled by changing climate. Davis (1965) pointed out the ironic contrast between the types of explanations traditionally sought for paleodistributions and for modern species distributions. Paleoecologists tend to invoke control by unknown past climates, whereas few investigators have even attempted to establish relationships between present species distributions and known climatic gradients. Present
distributions have commonly been explained by invoking so-called historical factors, that is, residual effects of unknown past distributions. In North America as in Europe, it is now generally recognized that Postglacial plant migrations did not necessarily keep up with climatic change, but may have been temporarily shaped by successional and migrational lags.
Edaphic conditions in recently deglaciated terrain obviously would have acted as a selective brake on colonizing species. In Chapter 1, it was noted that on historically deglaciated terrain, Picea colonization was delayed for decades until edaphic conditions on the raw moraines had changed, partly due to nitrogen-fixing pioneers, including Dryas and Alnus . A relay floristics succession cannot, however, be invoked as a general explanation of the sequence of species migration in Late Glacial and later time. For example, the millennia that elapsed between deglaciation of New England and forest invasion are far too long. Also, Davis (1983) pointed out that Alnus generally followed rather than preceded Picea . She also pointed out that sequence of arrival of species varied greatly within the region, often as a function of distance from refugia rather than in a regular successional sequence. She showed that 12 dominant forest tree genera advanced across the region at rates varying from a low of 100 m/year for Castanea to 400 m/year for Pinus ; in all 12, the average advance was at least several kilometers per generation. Moreover, the rivers in this region, unlike those in northern Europe, do not generally run north; thus long-range flotation could not have been generally important. Amazingly rapid advances were accomplished by species with light wind-dispersed seeds and by species with heavy animal-dispersed seeds. Howe and Smallwood (1982) noted that Picea , with seed averaging 0.002 g in weight, migrated at rates averaging 250 m/year, while Quercus , with seed 500 to 1,500 times heavier, advanced an average of 350 m/year. The former was probably mainly wind dispersed and the latter mainly bird dispersed.
Thus, in North America as in Europe, climate may have changed so abruptly that a period of free migration ensued. Until the advancing species eventually bumped into their relocated Holocene climatic limits, location of their moving borders may have been controlled by how long it took for each new generation to start producing seed and how far the seed was dispersed.
The migrations that can most reasonably be interpreted as under climatic control are later Holocene ones after borders had been stabilized for a while and then advanced again or retreated, for example, the fluctuations in arctic and alpine timberlines and in the prairie—forest ecotone that were discussed above. It is not yet clear, however, how widespread or local these fluctuations were. Many paleoecologists believe that North America had a climate that was warmer and drier than now during some part of the Holocene. This has been named the Xerothermic, Altithermal, or Hypsithermal period. This is problematic because dates suggested for the beginning and end of the period disagree by millennia, even within the part of the continent under consideration
here. For example, it was dated by Delcourt (1980) at 12,500–5,000 B.P. on the Gulf coast and at 8,500–4,500 B.P. on the Cumberland Plateau of Tennessee, by Watts (1979) at 10,000–6,000 B.P. in Appalachia, by Davis (1983) at 9,000–500 B.P. in northeastern North America, by King (1981) at 8,300–5,000 B.P. in Illinois, by Swain and Winkler (1983) at 5,500–2,500 B.P. Wisconsin, and by Wright (1976) at 8,000–4,000 B.P. in Minnesota. Wright (1976) and other workers have concluded that the warm dry period was "time transgressive" in different regions. If so, there is no reason to assume that either the causes or effects of whatever climatic fluctuations occurred in eastern North America during the Holocene were similar in different parts of the region. Placing such presumably heterogeneous phenomena under a single label can only cause confusion.
Davis (1965) noted that some late Holocene vegetation changes that had been attributed to climatic cooling were actually anthropogenic. For example, she found that in New England, changes in tree pollen that had been considered prehistoric and used as evidence for the end of the Xerothermic interval actually dated from the time of European colonization. The changes in abundance of tree species may have resulted from agricultural clearing, old field succession, and actual conifer planting. This reinterpretation was bolstered by the discovery of pollen of European weeds, Plantago major and P. lanceolata , in the same strata.
Davis (1965) also made a plea for greater attention to possible effects of prehistoric Indian activities on vegetation. Compared to Europe, very little has been done in North America to correlate palynology and archaeology. The American story is quite different from the Eruopean one because of the absence of pastoralism and the later development of agriculture. The Early Woodland Indians of the period 3,000 to 2,000 B.P. grew a little maize and a few other domesticated plants, but had a basically hunting and gathering economy. Only after about 1,000 B.P. was maize a major crop. Because Indian shifting cultivation was limited in time and localized in space within the region, it may be possible some day to evaluate its impact on forests of the region by correlating changes in archaeologic and palynologic data. Evaluating the impact of the more ubiquitous hunting and gathering people may be impossible. Their arrival in the region evidently coincided with the climatic revolution of the Late Glacial. It is generally assumed, perhaps correctly, that Indian burning had profound vegetational effects, particularly on the prairie—woodland ecotone, but there may be no way today to discriminate between the effects of Indian- and lightning-set fires in a vegetation mosaic that no longer exists.
Davis (1981b ) suggested a natural biotic rather than an anthropogenic or climatic cause for the dramatic hemlock decline at 4,800 B.P. , namely, outbreak of a pathogen or insect parasite specific to Tsuga .
Southwestern United States
(Baker 1983; Spaulding 1983; Spaulding et al. 1983; Thompson and Mead 1982; Van Devender and Spaulding 1979; Van Devender et al. 1984; Wells 1977, 1983; Wells and Hunziker 1976; Wells and Woodcock 1985)
The region considered here extends from the central Great Basin in Utah and Nevada through the Mojave and Sonora deserts of California and Arizona to the Chihuahuan Desert of New Mexico and Texas; this region is locally known as the Southwest. Rising above the present deserts are many plateaus and mountain ranges with scrub woodlands, conifer forests, and a few alpine tundras. The rugged topography, steep environmental gradients, and complex flora make fossil pollen records hard to decipher. Pines, junipers, oaks, and other dominant genera each have multiple ecologically diverse species with indistinguishable pollen. The pollen rain is an inseparable mixture from different altitudinal zones. In some vegetation types, imported pollen may actually be deposited more abundantly than local pollen. Moreover, pollen is preserved in relatively few sites.
Fortunately, the poor pollen record is supplemented by abundant macro-fossils from caves and rock shelters, mainly deposited by so-called pack rats or wood rats of the genus Neotoma . These animals gather all sorts of plant materials to build middens around their nests. Supposedly, they forage non-selectively and only within a radius of about 100 m from their nests. Hundreds of Neotoma middens in the Southwest contain fossil plant materials that have been 14 C dated to the Wisconsinan Glacial or the Holocene Epoch. The fossils are commonly identifiable to species. The middens present some problems in sorting out deposits made at different times. As in other kinds of fossil records, there are occasional disagreements between experts as to dating.
Full Glacial
During the Full Glacial, from about 21,000 to 12,500 B.P. , the Southwest had only scattered mountain glaciers. Presumably, alpine tundras and Artemisia steppe extended well below present timberline.
In the Great Basin, pluvial lakes, some of enormous size, set a regional base level at about 1,500 m elevation. Conifer forests grew down to this level over huge areas now dominated by pinyon—juniper scrub woodland and desert shrubs. Bristlecone pine, Pinus longaeva , now a rare subalpine species, grew as much as 600 m below its present lower limits; its range was continuous over the higher, eastern part of the Great Basin, becoming discontinuous
westward and southward. Limber pine, P. flexilis , and boreal juniper, Juniperus communis , both extended down more than 1,000 m below their present lower limits and may have had continuous ranges from the Rockies to the Sierras. Also present were a few deciduous shrubs, for example, Acer glabrum and Rosa woodsii , that are still associated with mountain conifer forests. In southeastern Nevada at the southern margin of the Great Basin and slightly below its general base level, Douglas fir, Pseudotsuga menziesii , joined Pinus flexilis as a dominant. Also present there were Rocky Mountain juniper, J. scopulorum , and a cactus, Opuntia polyacantha . Nowhere in the Great Basin, however, was there Full Glacial evidence of the species that dominate the modern pinyon—juniper woodlands, Pinus monophylla, P. edulis, and J. osteosperma, nor of the modern desert shrub communities.
The Mojave Desert and the adjacent Grand Canyon region had lower base levels and fewer pluvial lakes. The forest conifers that blanketed the Great Basin were confined to highland areas. The subalpine species, including Pinus longaeva and P. flexilis , grew above 1,800 m elevation. The montane species, including Pseudotsuga menziesii, Abies concolor, and Picea spp., grew down to about 1,500 m. At lower levels, the conifers did not form continuous forests, but were interspersed with pinyon—juniper woodland and high desert shrub species, including Pinus monophylla ; Juniperus osteosperma ; shadscale, Atriplex confertifolia ; desert almond, Prunus fasciculata ; bitter brush, Purshia tridentata ; Apache plume, Fallugia paradoxa ; curl-leaf mahogany, Cercocarpus ledifolius ; and big sagebrush, Artemisia tridentata . The lower limits of these species extended well below their present limits, but some had upper elevational limits as high as today. Shadscale actually extended to higher elevations than today.
Sites in lower parts of the Mojave, between 1,500 and 500 m, were almost all dominated by junipers, usually J. osteosperma , and accompanied in places by Pinus monophylla and various shrubs still found in pinyon—juniper woodlands today but at higher elevations, including joint fir, Ephedra viridis ; blackbrush, Coleogyne ramosissima ; antelope brush, Purshia glandulosa ; little leaf mahogany; Cercocarpus intricatus ; ash, Fraxinus anomala ; and desert sage, Salvia dorrii . Mixed with these were a few species that still grow at lower levels of the Mojave, such as wingscale, Atriplex canescens, and thornbush, Lycium andersonii . Some edaphically dry sites were nearly treeless; for example, on a rocky, calcareous, south-facing slope at Point of Rocks, Nevada, a 15,000-year-old midden had fossils of only one tree species, the Joshua tree, Yucca brevifolia . The dominants were shrubs, shadscale, and snowberry, Symphoricarpos longiflorus . The most likely region in the Mojave to have had a treeless desert scrub during the Full Glacial is probably Death Valley, with a floor below sea level and in an intense rain shadow. A Full Glacial midden dated at 20,000 B.P. from 400 m elevation on the flank of the valley in a zone now having only extremely sparse scrub of creosote bush, Larrea divaricata , and bursage, Franseria dumosa , showed Juniperus osteosperma and Yucca
brevifolia present but not abundant. Thus, junipers and Joshua trees grew more than 1,000 m below their present lower limits. The dominant species at the site was evidently the shrubby Yucca whipplei , accompanied by shadscale and Opuntia basilaris . It seems likely that the lower parts of Death Valley were completely treeless desert. Nowhere in the Mojave, however, is there Full Glacial fossil evidence of the creosote bush—bursage or other modern desert scrub communities. If creosote bush, now the most common dominant over huge areas of the Mojave, was present there during the Full Glacial, it must have been extremely rare.
The known Sonoran Desert fossil record for the Full Glacial is very sparse. Neotoma middens have been found at a few localities in the lower Colorado River basin of California and Arizona at elevations between 250 and 1,000 m. These generally show dominance by Juniperus osteosperma and Pinus monophylla , far below their present lower limits. Common associates were all species that are still common members of pinyon—juniper woodlands now restricted to higher elevations and moister climates: Ephedra viridus, E. nevadensis, Yucca brevifolia, Y. whipplei, Nolina bigelovii, Atriplex confertifolia, Acacia greggii, Cercocarpus intricatus, Arctostaphylos pungens, Rhus tribolata, Artemisia tridentata , and Haplopappus cuneatus and scrub oaks, including Quercus chrysolepis, Q. dunnii, Q. turbinella . As in the Mojave, the northern Sonoran Full Glacial vegetation evidently lacked the common dominants of the modern desert scrub. Whether they were present anywhere in the huge Sonoran Desert south of the U.S.—Mexican border is not known.
In New Mexico and Texas, the region now occupied by Chihuahuan Desert scrub at elevations below 1,500 m had a Full Glacial vegetation of pygmy conifer woodland. Midden deposits consistently show dominance by junipers, usually J. scorpulorum , and by pinyons, Pinus edulis and P. remota . The first two species grew at lower elevations and farther south than now; P. remota grew much farther west than now. Associated with the pinyons and junipers at lower elevations were scrub oak, other shrubs, and succulents, such as Agave, Yucca , and Opuntia spp. At higher elevations in the mountains of New Mexico and Texas, pinyons and junipers were associated with montane and even subalpine conifers. For example, middens dated 13,000 to 15,000 B.P. at elevations between 1,600 and 1,700 m in the Sacramento and San Andres mountains of southwestern New Mexico showed the presence of Pseudotsuga menziesii in a pinyon—juniper woodland. A San Andres midden also yielded a unique Late Glacial record of Pinus ponderosa . In the nearby Guadalupe Mountains of western Texas, middens dated at 13,000 B.P. at elevations of about 2,000 m showed that Pinus edulis and Juniperus scopulorum grew together with not only Pseudotsuga but also with subalpine spruces, Picea engelmannii and P. pungens , and a white pine, Pinus strobiformis .
Thus, during the Full Glacial the Southwest from timberline to base level was evidently dominated by conifers that are now restricted to disjunct highlands. These formed forests at high elevations and woodlands below.
There is no record of extensive ponderosa pine parkland, sagebrush steppe, or treeless desert scrub. Some species now present in those communities are known from Full Glacial fossils as subordinate members of conifer woodlands and also in local microhabitats. Other species that are today among the most widespread and abundant in deserts of the Southwest are completely missing from the Full Glacial record, including columnar cacti, Cereus spp.; ocotillos, Fouquieria spp.; bursage, Franseria dumosa ; and paloverdes, Cercidium and Parkinsonia spp.
Late Glacial
Fragmentary evidence suggests that plant migrations signaling the end of the Full Glacial in the Southwest began between 12,500 and 10,000 B.P. , a time span arbitrarily called the Late Glacial.
In the eastern Great Basin, sequences of midden deposits at different elevations in the Confusion Range show upward displacement of the lower border of the subalpine Pinus longaeva . At 12,000 B.P. it still extended down to the lowest sites at about 1,700 m elevation; by 10,300 B.P. it was gone even from the highest sites at 2,160 m. Its upward retreat was closely followed by advance of Pseudotsuga menziesii and Juniperus scopulorum . During the Full Glacial, their nearest approach to the Confusion Range had been at Rainbow Canyon, 160 km to the south. By 12,000 B.P. Pseudotsuga had arrived at the lowest sites in the Confusion Range, but Juniperus scopulorum was not yet present there. By 10,300 B.P. , these two species dominated the range up to the summit.
In the present Mojave Desert, major plant migrations evidently began about the same time. At Rampart Cave, at 500 m elevation in the Grand Canyon, where there had been pinyon—juniper woodland around 12,000 B.P. , there were only desert shrubs by 10,000 B.P. At Point of Rocks, at 900 m elevation in Nevada, the site mentioned above as having had Joshua trees in the Late Glacial had become much more similar to the present by 11,700 B.P. By then it had only desert shrubs, including desert almond, Prunus fasiculata ; snakeweed, Gutierrezia microcephala ; and Opuntia spp. being dominant. In Death Valley at 400 m, where a Full Glacial midden had shown juniper and Joshua trees present, a 10,000-year-old midden showed only bursage, Franseria dumosa . Higher up on the flanks of Death Valley at 800 m, where Utah juniper had been dominant in the Full Glacial, only shrubs were present by 11,000 B.P. These included Yucca whipplei ; antelope brush, Purshia glandulosa ; rubberbrush, Chrysothamnus teretifolius ; and Opuntia basilaris . The Mojave was evidently first invaded in Late Glacial by a scrub oak, Quercus turbinella , which had been present in the Sonoran Desert during Full Glacial. The first Mojave macrofossils of this species, dated 12,000 B.P. , were found 60 km west of Searles Lake, California. In the same region, a
rise of oak pollen before 11,000 B.P. may mark the advance of this now common Mojave oak.
In the northern Sonoran Desert, middens indicate that Pinus monophylla began its northward and upward shift between 13,000 and 10,000 B.P. , leaving behind an impoverished juniper woodland. The first midden record of a desert community without woodland species is from the lower Colorado River valley near Yuma, Arizona. Dated at 10,500 B.P. , the middens contained creosote bush, Larrea divarieata , and bursage, Franseria dumosa , which still grow in the area.
In the present northern Chihuahuan Desert of New Mexico and Texas, middens dated between 10,500 and 10,000 B.P. at elevations of 1600 to 1700 m in the Sacramento Mountains showed change from the Full Glacial vegetation. Pinyon and juniper persisted but Pseudotsuga menziesii was gone, replaced by desert shrubs, including zorillo, Choisya dumosa , and honey mesquite, Prosopis glandulosa . In the nearby Guadalupe Mountains at 2,000 m elevation, where there had been a complex Full Glacial mixture of pinyon, juniper, and forest conifers, all the conifers except juniper were gone by 11,500 B.P. , replaced by grassland. Pollen profiles from the Llano Estacado, adjacent to the Chihuahuan desert on the northeast, show a change between 14,000 and 10,000 B.P. from pine and spruce dominance to an association transitional to the present grassland and desert scrub.
Holocene
After the beginning of the Holocene, at about 10,000 B.P. , lower elevations in the Great Basin were abandoned by the subalpine conifers, such as Pinus longaeva, P. flexilis , and Juniperus communis , and were temporarily occupied by spreading montane conifer species, especially Pseudotsuga menziesii, Abies concolor , and Pinus ponderosa . The available midden record is so spotty in time and space that the migrations can be bracketed only loosely and at a few places. For example, in the Snake Range in eastern Nevada at elevations between 2,000 and 2,700 m, these three montane conifers were absent at 9,200 B.P. but present by 4,600 B.P. Pseudotsuga and Abies are known to have been present during glacial time 200 and 280 km south of the Snake Range, respectively. Where ponderosa pine came from is a great mystery. Its only known glacial record in the Southwest is from New Mexico. The first available Holocene record is also from New Mexico, 700 km east of the Snake Range, at Chaco Canyon, from about 5,500 B.P.
Wells (1983) noted that there is no evidence these montane conifers, unlike the subalpine species, ever had continuous ranges across the Great Basin (fig. 11) and that their migration rates during early Holocene time were orders of magnitude too fast to have been accomplished by short-range wind dispersal of their heavy seeds. He noted that common birds of the
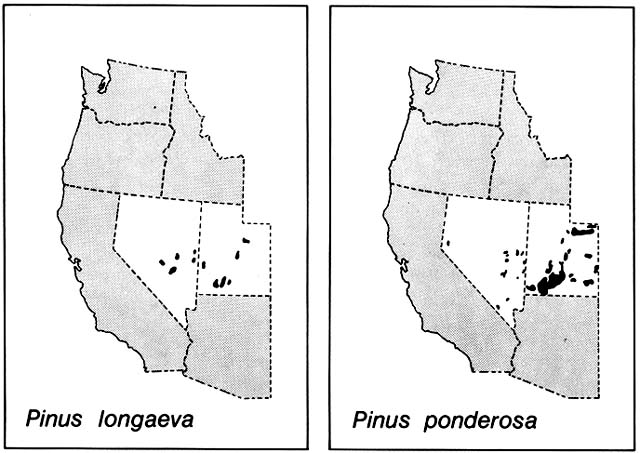
Figure 11. Partial Present Ranges of Pinus longaeva and P. ponderosa in the Great Basin. Bristlecone grows up to
timberline and Ponderosa at slightly lower elevations, but both are confined to high mountains that rise like forested
islands above a sea of desert scrub. These strikingly similar modern patterns of the two species conceal radically
different migrational histories. During the Wisconsin Glacial period, bristlecone blanketed lower elevations in the region
down to the great pluvial lakes, its present disjunctions are the result of upward retreat when the postglacial arid climate
developed. During the glacial period, ponderosa was evidently absent from the entire region and it did not immigrate until
the lowlands were too dry for it. Its disjunctions probably result from long-range bird dispersal between mountain
forests. (Adapted from Critchfield and Little, 1966)
region, especially jays, nutcrackers, and crossbills, are excellent long-range dispersal agents, not only of conifers but also of oaks and montane shrubs. Thus, the present disjunct, islandlike montane ranges of many plant species in the Great Basin probably are not the result of fragmentation of continuous early Holocene ranges but rather were achieved by long-range colonization. This colonization was not completed until after pinyon and juniper, also dispersed long distances by birds, had occupied intermediate elevations and after lowlands of the Great Basin had been taken over by desert scrub dominated by Artemisia and Atriplex .
By 10,000 B.P. in the Mojave Desert, the disjunct patches of subalpine conifers had begun their upward withdrawal, leading to extinction on most mountain ranges. They were being replaced at elevations of up to 2,400 m by Juniperus scopulorum and other montane conifers. The montane conifers, which had extended down to about 1,500 m elevation, were in turn being replaced by J. osteosperma, Pinus monophylla , and other members of the modern pinyon-juniper woodlands, which had previously occupied the lower levels of the region. By about 9,500 B.P. , these woodlands were being invaded by a scrub oak, Quercus dunnii , which was present in the northern Sonoran Desert during glacial time. By 7,500 B.P. the lower levels of the Mojave Desert were being taken over by desert scrub, including Larrea divaricata , similar to modern associations.
Early Holocene records in the Sonoran Desert are sparse. At some places, juniper woodland was withdrawing from levels below 1,000 m and being replaced by Larrea -dominated desert scrub by 7,500 B.P.
In the present Chihuahuan Desert of Texas, sparse evidence suggests gradual decrease in woodland species and increase in desert grassland species until about 4,000 B.P. , after which Larrea divaricata, Fouquieria splendens, Mammillaria spp., and other desert scrub species arrived.
Problems of Interpretation
Macrofossils preserved in pack rat middens have provided much more accurate information on the Southwest's prehistoric plant geography than was available from the region's sparse and almost indecipherable pollen record. The midden record, however, has its own interpretational problems. Each individual fossil assemblage gives highly accurate and complete information on plant species present, but only for a very tiny area and a short time span, leaving huge gaps to be filled by interpolation. This is problematic because location and preservation of middens were not necessarily random in relation to vegetation patterns. Some bias must be expected simply because preservation of ancient middens depended on shelter by rocks from rain and fire. The possibility that unrecorded plant species were present in the region
is taken more seriously by some experts than others. For example, Spaulding et al. (1983) suggest that many species that are still missing in the midden record were probably present since pre-Wisconsinan times on both sides of the Continental Divide. By contrast, Wells (1983) tends to take the absence from the record (at least of species that are presently common) at face value; he prefers to postulate that they immigrated from the south after Wisconsinan times.
Another limitation of the midden record is that the rats deposited material irregularly and episodically. Unlike pollen deposits, which under ideal conditions may accumulate continuously in neat strata through long time spans, midden deposits are commonly interrupted and disturbed, especially by rodent tunneling. This problem is well recognized by all investigators, but opinions differ as to the best methods of dissecting a midden and dating its components.
Thus, there is room for conflicting interpretations of the midden macrofossil record, as shown by the case of Larrea , the creosote bush, which dominates huge areas of all three North American deserts. It is agreed that the North American Larrea population, sometimes called L. tridentata , was ultimately derived from a species native to temperate South America and that its intercontinental dispersal was probably due to migratory birds. The North American population has differentiated into separate races, with the Chihuahuan Desert race being diploid, like the South American progenitor. The other two races are polyploid, 4N in the Sonoran Desert and 6N in the Mojave. The simplest hypothesis would be that the 4N and 6N races evolved in sequence as the species expanded westward from a founding colony. However, Larrea does not appear in the available macrofossil record of the northern Chihuahuan Desert until about 3,300 B.P. , whereas it was definitely present in the Sonoran Desert of southwestern Arizona by about 10,500 B.P. and in the Mojave Desert of California by about 7,500 B.P. There are reports of even earlier Larrea fossils from Sonoran and Mojave middens, but the dates are questionable. Unfortunately, the Neotoma fossil record has not been investigated south of the Mexican border.
Using a different approach, Vasek (1980) estimated the age of the largest known Larrea clone in the California Mojave Desert as nearly 12,000 years. This clone has a radius of nearly 8 m around a central bare area from which the old dead wood has rotted away. The age was extrapolated from modern growth rates. Vasek suggested that growth rates during the early Holocene may have been greater and that the clone's age might be reasonably estimated as about 9,500 years.
On the basis of current evidence, Wells and Hunziker (1976) proposed that dispersal of Larrea into North America occurred in the Late Glacial, with rapid northward and westward migration accompanied by differentiation of the polyploid races. Based on the same evidence, others (Spaulding
et al. 1983; Van Devender et al. 1984) proposed that Larrea arrived and expanded westward across the Continental Divide during an earlier Pleistocene interglacial period.
Whatever uncertainties remain, the Neotoma macrofossil record has provided solid evidence of major, rapid plant migrations in the Southwest since Late Glacial time. Some of these migrations could not have been even roughly reconstructed by postulating displacement en masse of modern vegetation.
In the Southwest, as in other regions discussed previously, Late Glacial and early Holocene plant migrations were generally so rapid that it seems gratuitous to postulate gradually changing climatic controls. The problem is explaining how species borders could shift so fast, not what was restraining their movement. The evident lack of climatic brakes on early Holocene plant migrations does not preclude possible later Holocene climatically controlled vegetation changes. The classic Altithermal model postulated that during middle Holocene, climate in the Great Basin was warmer and drier than now; there is some supporting evidence of this (Byrne et al. 1979). In the Southwest, however, as in other regions discussed previously, whatever late Holocene climatic fluctuations occurred were evidently not synchronous or equivalent in different parts of the region (Baker 1983), and their effects on plant distributions are unclear.
Some late Holocene vegetational changes have been attributed to human impact. For example, on the Colorado Plateau in northwestern New Mexico, from about 5,500 to 1,250 B.P. , Neotoma middens show an apparently stable altitudinal gradient from desert scrub at lower elevations up through pinyon and juniper to ponderosa pine. After 1,250 B.P. , middens no longer contained pinyon or ponderosa pine and contained little juniper, although all three are present in the region now. Archaeologists have postulated that the Anasazi people, who occupied Chaco Canyon and other parts of the region from before 1,000 to 800 B.P. , used approximately 100,000 ponderosa pines to construct their multistoried pueblos and other buildings and also used huge quantities of pinyon and juniper for fuel.
Northern South America
(Bradbury et al. 1981; Flenley 1979; Hammen 1974; Leyden 1985; Salgado-Labouriau 1982)
In the Colombian Andes, pollen profiles dated between 30,000 and 14,000 B.P. suggest that during the Full Glacial, borders of vegetation zones were about 1,000 m below their present levels. Thus, species belonging to the alpine paramo, the subalpine heathlike scrub, and the montane forest must
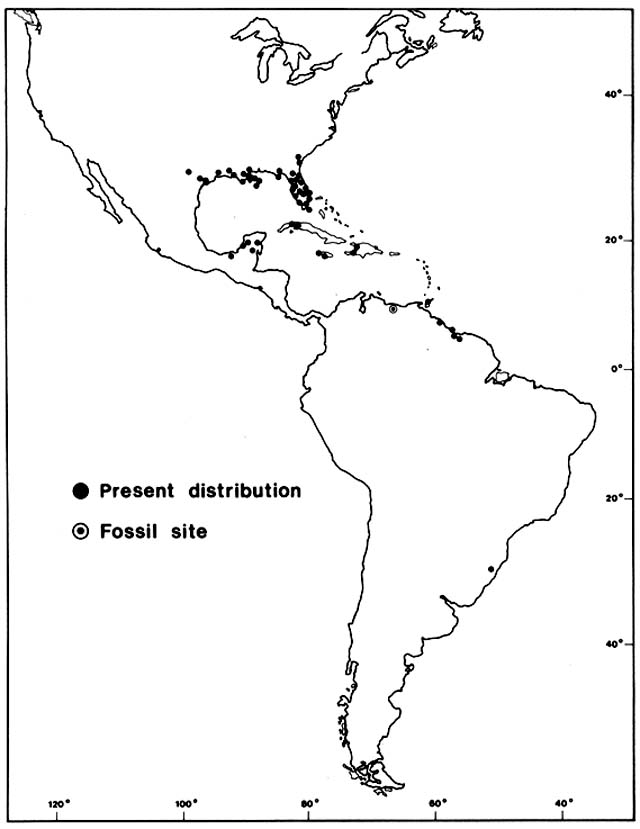
Figure 12. Present Distribution of Amaranthus australis . Without historical evidence, it would be debatable
whether such a disjunct distribution was ancient or recent and whether it was due to natural or human dispersal.
In this case the pattern is probably old and natural. Fossil evidence from Lago de Valencia in Venezuela suggests
that the population of this species now growing around that lake was established about 8,300 years ago. In
southeastern United States seeds of this species are eaten in large quantities by migratory waterfowl about to take
off for the tropics, and most of the West Indian and Latin American colonies grow along major flyways. All the
close relatives of this species are North American natives. It is doubtful, however, that A. australis could have
survived there during glacial periods. Perhaps birds reintroduced it to its homeland from tropical colonies.
(Adapted from Sauer, 1972)
have had ranges that were slightly broader than now but not continuous between major mountain ranges. In other words, ranges of highland species, including Podocarpus, Alnus, and Quercus, may have always been disjunct in the region. In the adjacent lowlands, savanna grassland and open woodland, with Byrsonima crassifolia and Curatella americana as dominants, were probably more continuous than at present. There is no evidence that lowland tropical forests, now extensive in the region, were present during the Full Glacial. Between 14,000 and 10,000 B.P. , pollen profiles suggest a rapid upward displacement of vegetation borders to approximately modern elevations, although individual cores within the region are not closely synchronous.
In Venezuela, a 40-m-deep lake, Lago de Valencia, lies at an elevation of about 400 m. Present natural vegetation of the surrounding region is a mosaic of savanna, tropical deciduous forest, and wet evergreen forest, depending on topography. Late Glacial profiles dated between 13,000 and 10,500 B.P. show that instead of a lake the basin at that time contained saline marshes dominated by Cyperaceae and Poaceae with some Asteraceae , including Ambrosia , and Amaranthaceae , especially Alternanthera . The pollen also suggests that during the Late Glacial upland vegetation surrounding the marshes was predominantly savanna. Rare tree pollen of Bursera and Acalypha indicates that there was some open deciduous woodland in the watershed but no forest. This does not support a hypothesis advanced by several biogeographers who attempted to deduce prehistoric migrations of taxa from present distributions. They proposed that the Lago de Valencia region was a Pleistocene refugium for various tropical forest plants and animals. Pollen profiles dated 10,500 to 9,800 B.P. from Lago de Valencia show that the Pleistocene saline marshes and savannas were replaced in the early Holocene by a shallow, brackish lake with widely fluctuating margins. This lake supported both aquatic and littoral halophytes, including Ruppia , chenopods or amaranths, and Ambrosia . Low but consistent input of tree pollen, mainly Bursera, Acalypha, and Moraceae, indicates an open woodland in the watershed but not near the lake.
Between 9,800 and 8,300 B.P. , the lake filled, overflowed, and freshened, with a change in aquatics and a sharp decline of littoral halophytes. At the same time, there was a sharp rise in tree pollen, especially Bursera, Spondias, Trema, Brosimum, and other Moraceae, indicating advance toward the lake of early successional tree species, presumably on thin, undeveloped soils. At about 8,300 B.P. , there was a sudden rise in a pollen type that may document arrival of Amaranthus australis, a species now abundant in marshes along the lake shore (fig. 12). This tall herb is native to the Everglades in Florida and to other marshes of the subtropical and tropical Gulf of Mexico region. It also has a widely disjunct distribution in marshes of the West Indies and South America, presumably due to long-range dispersal by migratory waterfowl,
as discussed above in Chapter 1. Pollen profiles from Lago de Valencia indicate that since 8,300 B.P. , the region around the lake has had a mosaic of savanna, woodland, and forest with moderate fluctuations in abundance of different taxa, but basically much like the modern vegetation.
Pollen cores on the coasts of Guyana and Surinam document drowning of some savannas during early Holocene time by eustatic rise of sea level. Grasses were replaced by mangroves, first Avicennia , followed by a long period of dominance by Rhizorphora , indicating deeper water. Later, Avicennia reappeared, perhaps due to sediment deposition after sea level rise tapered off.
Reconstruction of Late Glacial and Holocene plant migrations in northern South American is still in an embryonic state. Available fossil pollen records, especially those with adequate dating, are few and far between in a region with formidable vegetational diversity. Little is known about the pollen signatures of present vegetation types of the region. Translating fossil pollen spectra into vegetation types will be especially hard for tropical forests with predominantly insect pollination. Also, detecting prehistoric human influences on plant distributions may be extremely difficult. Most late Holocene pollen diagrams from northern South America show some indications of forest clearance, and some have maize pollen in their upper levels, usually since about 3,000 B.P. However, archaeological evidence indicates that agriculture had begun in the region long before that, presumably on such a local scale that the effect on the pollen rain was very subtle. Most tropical crops are poorly recorded in fossil pollen records, and the pollen of many milpa weeds and second-growth trees is indistinguishable from that of natural riparian pioneer vegetation.
Japan
(Tsukada 1982a,b,c, 1983a,b,c, 1985)
During the last Full Glacial, from about 25,000 to 15,000 B.P. , lowering of sea level made the Sea of Japan a lake, and the present Japanese islands formed an interconnected arc with land bridges to mainland China via Korea and to Siberia via Sakhalin. Arctic tundra extended southward to northern Hokkaido, and there were mountain glaciers and patches of alpine tundra down to central Honshu. The tundra graded through park woodland with pines and tree birches into boreal conifer forest, which covered most of northern Honshu and the highland areas to the southwest. Temperate conifers covered most of southwestern Japan and graded into a mixed forest of temperate conifers and deciduous hardwoods in southern coastal areas. In these forests, the species associations and dominance were completely different
than in the modern mixed conifer—hardwood forests. Perhaps members of the modern broadleaf evergreen laurel—oak forest survived in the extreme south, but fossil evidence for this has not yet been found.
After about 15,000 B.P. , in Late Glacial and early Holocene time, the vegetation changed rapidly. The borders of physiognomic formations moved roughly 10° northward in latitude at a given elevation and 1,400 m upward in elevation at a given latitude. By 10,000 B.P. , rising sea level had separated the islands and brought moist, marine climate to the west and east coasts.
Interpretation of the pollen record is difficult because most important tree genera are represented in Japan by multiple species. Tsukada (1982b , 1983a , 1985) has been able to discriminate between some congeners and, with the help of macrofossil evidence, has proposed a tentative reconstruction of tree migrations, only a few of which will be sketched here as examples.
The deciduous beech is represented in Japan by two species, Fagus crenata and F. japonica . The two are difficult to distinguish palynologically and have widely overlapping modern ranges; they will be treated here as a unit. Fagus evidently survived the Full Glacial in sparsely scattered populations along the coasts of Honshu south of 37°N latitude. About 12,000 B.P. , Fagus began invading inland mixed conifer—hardwood forests and expanded rapidly northward. By 9,000 B.P. , Fagus was moving into far northern Honshu, by 8,000 B.P. into southern Hokkaido, and by 7,000 B.P. was approaching its present northern limit. Meanwhile, in the south, Fagus had retreated from the lowlands of Kyushu and Shikoku during Late Glacial time and from the lowlands of southern Honshu in early Holocene time, when the deciduous hardwoods were replaced by evergreen broadleaf hardwoods.
Cryptomeria japonica , closely related to Sequoia and Taxodium , had Late Glacial refugia on both coasts of Honshu centering around 35°N latitude and on the south coast of Shikoku in lowland areas now partly covered by the sea. About 12,000 B.P. , Cryptomeria began migrating inland and upward. It also began expanding in both directions along the Sea of Japan coast of Honshu. Moving southwestward, it reached the southwest tip of Honshu by 7,000 B.P. ; moving northward, it reached its present natural limit on the west coast by about 4,000 B.P. Its northward expansion on the Pacific side of Honshu was slower, not being completed until about 1,000 B.P.
Tsukada (1983c ) has suggested that human activities played an intriguing and important role in the ancient vegetational history of Japan. When large game animals, including mammoth, bison, horse, giant elk, and boar, became extinct there in the Late Glacial, the Paleolithic people turned increasingly to plant foods. At that time, the advancing deciduous broadleaf forests supplied an abundance of beechnuts, acorns, chestnuts, walnuts, and hazelnuts. Human transport of these seeds may have accelerated forest migration. In the early Holocene, archaeological sites continued to be concentrated in deciduous forest regions with abundant nut supplies. There is evidence of a
primitive buckwheat agriculture as early as 6,600 B.P. in southwestern Honshu. In the Late Jomon period, about 4,000 to 3,000 B.P. , slash and burn agriculture spread in southwestern Japan, first for buckwheat and soon after for rice cultivation. As forest clearance intensified and spread into northeastern Japan, it was followed by spread of weed plants. Prominent among these was Japanese red pine, Pinus densiflora , which spread explosively in old fields starting about 2,000 B.P. in southwestern Japan and approached its present limit in northern Honshu about 700 B.P.
Cryptomeria was much exploited by prehistoric humans, the decay-resistant wood being easily split by simple, primitive tools. Since before 2,000 B.P. , Cryptomeria boards and posts were much used to dike flooded rice fields. Drastic reduction in Cryptomeria pollen followed its middle Holocene maximum. After about 1,000 B.P. , Cryptomeria again increased markedly, presumably due to protection and reforestation. Deliberate prehistoric planting is believed to be responsible for the arrival of Cryptomeria on Kyushu about 2,000 B.P. and on Tsushima Island, between Kyushu and Korea, about 1,500 B.P. Planting of Cryptomeria on Hokkaido began much later.
East Africa
(Butzer 1971; Flenley 1979; HSamilton 1974, 1982; Livingstone 1967, 1971, 1975)
Sharp climatic gradients on the towering East African volcanoes cause a whole spectrum of vegetation types, from alpine tundra through heath and montane forest to lowland forest, to be telescoped into a short horizontal distance. The region has no deposits of local macrofossils like the Neotoma middens of southwestern North America and therefore must rely on palynology for fossil evidence. As in other mountain regions, palynology faces problems in sorting out local from transported pollen, but careful work has revealed some major vegetation changes since glacial times.
During the Full Glacial, snowlines were about 1,000 to 1,200 m lower than today, producing glaciers on Mount Kenya, Ruwenzori, Kilimanjaro, and Elgon.
On Ruwenzori, valley glaciers flowed down below the snowline to about 3,000 m elevation until about 1,500 B.P. The moraines that they abandoned formed Lake Mahoma, which is now surrounded by montane forest. Fossil pollen and grass phytoliths from the lake indicate that until about 13,000 B.P. it was above timberline and close to the border between alpine tundra and heath; present nearby were some of the famous Afro-alpine endemic plants, including the giant Lobelia and Dendrosenecio . Soon after 13,000 B.P. ,
rising timberline evidently passed the lake with arrival of various montane forest species. However, the presently important montane forest conifer, Podocarpus , did not arrive until about 3,000 B.P. About 1,000 B.P. , there was a strong increase in grass and weedy dicot pollen that may have signaled agricultural clearing in distant lower elevation areas.
On Mount Kenya, borders between vegetation zones were about 1,000 m below their present levels from about 26,000 to 14,000 B.P. A pollen profile from a site at 3,100 m elevation, now surrounded by heath shrub, shows that it was surrounded by alpine tundra grassland until about 10,500 B.P. when the rising grassland—heath border passed that level. At the same time, heath vegetation was replaced by forest at a lower site, at 2,400 m elevation. The first tree species to arrive, Hagenia abyssinica , appeared about 10,500 B.P. and was soon followed by some other montane forest trees, but Podocarpus did not arrive until about 5,000 B.P. Hagenia remains dominant today at the heath—forest border, about 500 m above the site.
In the Cherangani Hills, near Mount Elgon, fossil pollen from a 2,900 m elevation site indicates an alpine grassland with Dendrosenecio from 28,000 to 14,000 B.P. , when heath vegetation took over. After about 10,000 B.P. , Olea and other dry montane forest trees arrived; Podocarpus arrived about 9,000 B.P. The present vegetation near the site is mainly savanna, which may have been formed by clearing of the dry forest.
The pollen profiles mentioned above are all from highlands and had alpine tundra or heath vegetation during the Full Glacial. There are no Full Glacial sites that recorded presence of forest, so locations of Pleistocene refugia of montane trees are unknown. They evidently did not descend low enough to spread out widely or have continuous migration routes between the mountain ranges. The few pollen records from lower elevations indicate that the great East African plains had Full Glacial vegetation much like the present. A pollen record from west of Lake Malawi at 1,400 m elevation indicates the presence since 20,000 B.P. of open grassy woodland similar to the present. A large number of species persisted from Late Glacial through Holocene time with virtually no change in the roster until signs of Iron Age agriculture appeared. Another pollen profile from Lake Victoria at 1,100 m elevation indicates a regional vegetation of grassy savanna until about 13,500 B.P. , when Olea, Trema , and other dry forest trees arrived. Much later, a decrease in forest and an increase in grass may date from agricultural clearing.
Thus, there was a fairly coherent pattern of abrupt upward displacement of borders between alpine and subalpine vegetation, starting about 14,500 B.P. , followed by more prolonged migrations of Podocarpus and other montane trees. Livingstone (1975) noted that Holocene migration rates and sequences of arrival in the East African highlands were clearly not limited by changing climate, but may have been controlled by distance from refugia and gradual soil development, especially on moraines.
7
Pleistocene Before
Last Glacial
The fossil record becomes more and more fragmented with increasing time depth. Also, time before the Last Glacial is beyond the reach of 14 C dating. Eventually it may be possible to establish a solid global chronology based on other kinds of dating, including seafloor magnetic reversals, such as the Jaramillo and Olduvai events. The terrestrial fossil record, however, has not yet been tied to marine chronology, and stratigraphic correlations between local series remain questionable. Furthermore, identification of fragmentary fossils with living taxa, particularly at the species level, becomes increasingly tentative with time depth. Nevertheless, the fossil record does give some insight into Pleistocene plant migrations; although the view is dim, it is real and not imagined.
Northwestern Europe
(Butzer 1971, 1974; Frenzel 1968; Pennington 1969; Szafer 1966; Turner 1975; West 1970)
The vegetation history of successive cold and warm phases (Table 2) was in some respects cyclic and repetitious. In Britain, for example, the forest invasions at the beginning of each warm phase or interglacial for which there is a good record followed a sequence roughly similar to that of the Holocene: pioneer herbs, shrubs, Betula , and Pinus preceded the mixed hardwood forest of Quercus, Ulmus, Fraxinus, Corylus , and the later arriving Carpinus and
sometimes other tree genera. At the close of each warm phase, the mixed hardwoods were replaced by pines, birches, and readvancing heaths and bogs before the spread of steppe and tundra in the next cold phase.
Within this gross cycle, species migrations showed many striking differences between successive phases, partly due to regional extinctions during cold phases. For example, Sequoia and Tsuga returned to the region for the last time during the Cromerian, Carya and Pterocarya for the last time in the Holstein Interglacial. The last record of Rhododendron ponticum in Ireland, before its modern reintroduction (discussed in Chapter 3), was also in the Holstein. By then, plant extinctions were nearly over and the flora was nearly identical to the Holocene flora. Animal extinctions continued longer. Forest elephants, hippopotami, rhinoceroses, water buffalo, and monkeys were still present in northwestern Europe in the Eem Interglacial.
The sequence of immigration of members of the mixed hardwood forest was not constant in different warm phases. For example, of three common associates in Britain, Corylus arrived first in the Pastonian Interglacial, Quercus in the Hoxnian, and Ulmus in the Ipswichian. On the continent, the mixed hardwood forest species rapidly replaced conifers during the Cromerian and Eem interglacials, but during the intervening Holstein Interglacial, the hardwoods remained a minor and patchy component of a persistent, monotonous conifer forest dominated by Picea and Abies . Also, the ultimate borders of some species varied greatly between successive warm periods. For example, macrofossils show that during the Holstein, the Eem, or both, Fagus sylvatica, Tilia platyphylla, Vitis sylvestris , and Buxus sempervirens had borders far north of their Holocene ones in both the British Isles and on the continent. Macrofossils of Hedera helix and Ilex aquifolium , however, showed that their Holstein and Eem borders remained south of their Holocene ones.
Szafer (1966), Frenzel (1968), and other workers have long recognized that plant associations throughout the Pleistocene were kaleidoscopic, not constant blocks of species. This was true not only during migrations following shifts in climate, but also of so-called climax vegetation that had equilibriated during the middle of warm and cold phases. This was probably partly due to climatic differences between successive cycles, but it was probably largely due to vagaries of migration. Reinvasions of forest species during successive warm phases had to come from distant southern refugia. Butzer (1985) noted that full glacial climates in the Mediterranean region were so harsh that refuges for temperate forest species were not islands of familiar vegetation but strange associations, probably stunted and sparse in growth, that were sporadically distributed in areas of broken topography on sunny slopes with shelter from the wind and favorable moisture conditions. The sparse fossil record in the Mediterranean obscures the locations of refugia, but it is likely that they varied greatly between successive cold phases and between species. Each readvance of a species during a warm phase may have followed
|
lowed a unique pathway. Rates of advance must have varied between species depending on time to maturity, radius of seed dispersal, and competitive and successional interactions with other species.
A less obvious point made by Frenzel (1968), Turner (1975), and other workers is that retreat of tree species late in the interglacial phases may not have been due solely to climatic deterioration. It is exceedingly difficult to disentangle soil acidification, podsolization, and expansion of healthland due to climatic change from soil changes mediated by vegetation. West (1970) suggested that hardwoods persisted longer in Britain during the Ipswichian than in other interglacials because the failure of Picea and Abies to immigrate in the Ipswichian may have reduced podsolization then.
Advances and retreats of cold-tolerant species were probably also individual rather than latitudinal shifts of blocks of species. Pollen evidence from glacial phase loess deposits is sparse, but what is present does not suggest a flora like the Holocene arctic-alpine tundras of Europe. Glacial floras of the region were perhaps more like those of the present high, cold, arid steppes of interior basins of Eurasia. Bell (1969) noted that glacial fossils, both in Britain and on the continent, included halophytes, such as Glaux maritima and Suaeda maritima, along with steppe species and did not include the whole steppe flora. Retreats of cold-tolerant species during interglacials were probably much less catastrophic than those of warmth-requiring species during glacials. Steppe and tundra plants were driven back not by warmth but by competing vegetation. They may have mostly retained a wide presence in northern Europe during interglacials on steep rocky slopes, along seacoasts, lakeshores, and streams, without remaining in the fossil record.
Extinct Homo erectus and forms transitional to H. sapiens were present in Europe since at least Elster time. During the Elster and Saale glacials, these Paleolithic people evidently subsisted largely by hunting big herbivores of open grasslands. During the interglacials, they evidently adapted to open woodland habitats. It is generally assumed that Paleolithic peoples lacked the population size and technology to have much effect on the flora and fauna. They may, however, have aided in the amazingly rapid migrations of hazel, beech, and other nut-bearing trees. Also, there is evidence from a Paleolithic site in Suffolk, Great Britain, of forest burning during the Holstein Interglacial. An associated pollen profile shows a sudden decline in tree pollen and a rise in pioneer weedy plants. Some plants now considered anthropophilic weeds, such as Rumex acetosella, Polygonum aviculare, and Stellaria media, were recorded as fossils in northern Europe by Cromerian time, that is before archaeological evidence of the arrival of Homo . They evidently were native pioneers preadapted to join in the migrations of early humans as ruderal camp followers.
Southern California
(Axelrod 1966, 1978, 1983a )
Macrofossils of the Soboba flora are believed to date from about 1 million years ago, contemporaneous with the Nebraskan Glacial. They are from a low elevation site on the western flank of the San Jacinto Mountains, where the present vegetation is sage scrub, transitional between chaparral and semidesert scrub. Most of the Soboba fossil flora is represented by species that survive in the region but that now grow fully 1,000 m higher in montane mixed conifer and chaparral: Abies concolor, Libocedrus decurrens, Pinus lambertiana, P. ponderosa, P. coulteri, Pseudotsuga macrocarpa, Populus tremuloides, several species of Ceanothus, Rhamnus crocea, Prunus ilicifolia, Rhus ovata, Garrya flavescens, and Cercocarpus betuloides . Other species are extinct in the region, including Acer brachypterum, which survives in summer rain regions of Arizona, and Magnolia grandiflora, which survives in nature only in the southeastern United States but has been reintroduced to California in cultivation and thrives if given summer watering.
Fossil cones believed to be roughly contemporaneous with the Soboba flora were found near Ventura; they had evidently floated downstream to coastal lagoons and were later buried in deeper water offshore by a turbidity current. They include several closed cone pines, Pinus radiata, P. attenuata, and P. muricata/remorata ; digger pine, P. sabiniana ; and Douglas fir, Pseudotsuga menziesii . All of these survive within 200 km of the site as highly disjunct relicts, but most are now abundant only considerably farther north or in the interior.
This very limited glimpse of early Pleistocene cold phase flora suggests that some montane conifer and chaparral species had more continuous lowland ranges than they do now and that many of the modern lowland species were absent. Considering, however, that it is 1 million years old, the early Pleistocene flora of southern California is startlingly modern, particularly in comparison to mammals of the same age, which mostly belonged to genera that are now extinct.
Northern Andes
(Hammen 1979)
A lake basin at 2,600 m elevation on the Sabana de Bogotá in Colombia has yielded a continuous pollen record for the whole Pleistocene. The lake now lies in an open edaphic savanna within the general cloudforest belt about
800 m below the present alpine tree line. Fossil pollen shows repeated oscillation between floras of forest and treeless alpine paramos, which would have caused repeated isolation and coalescence of paramo islands.
More significant migrations are suggested by an increase in the roster of species over the whole Pleistocene. Of cloudforest trees, Podocarpus was already present at the beginning of the Pleistocene, Ilex and Weinmannia arrived early in the Pleistocene, Alnus along with the shrubby Gunnera in the middle, and Quercus and Drimys late. In the alpine flora, Polygonum and Plantago were present at the beginning, Miconia and ericads arrived early, and Gentiana and Ranunculus late in the Pleistocene. Some of the cloudforest species, including Podocarpus, Weinmannia, Gunnera, and Drimys, presumably arrived from the south. Alnus, Quercus, and most of the alpine paramo species are believed to have immigrated from the north.
Much tectonic uplift of the Andean Cordillera was probably accomplished before the Pleistocene, providing a highland pathway from the south. Although the Panama landbridge was also formed before the Pleistocene, it never offered a highland bridge. Highland immigrants from the north presumably arrived by long-range bird dispersal.
8
Neogene (Miocene
And Pliocene)
The Neogene Period spans over 20 million years, more than ten times the span of the whole Quaternary (Table 3). The fossil record reveals only glimpses separated by great gaps in space and time. However, there are some rich macrofossil deposits with floras that are almost all identifiable with living genera; many members appear to be closely related or identical to living species. In paleobotany, Tertiary and older fossils are usually given discrete species names in separate time levels, partly because their fragmentary nature prevents complete comparisons. Since the fossil binomials are meaningless to nonpaleobotanists, however, a common practice (which will be followed here) is to give the name of the closest living counterpart in parentheses, for example, Pinus (strobus) .
Most of the fossil floras are from freshwater sediments and are thus biased in favor of local lakeshore or stream bank plants. Also there is bias in favor of species producing large, tough leaves or other organs capable of leaving a good impression in sediment. On the basis of sediment bedding and texture, Axelrod (1958a, 1980) has in some cases been able to discriminate between fossils from local riparian vegetation and material transported from elsewhere by floods and mudflows.
Dating of Neogene floras is currently in a state of flux. Recent advances in dating marine sediments have led to drastic revision of the Neogene time scale and to a shift of the Miocene–Pliocene border to a much later date. The terrestrial and marine chronologies are not yet well integrated. Time correlations between fossil floras of different sites are often controversial except in the few cases where radiometric dates have been established.
By the beginning of the Neogene, the global map of continents and
oceans was beginning to resemble its modern pattern, but some fossil floras deposited in Neogene time have since been significantly displaced in latitude or elevation. Also, major changes in ocean temperatures, rain shadows behind mountain barriers, and coastlines make reconstruction of paleoclimates a formidable problem, one which will not be considered here.
North-Central Europe
(Axelrod 1983b ; Butzer 1971; Ferguson 1971; Frenzel 1968; Hammen et al. 1971; Mädler 1939; Szafer 1966)
North-central Europe had a wonderfully rich Neogene forest flora of both conifers and broadleaf hardwoods. Listing of genera (Table 4) understates the richness because many genera were represented by multiple species. Nearly all these genera are considered members of the Arcto-Tertiary Geoflora, which was shared among all the northern continents. Where its members originated and their directions of migration are not known. Very few of them appear to be capable of ocean crossings. A few probably had been shared between Europe and eastern North America before formation of the North Atlantic Ocean in the Paleogene. Exchanges between Europe and Asia may have occurred repeatedly during both Paleogene and Neogene.
Various floras from Poland, Germany, and the Netherlands, from which Table 4 is compiled, suggest that this rich forest flora persisted into the early Pliocene, when it was decimated during the Villafranchian, a time with predominantly heath vegetation. Reforestation occurred during the succeeding warmer Tegelen period, but few tree species returned, although the high alpine barrier to migration did not yet exist. In the Netherlands, about 80% of the species present in the rich early Pliocene Reuverian flora but only about 20% of the species in the impoverished late Pliocene Tegelen flora are now extinct in the region. The late Pliocene Ludhamian flora of Britain was dominated by genera still common there, for example, Pinus, Alnus, Betula, and Quercus ; it had only a few genera that have since become extinct in the region, for example, Tsuga and Pterocarya . Thus, the so-called modernization of the European flora by regional extinction, although not completed until the Pleistocene, was well underway during the Pliocene. Most of the extinct species or close relatives survive elsewhere; for example, North America has living counterparts of the fossil European Pinus (strobus) and Populus (balsamifera) and eastern Asia has living counterparts of fossil Acer (palmatum) and Quercus (serrata) .
Freshwater aquatic species may have suffered less Pliocene and later extinctions in north-central Europe than forest trees did. Szafer (1946) reported
|
early Pliocene fossils in Poland of 23 species of freshwater aquatic and marsh plants. One water lily, a species of Euryale, is now extinct in Europe and survives only in eastern Asia. All the other species belong to genera that survive in the region, for example, Ceratophyllum, Najas, Sagittaria, Trapa, Carex, and Polygonum ; Szafer assigned 16 of these fossils to living northern European species.
Western North America
(Axelrod 1958a,b, 1973, 1980, 1983b, 1985a, Axelrod and Raven 1985; Cross and Taggart 1982; Leopold 1969; Raven and Axelrod 1974; Thorne 1978; Wolfe 1972; Wolfe and Tanai 1980)
The rich macrofossil record of western North America has long been studied by an outstanding corps of paleobotanists. Parts of the region have fossil beds preserved in ash falls and lake basins created by volcanic and
|
tectonic activity. These may record not only stable vegetation controlled by climate and topography but also temporary successional series. The region is so vast and diverse and the fossil record so discontinuous that only a tentative and sketchy migrational story has yet emerged.
In the Miocene time, the woody flora of the region included a great majority of the genera that are important there today and many others that no longer survive there (Table 5). For broad generalization, it is useful to separate Arcto-Tertiary and Madro-Tertiary floras, although the fossil record indicates that, then as now, species ranged individually and formed kaleidoscopic rather than constant associations. Many species, however, evidently had broader and more continuous ranges then because the present great mountain ranges did not yet exist.
|
Arcto-Tertiary Flora
All but a few of the gymnosperms and deciduous angiosperms in Table 5 are classified in the Arcto-Tertiary group. These genera had spread from uncertain origins all around the northern hemisphere during Paleogene time. Few if any of them appear to have been capable of ocean crossings. Some presumably had crossed between Europe and North America before the North Atlantic Ocean barrier developed in the Paleogene. Contacts across Beringia may have continued intermittently during the Miocene. Especially close relationships between eastern Asian and western North American members of the Arcto-Tertiary forest floras have long been recognized. Recent chemotaxonomic study has shown that Miocene fossils from Oregon of several Arcto-Tertiary genera have organic compounds more similar to living eastern Asian than to eastern North American congeners (Niklas and Giannasi 1978). Different authorities, including Batchelor (1979) and Frakes (1979), have irreconcilably different concepts of eustatic sea level changes during this time.
During the early Miocene, from about 25 to 15 million years ago, a rich assortment of Arcto-Tertiary conifers and deciduous hardwoods ranged from Alaska, and perhaps a Bering land bridge, down to northern California and inland to Idaho and northern Nevada. They did not comprise a homogeneous vegetation. Lowlands were presumably dominated by broadleaved evergreen and deciduous hardwoods, many of which are no longer present in the region. Also present were some conifers, such as Glyptostrobus and Metasequoia , that are no longer present there. Higher elevations were dominated by confier genera, some of which are still present there, such as Abies, Picea, Pinus, Pseudotsuga , and Sequoia. Abies, Picea , and Pinus forests were more extensive inland in Montana and Wyoming, although the major uplift of the modern Rocky Mountains had not yet begun. A few deciduous Arcto-Tertiary genera, including Metasequoia, Carya, Pterocarya, Sassafras , and Ulmus , that have since become extinct in the region were still present locally in early Miocene time.
At the same time, in central California and west central Nevada, the Arcto-Tertiary and Madro-Tertiary genera mingled in a mosaic, with the Arcto-Tertiary flora presumably occupying the cooler north slopes and higher elevations. Pure conifer forests may have been confined to isolated high volcanic peaks. In Miocene southern California, the Arcto-Tertiary flora was evidently represented only by riparian deciduous hardwoods, such as Acer, Alnus, Fraxinus, Platanus, Populus , and Salix , all of which have close modern relatives in the region, and by one extinct species, Ulmus (americana) .
Neogene migrations of the Arcto-Tertiary flora consisted mainly of retreat to disjunct relict areas and local extinctions, which Axelrod (1985a ) has
attributed mainly to decreasing summer rainfall due to ocean cooling reinforced in places by rising topography and rainshadows. Retreats were most drastic in Alaska, where few hardwood trees were left by late Miocene time except riparian Alnus and Salix . By late Miocene time, parts of Alaska had glaciers down to sea level, and above 65°N, taiga, tundra, and heath had taken over. Although probably open, the Bering land bridge was evidently no longer a pathway for intercontinental tree migration. Beginning about 14 million years ago forests in the Pacific Northwest, from Washington and Idaho to central California and central Nevada, became impoverished as many species retreated to maritime coastal regions, rising interior mountains, and riparian sites. Of the Arcto-Tertiary genera that have become extinct in the Pacific Northwest since the early Miocene, none of the conifers and only a few of the deciduous hardwoods are known to have survived after the Pliocene. However, the fossil record is so fragmentary that relicts may have persisted without being recorded.
Madro-Tertiary Flora
The woody flora at the start of Neogene time also included many genera that evidently originated and remained in North America and Mexico, being unable to penetrate high enough latitudes to use Tertiary land connections to Eurasia around either the North Atlantic or North Pacific. This Madro-Tertiary group includes nearly all the evergreen angiosperm genera listed in Table 5, which are still important members of the modern sclerophyll woodlands and chaparral in the region. The group also includes the deciduous angiosperms that survive in interior arid areas of the region (Table 5); these are members of modern thorn scrub and riparian desert vegetation. This flora also includes a few miscellaneous angiosperm genera no longer locally present but surviving in Mexico. Some of these genera are strictly confined to tropical lowlands today, such as Cedrela , a tall, compound-leaved deciduous tree of Mesoamerica.
During early Miocene time, some Madro-Tertiary species ranged much farther north than their surviving relatives. For example, in the Pacific Northwest's present Cascade Mountain region, the Arcto-Tertiary flora commonly had an admixture of Madro-Tertiary evergreen broadleaf trees and chaparral species, including Quercus (chrysolepis), Lithocarpus (densiflora), Arbutus (arizonica), Persea (borbonia), Heteromeles (arbutifolia), Ceanothus (cuneatus), Cerocarpus (betuloides) , and the broadleaf deciduous Cedrela (odorata) . During the Pliocene in central California, some Madro—Tertiary sclerophyll species still grew north of the ranges of their present relatives, Quercus (engelmannii, tomentella), Rhus (laurina, ovata), Ceanothus (spinosus) , and Lyonothamnus (floribundus) .
|
During early Miocene time, Madro-Tertiary sclerophyll woodland and chaparral species also ranged farther east than their surviving relatives. For example, in west-central Nevada, areas now dominated by desert shrubs and sagebrush had early Miocene floras including all the species noted in the preceding paragraph except Cedrela and Quercus (tomentella) . These fossil floras also included several other sclerophyll tree and shrub genera now confined to the west side of the Sierra Nevada.
In late Miocene time, the general level of the incipient Sierra Nevada was probably still 2,000 m lower than today. The 7-million-year-old Mount Reba flora, from a site now near timberline at 2,650 m, was dominated by Madro-Tertiary sclerophyll woodland species whose modern equivalents are present at about 600 m elevation in the adjacent foothills. Evidently, development of the intense Great Basin rainshadow and spread of desert flora postdate the Miocene. There is no Pliocene fossil record from Nevada.
Most of what is now southern California lay beneath the sea through the Neogene. The few Miocene floras known from the region all evidently grew at low or medium elevations and lack the montane conifers now present in the region. Along with the few deciduous riparian trees noted above, Miocene floras included a variety of chaparral, sclerophyll woodland, and desert shrub genera, mostly with close relatives surviving in the region (Table 6). Considering how much time and tectonic change has ensued, the Miocene floras were amazingly modern; by comparison the contemporary fossil fauna
included many strange, extinct genera. Also, Miocene floras found west of the San Andreas fault actually grew in much lower latitudes. For example, the Mint Canyon flora may have been displaced 200 km northward from its original location. This might be expected to explain the presence of xerophytic fossil plants north of the present limits of Mexican relatives (Table 6), but the same xerophytic species were present in Miocene floras found east of the fault and not displaced. Two species, Lyonothamnus (floribundus) and Prunus (lyonii) that were present on the southern California mainland during the Miocene have surviving relatives only on the offshore islands. When the retreat of the xerophytic and island species began is not known. Early, middle, and late Miocene floras from the region are very similar, and there is no Pliocene record from the region.
Middle America
(Graham 1973, 1976S, 1982)
Pollen records from Mexico and Central America suggest southeastward migration during the Miocene along the Cordilleran axis of many Arcto-Tertiary tree genera: Abies, Picea, Pinus, Alnus, Fagus, Juglans, Liquidambar, Populus , and Ulmus . All of these appeared in Vera Cruz, on the Mexican Gulf coast, during the Miocene. They seem to have formed a lowland forest of mixed conifers and broadleaf deciduous trees adjacent to coastal mangroves with no sign of intervening tropical lowland vegetation. Little work has been done, however, on the pollen signatures of modern tropical lowland forests. It is conceivable that insect-pollinated trees could be present in a region and leave little trace in the pollen record. In Vera Cruz at present, the mangroves grow next to extensive rainforest; the Arcto-Tertiary genera are still present in southern Mexico but grow high in the mountains and in different associations.
The Cordilleran system was being uplifted during the Miocene, and by late Miocene time the Panama land bridge had probably formed. Fossil pollen indicates that Alnus and Juglans had reached Panama during the Miocene and entered northern South America during the Pliocene.
9
The Deep Past
The Neogene and later periods discussed above cover less than one-tenth of the time that seed plants have existed. Their fossil record, which has great gaps in space and time, began in the Devonian Period (Table 7), more than 300 million years before the Neogene. Unlike the Neogene, for which nearly all fossil plants can be identified to living genera, if not species, the of characters becomes increasingly strange with greater age. More often than not, it is highly speculative whether these strange creatures were evolutionary dead ends or primitive ancestors of much changed modern descendants. Moreover, paleontologists not only have to deal with strange creatures, they usually have only bits of creatures that nobody has seen whole. Separate taxonomies have to be constructed for pollen, leaves, stems, seeds, and other organs. Articulating them is a great accomplishment. For example, the existence of a great extinct order, the bizarre seed ferns or pteridosperms, was not revealed until it was finally concluded that fossil seeds, long named Lagenostoma, and fernlike foliage, named Lyginopteris, belonged to the same plant.
Also, research on early seed plants has necessarily been partitioned among specialists in different taxonomic groups whose first task was to work out evolutionary relationships of particular lineages. Only gradually have plant geographic syntheses emerged.
For this time span, another change in approach to migrations is necessary. There is no hope of tracing geographic changes on the species level. Even for higher taxa, information on geographic distributions is generally rudimentary. All that can be attempted here is to seek evidence of really grand migrations of higher taxa. This will be done chronologically, starting with the origins of the first seed plants. Some notice will be taken of continental movements, but for all of the dazzling light that plate tectonics has shed on
|
Table continued on next page
|
geomorphology, it is still of limited use in reconstructing plant migrations. Contact between tectonic plates is irrelevant if the continental margins were submerged. A shallow sea is as much a barrier for land plants incapable of long-range dispersal as is a deep ocean.
Paleozoic And Mesozoic Gymnosperms
(Arnold 1969; Axelrod 1974; Bambach et al. 1980; Barnard 1973; Beck 1976; Brenner 1976; Chaloner and Lacey 1973; Chaloner and Meyen 1973; Douglas and Williams 1982; Florin 1963; Hallam 1977; Hughes 1976; Mamay 1976; Miller 1977; Penny 1969; Phillips et al. 1985; Plumstead 1973; Rabinowitz et al. 1983; Retallack and Dilcher 1981a ; Roche 1974; Stewart 1983; Taylor 1981)
Origin of Gymnosperms
About 3 billion years passed between the appearance in the fossil record of the first plants with chlorophyll, the cyanobacteria or blue-green algae, and the appearance of the first vascular land plants, the Silurian pteridophytes.
These non-seed-bearing pteridophytes diversified during the Devonian into several major lineages, commonly interpreted as having surviving members in the whiskferns, horsetails, clubmosses, and ferns. All these evolved complex vascular diploid bodies, which in some cases were large trees. Once established, a diploid pteridophyte is well fitted for survival on land. Also, the microscopic haploid spores, which pteridophytes produce in huge quantities, are well adapted to long-range wind dispersal. After the spores land on the ground and germinate, however, the ensuing haploid stages, including a swimming sperm, are not much better fitted for land life than their algal ancestors.
Evolution by the gymnosperms of pollen and seeds eliminated the weak links in the pteridophyte life cycle for sexual reproduction on land. The evolutionary steps were complex, but the net result was simply sheltering and nurturing the vulnerable stages within the body tissues of the mature vascular diploid. Gymnosperm cones are organs of the diploid plant and are supplied with water and food by its vascular tissues. The only stage of the sexual reproductive cycle that has to exist out in the world on its own is the haploid pollen. The target of a pollen grain, unlike a fern spore, is not the ground but an opening in the integument surrounding the young seed on a female cone. There it can deliver the sperm to the egg without external water.
Origin of the gymnosperms came remarkably soon after the origin of their pteridophyte ancestors. An intermediate group, the progymnosperms, appeared in the Devonian in what is now North America and Europe. The geography of the Paleozoic world is still very speculative, but reconstructions for the Devonian and early Carboniferous commonly show North America, Greenland, and Europe united as a single continent, called Euramerica, which was located in low latitudes and separated by oceans from proto-Asia and Gondwanaland. The oceans had evidently not barred pteridophyte migrations; from the Silurian to the Carboniferous, pteridophyte floras had fairly uniform global distributions. The seeds of early gymnosperms, however, like those of most modern ones, were evidently poorly equipped for long-range wind or water dispersal. Although gymnosperm pollen grains are capable of long-range wind dispersal, this serves only to maintain gene flow between already established disjunct populations of a species, not to found a new population.
So far as is known at present, the first gymnosperms were confined to Euramerica. The first named seed plant species, Archaeosperma arnoldii , appeared there in Late Devonian time. Its seeds were borne in pairs on open, cuplike structures arranged more loosely than in the tightly condensed cones of later gymnosperms, and there was a larger opening in the integument for entry of the pollen than in later gymnosperms.
Carboniferous
The Carboniferous is named for the remains of the great swamp forests laid down as coal. In both Europe and North America, the abundant fossil record shows that these swamps were dominated by pteridophyte trees, with gymnosperms as subordinate associates. The nature of the upland flora, if any, is not known.
The gymnosperms of the Early Carboniferous (or Mississippian) already represented several families within the extinct order of Pteridospermales or seed ferns. Two other gymnosperm orders, presumably also derived from the progymnosperms, namely the Cordaitales and Voltziales, became widespread in both midcontinental North America and western Europe in the late Carboniferous (Pennsylvanian). Both were structurally closer to modern conifers than to ferns.
Some paleogeographic reconstructions show Pangea beginning to be assembled in the Late Carboniferous, with proto-Asia and Gondwanaland approaching or contacting Euramerica. This would account for reports of Pennsylvanian age gymnosperms of all three orders in parts of Asia and Gondwanaland, although dating and identity of these fossils is not very clear. Even if Pangea was assembled, there were great barriers to seed plant migration during the Pennsylvanian because of extensive marine transgressions and, in Gondwanaland, extensive glaciation.
Permian
During Permian time, Pangea was complete, with broad contacts near the equator between Euramerica and western Gondwanaland. A single world ocean extended from pole to pole and spanned 300° of longitude at the equator. The interior of the continent must have had great deserts, particularly behind upthrust mountains; shallow inland seas created other barriers to seed plant migration. In spite of contacts between continental plates, the Permian had regional floristic differences.
The three Carboniferous gymnosperm orders, Pteridospermales, Cordaitales, and Voltziales, survived in the Permian in Euramerica and were joined by probable primitive representatives of two living gymnosperm orders, Cycadales and Ginkgoales. During the Permian, all five lineages appeared in Asia and Gondwanaland, where they were mainly represented by different genera than in Euramerica. The Cordaitales became extinct late in the Permian.
The most famous Gondwana gymnosperm genus is Glossopteris , which
can be classified either as a member of the Pteridospermales or in an order of its own. Glossopteris was a tree with trunks as large as 40 cm in diameter and with narrow, tongue-shaped leaves. It dominated coastal swamps that accumulated to form much of the commercial coal of the southern hemisphere, uplands being occupied by other gymnosperms. In the Permian, Glossopteris spread over huge areas of present South America, Africa, India, Antarctica, and Australia that had been glaciated during the Carboniferous. The paleolatitudes within which Glossopteris grew ranged from about 40°S to almost the Permian south pole (fig. 13). It is not known whether the trees were evergreen or deciduous, but in any case, it is hard to account for their survival in nearly six months without sun.
Triassic and Jurassic
The Mesozoic began with Pangea fully assembled and with low sea levels. During Triassic time, the Tethys Sea formed between the single northern continent, Laurasia, and Gondwanaland, but it remained narrow in places; there may have been intermittent contacts between the northern and southern continents during much of the Mesozoic. By the Middle Jurassic, sea level had risen and shallow continental seas separated North America—Greenland from Europe and South America from Africa, at least intermittently. Also during the Jurassic, India-Madagascar may have already broken away from Africa.
Glossopteris survived through the Triassic but died out early in the Jurassic, ending one pteridosperm lineage. Two other now extinct orders possibly derived from the pteridosperms, namely the Caytoniales and the Bennettitales, arose during the Triassic. The Caytoniales had a fleshy tissue partly enclosing the seed that suggests animal dispersal. One genus of Caytoniales, Lepidopteris , had a remarkable range across Laurasia and Gondwanaland in paleolatitudes from 60°N to 60°S. The Bennettitales, which superficially resemble cycads, had spectacular reproductive structures that suggest adaptation for animal pollination.
The two Paleozoic gymnosperm orders that survive today, Cycadales and Ginkgoales, spread widely through both Laurasia and Gondwanaland in the Mesozoic, increasing greatly in abundance and diversity. Some Jurassic Ginkgo leaves are indistinguishable from those of the living G. biloba, and some of the cycads were already similar to living genera.
Two other living orders of gymnosperms, Coniferales and Taxales, arose in the Mesozoic not long before the Voltziales, their probable progenitors, became extinct. Five important modern conifer families had evidently already diverged by the Early Jurassic: Pinaceae in Laurasia, Podocarpaceae in Gondwanaland, and Araucariaceae, Cupressaceae, and Taxodiaceae on both
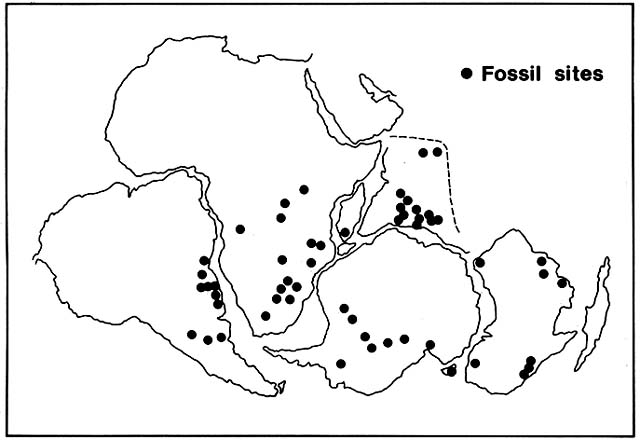
Figure 13. Late Paleozoic Range of Glossopteris , a Dominant Seedfern on the Supercontinent of Gondwanaland.
Gondwanaland broke up into several continents, outlined by dashed lines. Fossils of Glossopteris were then
displaced to widely separated parts of the globe. Since the genus evidently was not adapted for transoceanic
dispersal, its fossil distribution appears inexplicable on an earth with fixed continents. This was noted as evidence
for the hypothesis of continental drift long before the modern theory of seafloor spreading was developed.
continents. During the Jurassic, the Taxales, precursors of the living yew, entered the fossil record in Laurasia.
Cretaceous
The Tethys Sea continued to be a partial barrier between the northern and southern continents during Cretaceous time, but there were intermittent contacts between Europe and Africa and between North and South America. During the Early Cretaceous, the incipient Indian and South Atlantic oceans began to dissect Gondwanaland, but separation was incomplete except for isolation of India–Madagascar during most of the Cretaceous. By the Santonian, Africa was an island, but South America may still have been linked via Antarctica with Australia and New Zealand. By the Maestrichtian, the South Atlantic had widened to about 800 km between Brazil and Africa, but there had been little seafloor spreading in the North Atlantic. North America, Greenland, and Europe were still in contact north of 50°N latitude. At higher latitudes, Alaska and Asia had been in contact since the Coniacian. Laurasia, however, was divided during much of the Cretaceous by marine transgressions. Although the extensive Jurassic epicontinental seas had receded at the start of the Cretaceous, the regression was temporary. Seaways extending from the Tethys Sea to the Arctic Ocean bisected both North America and Eurasia from mid-Cretaceous until they withdrew in the Maestrichtian.
Two Triassic gymnosperm orders; the Caytoniales and Bennettitales, remained widespread in the Early Cretaceous but were extinct by the end of the Cretaceous.
Cycadales and Ginkgoales continued to be widespread throughout the Cretaceous, ranging to amazingly high latitudes in both northern and southern continents. The Taxales remained confined to Laurasia.
Among the Coniferales, the Pinaceae were represented by fossils confined to Laurasia; extant genera represented included Pinus and possibly Cedrus and Larix . The Podocarpaceae continued to be widespread in parts of former Gondwanaland, excluding Africa; fossils are commonly identified with living genera, especially Dacrydium and Podocarpus . The Araucariaceae, Cupressaceae, and Taxodiaceae continued their Jurassic pattern of wide ranges in both northern and southern continents. Jefferson (1982) described a puzzling fossil forest of conifers resembling Araucaria that lived during the Early Cretaceous in Antarctica at a paleolatitude of about 70°S. The trees were buried by volcaniclastic deposits and remained standing and rooted. The trees must have had problems of shading under the low sun angle, but they were closely spaced and had an understory of pteridophyte vegetation. Also, their well-defined growth rings show that annual growth was at a rate that would be very high for modern conifers, even at low latitudes.
Among the Taxodiaceae, fossils tentatively assigned to Sequoiadendron , which survives only in California, were deposited in eastern North America and Greenland in Early Cretaceous time (Florin 1963). By Late Cretaceous, this taxon ranged to Europe and western North America. Metasequoia , which survives only in China, had a coherent Cretaceous range from eastern Asia through Alaska to western North America. Sequoia , now confined to western North America, evidently originated there, perhaps by hybridization between Sequoiadendron and Metasequoia . Various other genera of Taxodiaceae had close Cretaceous precursors. Cryptomeria, Cunninghamia, Glyptostrobus , and Sciadopitys , which survive only in eastern Asia, had Cretaceous precursors in widely scattered sites in Eurasia, North America, and Greenland. Arthrotaxis , which survives only in Tasmania, had Cretaceous precursors in New Zealand and South America.
Cretaceous Angiosperms
(Axelrod and Raven 1978; Batten 1981; Beck 1976; Brenner 1976; Dilcher and Crane 1984, 1985; Doyle 1973, 1977; Doyle and Hickey 1976; Hickey 1977; Hughes 1976; Muller 1970, 1981; Retallack and Dilcher 1981a,b ; Srivastava 1981; Tralau 1973; Tschudy and Scott 1969; Walker and Doyle 1975; Walker et al. 1983)
The origin of the angiosperms has long been one of the most baffling problems in paleobotany. Various fossil gymnosperm orders and all living ones except the conifers have been suggested as possible progenitors, but missing links in the fossil record make all the alternatives highly speculative. Until recently it was generally supposed that the angiosperms originated someplace where there was no fossil record and underwent major phylogenetic diversification, including initiation of many living families, before entering the fossil record. Major theories of angiosperm phylogeny have been built almost entirely by deductive comparisons between living groups, with any relevant fossil evidence considered to be simply missing.
Recently, paleobotanists have launched a new attack on the problem of Cretaceous angiosperm evolution, and hopes are currently high that the fossil record is much more complete than had been realized. As noted by Wolfe (1973), the amount of missing record may have been greatly exaggerated by a practice by pioneer paleobotanists of seeking resemblances between Cretaceous leaf fossils and living genera and assigning the fossil to whatever modern family offered the closest resemblance. This practice has worked well for Neogene and Pleistocene woody species that have undergone very little subsequent evolution or extinction. In these relatively young fossil floras,
identifications based on leaves, other macrofossils, and pollen have all fit together nicely. Identifications of Cretaceous leaves with modern taxa, however, have not generally been supported by other fossils, and resemblances may be merely superficial.
Instead of leaping to identify primitive angiosperm fossils with familiar names, efforts are now being made to work forward from the beginning of the record in early Cretaceous, to build an independent taxonomy of initially strange pollen and macrofossils, which are not expected to have already become stabilized in their present forms. Pollen is being given special attention because it provides a more abundant and complete sampling of the flora from a catchment area larger than that of the macrofossils. By comparative study of enough floras worked up in this way, it may eventually become possible to trace angiosperm evolution and migration through the Cretaceous and to follow some lineages to extinction and others to recognizable modern descendants. Only glimpses of such a story are now possible.
Neocomian
The earliest generally accepted fossil evidence of an angiosperm comes from beds deposited over 100 million years ago in Siberia; the fossil consists of small, pinnately veined, simple leaves, presumably from a dicot. How far this plant had proceeded in transition from gymnosperm to angiosperm reproductive structures is unknown.
Barremian
A sparse macrofossil record of apparent monocot and dicot leaves and of dicot fruits and a more abundant pollen record suggesting both monocots and dicots are reported from both sides of the Tethys Sea in North America, Europe, Siberia, South America, and Africa. The angiosperm fossils were deposited in sediments indicating that they inhabited shores of lagoons, estuaries, and other sites disturbed by coastal erosion and deposition. Retallack and Dilcher (1981b ) suggested that the angiosperms originated as coastal pioneers capable of rapid migration by wind and water dispersal. The ocean may have served more as a highway than as a barrier to migration, as is true of later seashore angiosperms, which typically have seeds or fruits dispersed by ocean currents. Because of the pervasive symbioses between angiosperms and animal pollinators and seed dispersers, it has been widely assumed that the very origin of angiospermy involved coevolution with animals, for example, that the ovary served initially to protect the young ovules from chewing insects visiting the flowers. If the angiosperms really arose as coastal pioneers,
perhaps the ovary served initially for sea dispersal and coevolution with animals was secondary.
Barremian pollen attributed to angiosperms include a probable monocot form, Liliacidites , and a probable dicot form, Clavatipollenites . Both resemble gymnosperm pollen in being monosulcate, that is, having a single germination furrow, but differ from gymnosperm pollen in having intricately sculptured instead of smooth coats. This sculpturing may have adapted the pollen grain to adhere to the body of an insect or to the stigma of a flower. The latter would mean that the plants had crossed the crucial line defining angiospermy, that is, the ovules were no longer exposed to direct pollination but had to be reached by growth of a long pollen tube penetrating the ovary from a stigma. Among living angiosperms similar primitive monosulcate pollen is produced by many monocots and by one of the six subclasses of dicots, namely, the Magnoliidae, which include Magnoliaceae, Lauraceae, Nymphaeaceae, and related families.
Aptian
Monosulcate angiosperm pollen continued to be widespread in both the northern and southern hemispheres. In Gondwanaland, it was joined by the first tricolpate pollen, that is, grains with three germination furrows, almost certain proof of angiospermy. In the moist pollen chamber of a gymnosperm ovule, a single germination locus suffices, but on the exposed stigma of an angiosperm flower, multiple pores on different sides of the grain are highly advantageous. Aptian tricolpate pollen has been found in Israel in sediments deposited at low latitudes on the Gondwanaland coast and also in sediments deposited along embayments in the rift valley system lying between what is now equatorial Africa and South America. Tricolpate pollen and forms with similar names derived from it by elaboration and modification are characteristic of all the six subclasses of dicots except the Magnoliidae.
Albian
During the Albian, tricolpate and derivative pollen types diversified vigorously, not only in Gondwanaland but also north of the Tethys Sea in both western and eastern North America and in Europe. Later in the Albian, angiosperms evidently spread to higher latitudes than ever before in both Laurasia and Gondwanaland; this expansion is indicated by both monosulcate and tricolpate pollen and by macrofossils. In North America and Europe, dicot leaves became much more diverse; in addition to forms that suggest Magnoliidae, leaves appeared that may represent other dicot subclasses, such
as Hamamelidae, exemplified by Platanus . Actual fossilized flowers are known from the Albian of North America and Europe; they indicate divergent adaptations for insect and wind pollination. Some are bisexual, with large, showy petals and many ovules, closely resembling Magnolia . Some are bisexual and have small, delicate petals, probable nectaries, and few ovules, closely resembling Pyrus , the apple. Some are unisexual, tiny, inconspicuous, and grouped in inflorescences strikingly similar to Platanus .
Generally angiosperms were still subordinate to gymnosperms and ferns except in coastal and riparian sites. In North America, angiosperms penetrated from the Gulf Coast to Alberta along the shores of Albian epicontinental seas and also first appeared in Alaska along shifting seashores. In river deposits, there was some segregation of fossils in different kinds of alluvium, ferns and gymnosperms being found in fine sediments and angiosperms being relatively abundant in coarser sediments deposited by high-energy streams. The leaves attributed to Platanus were commonly buried in sand.
Cenomanian
Patterns changed only slowly. Until late Cenomanian time, angiosperm pollen generally remained rare compared to gymnosperm pollen, especially in Gondwanaland. Retallack and Dilcher (1981b ) concluded that angiosperms were still mainly confined to coastal and riparian habitats, growing as mangroves along tidal distributaries, in swamps along lagoon margins, as scrub on natural levees, and as floating aquatics. Some of the richest Cenomanian fossil beds are in the Dakota Formation of Kansas, laid down in a variety of coastal and deltaic environments. Angiosperm leaf fossils there and elsewhere in North America and Europe show increasing diversity. Many have been questionably identified with living families.
Tricolpate dicot pollen continued to be widespread and appeared for the first time in high arctic latitudes. A derivative type, Normapolles , first appeared in the Cenomanian in eastern North America and Europe. It has been interpreted as signaling a reversion to wind pollination, perhaps by Fagaceae. Wind pollination implies large stands of a species, not just scattered seashore or streambank pioneers.
Turonian
Eastern North America and Europe were still in contact at high latitudes in Turonian time. They retained pollen floras that were broadly similar but already had distinct genera. In both regions Normapolles diversified rapidly. There, for the first time, angiosperm pollen was abundant relative to gymnosperm
pollen, suggesting extensive hardwood forests. The ancient, primitive Clavatipollenites had become rare except in Australia. Gondwanaland remained conifer dominated.
Coniacean—Campanian
Normapolles pollen, presumably from hardwood forests, continued to dominate eastern North America, Greenland, and Europe, which were still in contact during Coniacean—Campanian time. Western North America and eastern Asia had evidently come into contact; they shared a newly evolved pollen assemblage called Aquilapollenites . Also derived from the tricolpate type, Aquilapollenites was presumably dicot pollen, but it has no living counterpart. Some Aquilapollenites also appeared in Gondwanaland during this period. Another new derivative of the tricolpate dicot type, Proteacidites, appeared in Australia in the Coniacean; this may mark the appearance of the Proteaceae, another family in the Rosidae. The Proteaceae are a classic example of a group whose modern distribution suggests a Gondwanaland origin. In the Coniacean, it would have still been possible for the Proteaceae to migrate over land between Australia and Africa. By the Santonian, Africa was isolated.
The Gondwanaland migrations of the southern beech, Nothofagus, are especially intriguing (Axelrod and Raven 1982; Schuster 1976). A member of the Fagaceae and almost certainly originally from the northern hemisphere, Nothofagus appeared in the fossil record in South America, Australia, and New Zealand in Senonian time. Its progenitor had evidently migrated from North to South America during one of the intermittent Cretaceous contacts and had continued through Gondwanaland before the link between South America and Australia via Antarctica was broken. It is unlikely that Nothofagus could have made this migration except over a continuous land path. The seed dispersal radius of living Nothofagus is very short, and the trees are dependent on symbiotic mycorrhizal fungi that are not dispersed with the seed.
Maestrichtian
The sharp distinction between the angiosperm floras of eastern North America—Europe and western North America—eastern Asia lasted into the early Maestrichtian. The discreteness of these provinces was destroyed in the late Maestrichtian, when the epicontinental seas that had bisected the continents withdrew. The pollen record then shows general mixing of Normapolles and Aquilapollenites taxa.
From the Maestrichtian on, paleobotanists are less cautious about assigning
angiosperm fossils to living families and even genera. Muller (1981) recognized 31 extant families as present in the Maestrichtian, including those noted above as present in earlier periods.
It is customary to characterize Cretaceous angiosperm evolution as explosive, with some justification. Hughes (1976) estimated that at the start of the Cretaceous, the world had about 3,000 species of vascular plants, roughly half of them ferns and half gymnosperms. He estimated that by the Maestrichtian, there were about 2,500 surviving ferns and gymnosperms and about 20,000 species of angiosperms. The explosion had scarcely begun then; the world now has about 285,000 angiosperm species in about 300 families.
The diversification of the angiosperms is commonly credited to their specialized symbioses with animal pollinators and seed dispersers. Escape from wind pollination and wind dispersal allowed origin and survival of species growing in special scattered habitats and forming complex associations rather than dominating extensive, simple stands. What little evidence there is on Cretaceous angiosperm reproduction, however, suggests that angiosperms were then mostly generalists, with pollen dispersed by wind and unspecialized insects and seeds dispersed largely by wind and water. By the Maestrichtian, some coadaptation for pollination and dispersal by specific animal groups was evidently finally beginning, but major diversification of the most important modern pollinators and dispersers, namely butterflies, moths, bees, birds, and bats, was still far in the future.
Cretaceous—Tertiary Transition
(Hickey 1984; Pillmore et al. 1984; Smit and Kaars 1984; Tschudy and Tschudy 1986; Wolfe and Upchurch in press)
Controversy is currently very active over a hypothesis that the Cretaceous ended with a bang: a huge asteroid impact caused clouds of debris to darken the earth and shut down photosynthesis, resulting in mass mortality and extinctions of many marine and terrestrial species. There is no doubt that there were major changes in flora and fauna at the Cretaceous—Tertiary transition. The argument is over the causes and whether the changes were nearly instantaneous or spread out over perhaps 10,000 to 100,000 years or more.
So far, only a little evidence on both sides of the controversy has been adduced from the fossil record of seed plants.
Evidence from various sites in the Rocky Mountain region suggests that several fossil pollen taxa, including most kinds of Aquilapollenites, disappeared abruptly at the end of the Cretaceous. Disappearance of the angiosperm
pollen was followed by a temporary increase of fern spores from less than 30% to nearly 90% of the microfossil record. According to Wolfe and Upchurch (in press), the subsequent return to dominance of angiosperms followed a pattern of normal ecological succession but at a slower rate; this implies migration from survivors outside the region.
Axelrod (personal communication 1985) suggested that such changes might have been due to regional volcanic ashfalls rather than a global phenomenon. Hickey (1984) described floristic changes at the Cretaceous—Tertiary transition as geographically variable and gradual. Aquilapollenites taxa reached their peak diversity at the end of the Maestrichtian, but their decline extended through the Paleocene until extinction in the Eocene, a span of about 10 million years. In many other regions of the world, Hickey (1984) described floristic changes at the end of the Cretaceous as slight to moderate, with Cretaceous plant species persisting after dinosaur extinctions.
The argument is not over.
Paleogene (Paleocene, Eocene, And Oligocene
(Axelrod 1984; Axelrod and Raven 1978, 1985; Daghlian 1981; Dilcher 1973; Germeraad et al. 1968; Hickey 1984; Hos 1975; Kemp and Harris 1975; Lakhanpal 1970; Leopold 1969; Muller 1981; Penny 1969; Raven and Axelrod 1974; Stebbins 1981; Tanai 1972; Tiffney 1985; Tschudy 1984; Wolfe 1972)
Whatever extinctions took place at the Cretaceous—Tertiary transition, a variety of seed plant lineages came through and radiated rapidly in the Paleogene, when a great many living genera of conifers and angiosperms first appear in the fossil record.
The global configuration of continental contacts and separations changed drastically during the Paleogene. The North Atlantic continued to drive an oceanic wedge northward, and by the Oligocene, North America—Greenland and Europe were separated. Asia and Alaska, however, remained in contact, sea levels remained low, and there may have been no other major oceanic barriers to migration in Laurasia. In contrast, the breakup of Gondwanaland became complete during the Paleogene. Africa was an island through the whole period, except for possible brief contact with Eurasia. India separated from Madagascar early in the Paleocene and moved northward to collide with Asia in the Eocene. By late Eocene or early Oligocene, South America, Antarctica, and Australia had separated and Australia was moving into lower latitudes.
For coastal angiosperms, the oceans probably continued to be pathways
of migration rather than barriers. For example, Nypa, a mangrove swamp palm, which had first entered the fossil record in the East Indies in Late Cretaceous time, evidently migrated westward by the Tethys Sea route and across the widening Atlantic Ocean. In the Paleocene and Eocene, Nypa pollen and its sea-borne fruits were present in Southeast Asia, Australia, Europe, West Africa, and on the Atlantic coasts of both North and South America. Nypa is not known to have survived outside the tropical Indo-Pacific Ocean region after the Oligocene. Eocene pollen of Nypa and other mangroves, including Avicennia, Rhizophora, and Sonneratia, and of the beach tree, Barringtonia, has been found in southwestern Australia, which was situated south of 50°S at that time; this is a much higher latitude than is reached by any modern mangroves. Rhizophora was also present in the Paleogene of Borneo and the Caribbean. Likewise, there are widely scattered Eocene fossils attributed to various modern genera of pantropical beach plants, such as Caesalpinia in Nigeria and South America.
Transoceanic dispersal appears unlikely for the forest conifers and broadleaf hardwoods often assigned to the Arcto-Tertiary geoflora, whose ranges around the northern hemisphere were discussed previously. Some of these broad ranges, for example, of Sequoiadendron, Magnolia, and Platanus, may date from the Cretaceous, but many of the genera are not known from before the Paleogene. Perhaps some of them crossed between eastern North America and Europe in the early Paleogene before the Atlantic barrier developed, while others crossed by the longer available Bering land bridge. The relative importance of these two routes is still highly speculative. There is no evidence that the Arcto-Tertiary geoflora originated in a limited region and time span and spread as a unit. Presumably it was assembled gradually by convergence of taxa migrating over differing pathways from a variety of geographic origins. It may eventually be possible to sort out their migrational histories, but this awaits more and better dated fossil records.
There is an intriguing possibility that the northward drift of the Indian subcontinent ferried some seed plant lineages from Gondwanaland to Asia. Some Indian Paleocene and Eocene fossil floras are known from coastal and freshwater sediments associated with Deccan Traps lava flows. Some of these fossils are attributed to Podocarpus, a genus that survives in India today. Living Indian podocarps, however, belong to sections of the genus unknown in Africa or Madagascar and believed to have immigrated to southern Asia via the East Indies from Australia in much later Neogene time. Also, several of the Paleogene Indian fossils were attributed to genera of sea-dispersed coastal plants for which no ferry would have been needed, such as Nypa, Cocos, Barringtonia, Sonneratia, and Terminalia . Some of the Deccan fossils, however, belong to taxa not adapted for ocean dispersal, including Musaceae, which have a wide disjunction between Africa, Madagascar, and Southeast Asia.
Deep sea drilling has brought up Paleogene fossils from now sunken Indian Ocean regions: Broken Ridge, then perhaps part of Australia, and Ninetyeast Ridge, then perhaps a chain of volcanic islands. The floras included Araucaria, Casuarina, Proteaceae, and other taxa then present in Australia. Early Eocene floras in Australia show that Araucaria, Podocarpus, Casuarina, Nothofagus, and many genera of Proteaceae grew as far south as 60°S paleolatitude. Axelrod (1984) discussed the problem of explaining the presence of evergreen trees in polar regions. In the Oligocene, Eucalyptus and Leptospermum appeared in the Australian fossil record. It is uncertain whether their progenitors were present but undetected in the old Gondwana flora or whether they immigrated from the tropical Indo-Pacific islands.
10
Discussion Of
Prehistoric
Migrations
The clarity with which plant migrations can be resolved from the fossil record decreases drastically with increasing time into the past.
In the Late Glacial and Holocene fossil record, plants are generally assignable to living genera, if not species. There are many gaps and uncertainties, but the record is good enough to establish the presence or absence of dominant plant taxa over substantial areas and time spans. In so doing, the record reveals that in postglacial time, plant species carried out amazingly rapid and highly individualistic migrations. This fact conflicts with conceptual models that visualize vegetational associations or even whole floras as migrating ponderously as units. Also, this evidence does not support the assumption that locations of Pleistocene refugia can be deduced from present plant distributions. What have sometimes been regarded as primeval forests, both temperate and tropical, were assembled particularly late in the Holocene, and their present patterns bear no resemblance to glacial ones.
The earlier Pleistocene and Neogene records still provide evidence of past distributions of the ancestors, or close relatives of the ancestors, of living species. But the lacunae are too great and the dating too imprecise to follow migrations continuously as they proceeded. It is only possible to note geographic changes within very loose temporal and spatial brackets. The Neogene record does show that various species then had gross ranges much different from those of their present descendants, partly because present mountain ranges did not exist.
Farther into the past, it becomes increasingly uncertain whether fossils represent ancestors of much changed modern taxa or lineages that became extinct. Also, the gaps in the record become enormous, and it is only possible to discern vague outlines of rise, spread, and fall of major lineages. It is intriguing to try to relate spread of plant taxa to changes in the geography of continents as postulated by plate tectonic models. In some cases, it appears that overland pathways may have been available at the right time to allow spread of taxa that are not known to be capable of long-range transoceanic dispersal, including most gymnosperms and many angiosperms, such as Proteaceae, Nothofagus , and many other hardwood tree genera. In some other cases, seaways appear to have been available at the right time for spread of mangroves and other coastal and riparian pioneers likely to have been capable of long-range water dispersal. There is, however, too little paleobotanical evidence on dispersal mechanisms to make such interpretations more than working hypotheses.
It would obviously be a mistake to try to classify seed plants in general into those that migrate by either land or water pathways. Various taxa that are not primarily coastal or riparian and that lack adaptations for sea dispersal have certainly accomplished transoceanic migrations. This has been deduced from their ancient presence on the Galapagos, Hawaiian, and other oceanic islands. It is also demonstrated by their late appearance in the fossil record, too late for intercontinental land routes. Three of the most cosmopolitan angiosperm families, grasses, composites, and legumes, entered the fossil record during the Paleogene on various continents that were not then in contact.
Raven and Axelrod (1974) noted that the presently pantropical legume genus Acacia had crossed water barriers from South America to the West Indies by the Oligocene. Acacia has not been found in Oligocene floras of Australia, although it was formerly believed that it had been present there before the breakup of Gondwanaland. Acacia probably immigrated after Australia was completely isolated and had drifted into lower latitudes. In spite of its relatively recent arrival, Acacia has radiated into about 600 endemic Australian species, which play a major role in the continent's vegetation. Raven and Axelrod (1974) also cited the kapok family, Bombacaceae, as another pantropical family that spread by transoceanic dispersal between former Gondwanaland continents. On the species level, a member of the Bombacaceae, the kapok tree, Ceiba pentandra , was cited by Baker (1965b ) as a probable example of a noncoastal species that crossed the Atlantic in relatively recent geologic time by flotation of pods from South America to west Africa.
Part III
Migration and Evolution
11
Deductive
Interrelationships
In any sexually reproducing species, each generation can be expected to differ at least slightly from the parents in both spatial distribution and hereditary characters. It is easy to imagine the processes of migration and evolution proceeding simultaneously and interactively.
Theoretical Model Integrating Mechanisms
Of Migration And Evolution
In the Introduction, the abstract model of migration considered the process to be the result of interplay between opposing forces:
|
This obviously parallels the orthodox abstract model of evolution as the result of interplay between:
|
The two processes may be integrated by recognizing that an individual may be simultaneously a migrant and a variant. Its survival may be contingent on both its location and its genotype. A single event, its success or failure, affects both the geographic and genetic range of the species to which it belongs.
Again, as in the case of the simple migration model, it is obvious that centrifugal and centripetal forces may equilibrate. Seed dispersal and genetic recombination may be going on, but both the geographic and genetic limits of the species may be static. Intuitively, it seems that geographic and genetic equilibrium should go together.
Hypothetical Parallels And Feedbacks
Between Migration And Evolution
Given a single coherent population, geographic expansion and genetic diversification (of the population as a whole) might be expected to go hand in hand. This should hold whether the argument is based on purely demographic grounds, that is, increased survivorship and population growth, or on ecological grounds, that is, increased environmental diversity and altered selection pressures. Conversely, geographic retreat and genetic impoverishment might be expected to go hand in hand.
Given a population that has become disjunct, whether by long-range colonization or by retreat and fragmentation, the allopatric speciation model postulates consequent genetic divergence. Again, the argument can be based on either demographic grounds, that is, random differences in mutation and genetic drift in divided populations, or on ecological grounds, that is, environmental dissimilarity of separate regions. In the sympatric speciation model, genetic divergence is primary and geographic segregation secondary. In the parapatric model, geographic and genetic divergence develop together. However, in all three models, geographic and genetic divergence are interconnected. Conversely, if geographic contact is restored between temporarily disjunct populations, resumption of gene exchange might be expected to obliterate genetic divergence.
12
Case Histories Of
Evolution Associated
With Migration
As before, case histories will be restricted to spontaneous populations; evolution of domesticated plants will be excluded. Also, as before, only case histories with actual evidence of change through time will be used. Unfortunately, this includes mostly very recent changes because the fossil record is too incomplete to tell whether similar but different fossils from different time levels and regions were connected in a direct ancestor—descendant lineage or were on separate migrational and evolutionary pathways.
Local Expansion And Diversification
Of Coherent Populations
Colonization of Mine Dumps in Britain
(Antonovics 1968; Cook et al. 1972; Jain and Bradshaw 1966; Macnair 1981)
Studies of plant colonization on historical mine wastes in England and Wales have shown that toxic concentrations of the heavy metals lead, copper, and zinc exclude normal members of the local pioneer flora. Within decades, however, the barren areas begin to be invaded by genetically novel, tolerant
individuals of a few perennial grass species: Agrostis tenuis, Anthoxanthum odoratum, Festuca ovina , and a perennial ribwort, Plantago lanceolata . These are native to moors, heaths, and other open habitats over wide regions of Eurasia; they were present on nontoxic soils adjacent to the mine dumps. Careful field and experimental studies have shown sharp genetic differentiation within a few meters distance between individuals of the same species growing on normal and toxic soils; divergence includes hereditary morphological and physiological traits. Although cross-pollination by wind freely transgresses the border, rigorous natural selection eliminates hybrid progeny: only progeny of tolerant parents survive on the mine dump. Some of the tolerant grass populations have evolved capacity for increased self-pollination as compared to cross-pollination in normal populations. This barrier to gene flow suggests incipient parapatric speciation.
Mimicry of Crops by Weeds
(Barrett 1983; Harlan 1982a,b )
Weeds have migrated and evolved with crops since the Neolithic. Commonly the farmers' efforts to eliminate weeds have resulted in unintentional selection of new kinds of weeds that mimic the crop. In some cases, the weed and its host crop interbreed. For example, domestication of Asiatic rice, Oryza sativa , involved selection for a mutant, nonshattering inflorescence that retains the ripe grain until harvest rather than scattering the grain as in wild rice. Asiatic rice fields, however, are plagued with races of weed rice that have shattering inflorescences, with their seed escaping harvest. In other respects these mimic cultivated rice varieties so closely that the farmers cannot recognize them and weed them out before their seed is scattered. Recently, rice cultivars have been bred with purple leaves to mark them off from the weed races, but purple leafed weed races promptly evolved, perhaps by gene exchange with the crops. Oryza sativa has also been accompanied in its migrations by coevolving weed grasses incapable of interbreeding with the crop, for example, the mimic Echinochloa species discussed above in Chapter 4.
The great wheat of antiquity, Triticum dicoccum , also had coevolving weed mimics in other grass genera. Wild oats, Avena spp., and wild rye, Secale cereale , originally had shattering inflorescences. In some regions, they evolved weed races with nonshattering inflorescences producing grain that was harvested and spread with the wheat crop. These weed oats and rye were eventually taken into cultivation as grain crops in their own right in regions of Eurasia marginal for wheat. Cultivated rye later picked up a weed mimic of its own, Bromus secalinus , with a nonshattering inflorescence unlike the wild type.
In Mexico, wild maize or teosinte, Zea mexicana , has spread and evolved as a weed in milpas where its domesticated derivative, Zea mays , was planted. Characteristics bred into maize by artificial selection, such as wide, red leaves, are also acquired by teosinte, presumably by interbreeding, except that the weed retains the wild type shattering inflorescence and the grain escapes harvest. In Sinaloa, Mexico, where a practice was begun in the 1920s of rotating maize with pasture in alternative years, the local teosinte has evolved a dormancy mechanism by which it escapes germination and grazing during fallow years and germinates in phase with the next maize planting.
Currently, weeds are commonly evolving the same reaction spectra to herbicides (intended to control them) as the crops they infest; highly resistant races are evolving very rapidly. An example is the European weed, Abutilon theophrasti , which is a major pest of maize and soybeans in the U.S. corn belt (Spencer 1984).
Evolution of Weeds from Cultivars
(Harlan 1982a ; Jain and Martins 1979; Oka and Morishima 1982)
In prehistoric Africa, domestication of grain sorghum, Sorghum bicolor , initiated separate cultivar lineages with two different mutations, either one of which causes nonshattering when present as a homozygous recessive. If cultivars of both kinds are brought together and interbreed, some of the progeny revert to the wild type shattering habit and their grain escapes harvest to grow as a weed in sorghum or other crops. Similarly, domesticated oats, Avena sativa , with nonshattering inflorescence, gave rise to Avena fatua , which has reverted to a shattering inflorescence like true wild oats but is a specialized weedy type confined to crops and margins of cultivated land. Avena fatua was accidentally introduced to Australia as a weed of grain crops; within 50 years, it had evolved new races there, differentiated according to local crop rotation practices.
In California, rose clover, Trifolium hirtum , was introduced from the Mediterranean as a forage crop in 1946. In various places in the Sierra Nevada foothills, it has escaped from range plantings to roadsides. Within about 20 years, these escapes have evolved subtle differences in plant morphology and seed germination that evidently make them better adapted for roadside rather than pasture conditions.
Comment
The few cases cited of parallel geographic spread and evolutionary diver-sification all involved human dispersal or artificial selection. It is easy to
imagine comparable cases involving changes in natural dispersal or selection, but these would be hypothetical rather than actual histories. This discrepancy is probably partly because plants closely associated with mankind are more likely to be closely observed, but mainly because mankind has caused the most drastic recent changes in plant dispersal and selection.
Geographic Retreat And Genetic Impoverishment
Of Coherent Populations
Although it seems likely that these processes would go hand in hand, recorded case histories of impoverishment short of extinction are not available.
Geographic Disjunction And Genetic Divergence
Of Separate Populations
The Slender Wild Oat in Central California
(Jain 1983)
The European Avena barbata was introduced to the San Francisco Bay region over 100 years ago and forms extensive stands in coastal grasslands as well as isolated roadside colonies. The roadside stands generally show little or no genetic differentiation within stands, but do show some differentiation between stands. The large, continuous grassland stands are more polymorphic. Jain (1983) attributed the lower polymorphism of the isolates mainly to random founder effects and genetic drift rather than to adaptive differentiation.
Endemics on Juan Fernandez Islands
(Stuesy et al. 1984)
Lavas that formed these three islands off the Chilean coast have been potassium-argon dated, the youngest at about 1 million B.P. , the oldest at about 5 million B.P. The native flora includes about 150 angiosperm species, of which about 100 are endemic. One magnolialike shrub species and one palm belong to genera known only from these islands, Lactoris and Juanea ,
respectively. The other endemic species belong to genera shared with Chile. The Lactoris and Juanea are believed to be older than the islands and to survive there as relicts after extinction on the mainland. Many of the endemic species presumably evolved on the islands.
Comment
Neither of these cases provides much evidence of a close parallel between geographic and genetic divergence. Genetic differences among local California wild oat populations are too slight to signal initiation of progressive evolutionary separation. On another level, there is no proof available that the species in California has diverged from its ancestral Mediterranean population. Only about a century has elapsed, but a plant breeder would expect substantial changes in isolated and differentially selected populations in less than 100 generations. There are innumerable similar cases of weeds that have shown little or no apparent evolutionary divergence of geographically isolated populations after a century or centuries.
The abundant cases of endemics on oceanic islands, like the Juan Fernandez case, are often assumed to be examples of allopatric speciation. However, even if the species really evolved on the islands rather than being relicts of extinct mainland types, there are alternative possibilities. Lack of gene flow between mainland and island populations of a species may be irrelevant. Endemism may instead be due to the limited number of species able to reach the islands. With an impoverished flora, wide evolutionary opportunities were available to the few kinds of plants on the ground. Sympatric or parapatric speciation within a single island may have produced multiple species in different environments, not merely one species per island. For example, in the three Juan Fernandez islands, one genus in the Asteraceae, Dendroseris , has evolved 11 species on Masatierra and 3 on Masafuera.
Geographic Overlap And Hybridization
Between Formerly Discrete Species
Introgression Between Native Species in Disturbed Habitats
(Anderson 1949; Fassett and Sauer 1950; Harlan 1982b ; Sauer 1957, 1972)
Anderson's (1949) classic book on introgressive hybridization presented the case history of two Mississippi Delta iris species as a prime example. The
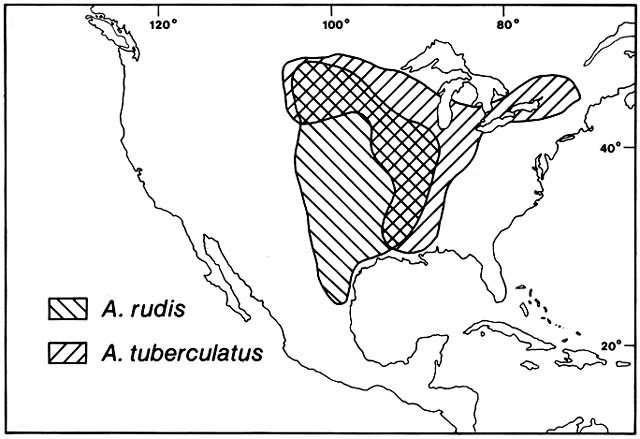
Figure 14. Present Distributions of Two Riparian Amaranthus Species Native to the Midwest. Until mid-nineteenth
century, there was little overlap or hybridization between these species. A. rudis was preadapted to become a weed
of agricultural clearings in floodplain forests. Since the late nineteenth century, A. rudis has invaded much of the territory
of A. tuberculatus . In the zone of overlap massive hybrid populations have formed, but these have remained concentrated
in cultivated fields while pure A. tuberculatus populations still occupy nearby lake shores and stream banks.
two species are related and interfertile but differ strikingly in many characters, the most conspicuous being that Iris fulva plants are low growing and have red flowers, while I. giganticaerulea is tall and produces blue and yellow flowers. The two have parapatric, that is, contiguous but not overlapping, distributions in natural habitats in the delta. Iris fulva grows in the semishade of gallery woodlands along the back margins of natural levees, where it is subject to shallow flooding, whereas I. giganticaerulea grows in full sun in the grassy marshes behind the natural levees, where it is subject to deeper flooding.
For more than 100 years, French farmers have been settling along the natural levees. By the early 1900s, cleared and pastured areas on their farms were being colonized by swarms of amazingly varied iris, some of them so beautiful that they have become favorite garden varieties. Initially, some botanists mistook these hybrid swarms for a whole suite of ancient unnamed Iris species, but it was soon recognized that the complex resulted from colonization of recently disturbed sites by hybrids between the two species. A few of these sites have been opened up by changes in river channels, but the bulk are due to human activities. Anderson (1949) coined the term introgression for such limited hybridization between species that remain essentially discrete in their original, undisturbed habitats, with few genes of one species introduced into the germplasm of another.
The large genus Amaranthus includes a small group of 10 dioecious species, all native to North America and all annual pioneers of riverbanks, lakeshores, coastal marshes, or sea beaches. Their massive herbarium record shows that during the last 150 years, some of the species have stayed in their natural habitats and have had nearly static ranges, while others have expanded into artificially disturbed sites, where they have met and hybridized with other Amaranthus species. Only two of these species will be discussed here.
Amaranthus tuberculatus has a gross range like a great T, spanning the lower Great Lakes region and extending down the Mississippi Valley (fig. 14). It is at home on the margins of freshwater marshes, bogs, lakes, and streams. The seedlings commonly colonize a narrow zone where falling water level has exposed a strip of bare sand or mud. Where artificial disturbance opens ground close to its natural habitat, as in floodplain corn fields, A. tuberculatus commonly moves in as a weed; elsewhere it is rarely found as a weed. Its gross range has been almost static since the beginning of the herbarium record. Amaranthus rudis originally had a gross range confined to Kansas, Missouri, Oklahoma, and Texas. It was also a riparian pioneer, especially common on river sandbars after spring floods. Its native range was in contact with that of A. tuberculatus in Missouri. The two species are sharply distinct morphologically, but they form fertile hybrids, a few of which are known
from the mid-nineteenth century. Starting late in the nineteenth century, A. rudis began expanding its range northward and eastward into territory of A. tuberculatus , invading a new state about every decade until 1970. In the zone of overlap, natural riparian habitats have remained occupied mainly by unhybridized A. tuberculatus , while artificially disturbed habitats have been invaded by either A. rudis or A. rudis × A. tuberculatus hybrids. In the early stages of the invasion, until about 1905, most of the weed populations in the overlap zone were hybrids. Since 1905, after buildup of weed populations, A. rudis has become increasingly abundant there.
In tropical America, two distantly related and morphologically very distinct species of Phytolacca are native to riparian and other naturally open habitats at contrasting elevations: Phytolacca rivinoides in the tropical lowlands and P. rugosa in the temperate highlands above about 2,000 m. Presumably, prehistoric Indian agriculture at intermediate elevations provided openings where these species met and hybridized. Under modern human occupation, hybrid swarms became abundant along roads and in agricultural clearings between about 1,200 and 2,500 m elevation.
A very similar case of introgression involves two grass species native to the Karakoram and western Himalaya. Bothriochloa ischaemum is common above the present tree line and B. bladhii in dry valleys below the forest zone. Neither species is a forest grass, and the two were probably not in contact until the last few centuries when the forests have been largely cleared for agriculture. Bothriochloa ischaemum has extended its range downslope and B. bladhii has climbed upslope, so the two now commingle. In the hybrids, characters of B. ischaemum can be detected 1,000 m below the elevational limits of the pure species and characters of B. bladhii range 1,000 m above the limits of the pure species.
Hybridization Following Long-Range Introduction
(Baker 1972; Barnes 1972; Barnes and Cottam 1974)
Occasionally an exotic species, recently imported by humans, has met a native congener with which it can interbreed. For example, Nicotiana glauca , a riparian pioneer native to South America, has naturalized along desert washes in Australia, where it met and interbred with a wild Australian tobacco, N. suaveolens . Similarly, a mint, Prunella vulgaris var. vulgaris , native to Eurasia, was introduced to North America, where it became a common lawn weed. In California it has met and interbred with P. vulgaris var. lanceolata , native to North America grasslands and the margins of conifer forests.
There are various cases where two introduced species have hybridized outside their native lands. For example, two attractive Asiatic honeysuckles have formed hybrid swarms in North America, where they were imported as ornamental shrubs. Lonicera tatarica , native to riparian sites from the Caspian to Lake Baikal and Mongolia, was introduced to the United States in 1752 and widely planted. A century later, L. morrowii , native to the coasts of Japan, was brought back to the United States by Commodore Perry's expedition and also widely planted. Interspecific hybrids, named L. × bella , are fully fertile; they have also been widely planted. Both species and their hybrids have berries attractive to birds. Their seedlings have colonized both natural and artificial habitats from Alberta and Nova Scotia down to Missouri and the Carolinas.
Lonicera × bella has been intensively studied in the University of Wisconsin Arboretum, where it has become a serious weed in what are supposed to be communities of native species. It was planted there in the mid-1930s during development of the Arboretum by the Civilian Conservation Corps. It is now present in every plant community, from swamps to dry prairies, being least aggressive in the densest hardwood and conifer forests. Lonicera × bella has hybrid vigor and grows to 6 m tall, twice as tall as its parents. It is entirely free from pests and disease. A single shrub can produce 3,500 berries a year, each with about 6 seeds, which are spread by common bird species. Some of the seeds germinate immediately, others lie dormant. The hybrid also spreads vegetatively. It begins growth about a month before the native shrubs and retains green foliage almost a month later than they do in the fall. It competes aggressively, except under very dense canopies, with such dominant native understory species as Cornus racemosa .
Allopolyploid Speciation Following Long-Range Migration
(Marchant 1967, 1968, 1977; Musselt 1952, Ownbey 1950; Ranwell 1967, 1972; Roose and Gottlieb 1976; Rosser 1955)
When two species are capable of producing a sterile diploid hybrid, it may be possible for a third, polyploid species to arise, either by somatic chromosome doubling in the sterile F1 or by union of unreduced sperm and egg. In either case, the resulting allopolyploid may be fully fertile and capable of sexual reproduction, but barred from interbreeding with either parent. Many new crop species and ornamental cultigens have originated in this way.
The classic example of modern allopolyploid speciation in a natural habitat occurred in the saltmarsh grass genus Spartina . The new species originated where a diploid species native to the Atlantic coasts of North
America, S. alterniflora , had been introduced to marshes inhabited by a European diploid species, S. maritima. Spartina alterniflora probably arrived on the Atlantic coasts of Europe about 1800 in ballast dumped by ships. It was found growing on the French coast on the Bay of Biscay in 1803 and on the English coast near Southampton soon after, in both cases invading territory of S. maritima . Sterile diploid interspecific hybrids (2n = 62) appeared near Southampton by 1870, named S. townsendii , and on the Spanish Bay of Biscay coast by 1894, named S. neyrautii . These F1 hybrids were vigorous and spread locally vegetatively.
The allopolyploid (4n = 120 +) appeared near Southampton about 1890; it has since been named S. anglica . Sexually fertile, S. anglica spread rapidly by seed and was also widely planted in Britain and on the continent to stabilize coastal sediments. The clones produced by vegetative spread are long-lived. Experiments with S. anglica show that it tolerates a wide range of salinity and can survive continuous submergence in clear seawater for over 4 months. It is thus more capable of growing from the inner margins of a salt marsh to lower levels than any other marsh plant. It has commonly completely replaced diploid Spartina and other marsh species on British and continental European coasts. By the 1970s, S. anglica had been extensively planted for mudflat stabilization along the coasts of China and sporadically in many other places. It has not succeeded in the tropics or where winters are too severe. Its total area worldwide is probably over 50,000 ha.
As discussed above in Chapter 4, after introduction to Britain, Senecio squalidus spread widely as a ruderal. It is a diploid (2n = 20). It met and hybridized with a polyploid, Senecio vulgaris (4n = 40), native to British sea beaches and also a common weed. Some of the hybrids are sterile (3n = 30) and rare. Others are fertile allopolyploids (6n = 60), named S. cambrensis , which have become ruderal weeds; these were first found in Wales in 1948.
Another genus in the Asteraceae, Tragopogon , has given rise to new allopolyploid species in the present century. Three European diploid species, all called goat's beard, are involved; all three have become widely naturalized in the western United States as ruderals and field weeds. Tragopogon dubius and T. porrifolius have repeatedly formed sterile F1 hybrids and also a fertile allopolyploid, T. mirus , which originated independently in Washington state and Arizona. Similarly, T. dubius and T. pratensis have repeatedly formed sterile F1 hybrids and also a new allopolyploid species, T. miscellus , which evidently arose independently in Idaho, Montana, and Wyoming.
Comment
A large proportion of the world's flora consists of polyploid species, mostly prehistoric in origin. In many cases, the genomes that were assembled
in ancient polyploid species have been identified with those of living diploid species, and in some cases the polyploid has been resynthesized experimentally by artificial hybridization. This contradicts an orthodox dogma in taxonomy that species have a single origin in space and time.
A generally accepted example of ancient allopolyploid species is found in the genus of cotton, Gossypium . Cotton geneticists believe that the common polyploid species, including G. hirsutum (4n = 52), originated in the Pleistocene by hybridization on tropical American seacoasts between native diploids (2n = 26) and an African diploid with seed dispersed by ocean currents. More speculative is the hypothesis that Sequoiadendron (2n = 22) and Metasequoi (2n = 22) hybridized in Cretaceous North America to produce Sequoia (4n = 44).
Both the cases of introgression and allopolyploid speciation show some sort of interplay between the processes of migration and evolution. They do not, however, fit a simple model of parallel geographic and genetic coalescence when formerly discrete species come into contact and interbreed.
13
Migration Without
Evolution?
The preceding chapter offered only a few examples of the interplay between the processes of migration and evolution. The meagerness of the record can be attributed partly to the recency of efforts to observe genetic changes in natural plant populations, the difficulty of monitoring such changes, and the limited manpower devoted to the task. There can be no doubt that the processes can interact. Obviously, evolution of a successful novel adaptation, whether for dispersal or survival, allows geographic expansion of a species. In the few widespread species that have been carefully studied genetically, local races or ecotypes have commonly evolved in different latitudes, altitudes, and edaphic situations. These have usually been detected by experimental transplants and would not be evident in the historical or fossil record.
It does not logically follow, however, that migration is necessarily always dependent on or accompanied by evolution. Most of this book has dealt with cases of migration, pure and simple, with little or no evidence of parallel evolutionary changes. Cases ranged from extremely local to global and included migrations set off by changes in both dispersal and environment. Whatever the undetected genetic changes, the fact remains that there can be a strong asymmetry: geographic changes have been obvious while genetic ones have not. Some plant species have moved complacently, without evident evolution between distant and environmentally dissimilar regions. Also, some have persisted without apparent evolution through long time spans. Unlike mammals, many modern-appearing seed plant species were already in existence before the climatic cycles and orogenic upheavals of the Pleistocene. For example, in western North America, different species of mountain mahogany, Cercocarpus , have been remarkably stable since the Miocene
(Searcy 1969). Similarly, the five mangrove species whose ranges were sundered in the Tertiary by rise of the Panama land bridge have remained taxonomically indistinguishable on the Caribbean and Pacific coasts; this is in spite of dissimilar rainfall and tidal ranges on the two coasts. Clearly, species can be far more plastic geographically than genetically.
These observations recall an old idea, recently being seriously reconsidered, that evolution may commonly be concentrated in very rapid events, so-called macroevolution or punctuated equilibrium, rather than proceeding continuously by small steps, so-called phyletic gradualism. It has been argued that phyletic gradualism is very rare, that most species during their geologic history either do not change or fluctuate mildly in morphology (Gould 1984; Gould and Eldridge 1977; Stanley 1979). Carson (1982) suggested a connection between the stasis observed in fossil morphology over long geologic time and the evidence from living species that highly variable gene pools may be maintained by stabilizing selection in a state of balanced equilibrium. The fossil record shows such stasis for some seed plants, but is not continuous enough to determine whether their origins were abrupt or gradual.
The hypothesis of stabilizing selection is related to one proposed by Baker (1965a ) for a special group of pioneer plants, the so-called colonizing species. Baker characterized these plants as having general purpose genotypes adapted for a broad spectrum of nonuniform, unstable, rapidly changing environments. They have been selected for dispersibility of seed to exploit disjunct and often ephemeral habitats. Evidently, stabilization of a compromise genotype allows maintenance of larger populations in variable environments than could be maintained by constant genetic tinkering. Finer tuned adaptations might be of temporary advantage in some situations but would preclude exploitation of others. Baker's general purpose genotype concept is widely accepted for ecological pioneer plants, especially weeds. It may apply to many other kinds of plants as well. The records of migration outlined in Chapter 6 show that forest tree species that are now members of late successional or climax communities were colonizing species in Pleistocene and Holocene time. They were highly dispersible and moved very rapidly into disjunct, nonuniform habitats. Such migrations were carried out by members of a wide spectrum of modern communities, from boreal forest to tropical rain forest, and in various continents.
Over a much longer geologic time span, the whole record of gymnosperm and angiosperm migrations suggests that colonizing ability is a primitive and general trait inherited in different degrees by most seed plant taxa, including those with the longest life expectancy through geologic time.
Truly sedentary species incapable of macromigration may have run into evolutionary dead ends; they may be trapped in local habitats with a very limited future. Some may have formerly been wide-ranging colonizers, such as Ginkgo or Lyonothamnus . Others may have evolved into narrow endemics
by selection for finely tuned environmental adaptations and low dispersibility. This process probably occurred mainly in habitats that were very isolated and discrete from their surroundings, classic examples being on rugged oceanic islands (Carlquist 1974). Such endemics tend to lose their primitive colonizing ability because their seed is mostly wasted when dispersed to a distance. Even if a freak, long-range dispersal succeeds, the genes are lost to the parent population because of lack of return dispersal.
To the extent that it consisted of colonizing species, the earth's flora was preadapted to survive with Homo sapiens . Many of the case histories in Part I demonstrate how colonizing species were able to exploit the catastrophic effects of human habitat disturbance and human seed dispersal. As with natural changes in habitat and dispersal, human impact had asymmetrical effects on plant migration and evolution. In wild plant species and to a slightly lesser extent in weeds, human intervention has had much more drastic consequences in plant geography than in heredity. The same is not always true of domesticated plants, but that is another story.
14
Conclusion
We have seen that similar plant distribution patterns often develop by convergent rather than parallel migrations. Let me recall three examples on different time scales:
|
Because plant taxa are normally much less conservative in their geographic ranges than in their hereditary characteristics, migrational reconstructions require more complete fossil and historical evidence than do phylogenetic
ones. As a science, plant migration is in a more primitive state than plant evolution, and we probably recognize only dimly how much we need to know. At present, generalizations about migration, whether inductive or deductive, are likely to be credible and interesting only if they specify some limits on taxonomic groups and geologic time spans. Any laws that exist to govern processes of plant migration have not been immutable but have evolved with the earth and its flora.
Rules governing geographic patterning of marine algae dispersed by ocean currents obviously ceased to be universal when the first plants emerged onto the land. The early land plants, with global wind dispersal of spores and with local survival in wet habitats, may have followed Beijerinck's law: everything is everywhere but the environment selects.
When the first seed plants evolved, they had a different law because their migrations were more constrained by dispersal and less by survival. Pijl (1982) postulated that the pteridosperms and other early gymnosperms were not well adapted for dispersal by water or wind but that they evolved mechanisms for internal seed transport by reptiles. He suggested that this involved developing attractive, juicy outer seed coats or edible arils together with indigestible, hard, inner seed coats. Similar structures persist in many living gymnosperm groups: cycads, Ginkgo, Gnetales, podocarps, and yews. He suggested that later in the Cretaceous birds became more important than reptiles in seed dispersal. Entry of birds as long-range dispersal agents must have changed the rules of plant migration as drastically as did the entry of mankind about 100 million years later.
With the origin of the angiosperms, the plot thickened again. Although the angiosperm ovary now commonly functions in seed dispersal, it may have originally functioned in other stages of the sexual cycle, for example, in protecting young ovules against chewing by pollinating beetles or in screening out foreign pollen. Pijl (1982) suggested that in primitive angiosperms, the ovary was merely a hindrance to seed dispersal and burst open to release seeds for dispersal on their own, as in magnolias and other supposedly primitive living angiosperms. Whatever its original function, however, the ovary was soon exploited in a variety of ways to promote seed dispersal. In coastal and riparian angiosperms, perhaps an early and simple adaptation was evolution of buoyant fruits. In other cases, the ovary became a juicy fruit that took over the former function of the seed in attracting birds and later mammals.
Understanding the rules governing seed plant migration requires recognizing dispersal as a significant variable. Such understanding, of course, also requires recognizing environmental selection as another significant variable. I will not try to recapitulate here the diverse ways in which species migrations have been channeled by environment, a basic theme throughout this book.
We have seen species borders respond to changes in their physical and biotic environments over a huge range of temporal and spatial scales. We have also seen that through time the rules of survival, like those of dispersal, have become more complex, largely because of the increasing diversity of the flora.
I am pleased rather than disappointed that I can see no prospect of easy, simple generalization about seed plant migration.
Appendix
|
|
|
|
|
|
|
|
|
References
Aellen, Ernst. 1958. Beitrag zur Kenntnis der Ausbreitung binnenlandischen Halophyten durch Vogel. Bauhinia 1:85-89.
Anagnostakis, Sandra L. 1982. Biological control of chestnut blight. Science 215:466-469.
Anderson, Edgar. 1949. Introgressive Hybridization . 109 pp. John Wiley, New York.
Anderson, Edgar. 1952. Plants, Man and Life . 245 pp. Little, Brown, Boston.
Antonovics, Janis. 1968. Evolution in closely adjacent plant populations. V. Evolution of self-fertility. Heredity 23:219-238.
Arno, Stephen F. 1980. Forest fire history in the northern Rockies. Journal of Forestry 78:460-465.
Arnold, Chester A. 1969. The fossil plant record, pp. 127-143. In Robert H. Tschudy and R. A. Scott, eds., Aspects of Palynology . Wiley-Interscience, New York.
Axelrod, Daniel I. 1958a . The Pliocene Verdi flora of western Nevada. University of California Publications in Geological Sciences 34:91-160.
Axelrod, Daniel I. 1958b . Evolution of the Madro-Tertiary Geoflora. Botanical Review 24:433-509.
Axelrod, Daniel I. 1966. The Pleistocene Soboba flora of southern California. University of California Publications in Geological Sciences 60:1-79.
Axelrod, Daniel I. 1973. History of the Mediterranean ecosystem in California, pp. 225-277. In F. di Castri and H. A. Mooney, eds., Mediterranean Ecosystems: Origin and Structure . Springer-Verlag, New York.
Axelrod, Daniel I. 1974. Revolutions in the plant world. Geophytology 4:1-6.
Axelrod, Daniel I. 1978. The origin of coastal sage vegetation, Alta and Baja Calfornia. American Journal of Botany 65:1117-1131.
Axelrod, Daniel I. 1980. Contributions to the Neogene paleobotany of central California. University of California Publications in Geological Sciences 121:1-212.
Axelrod, Daniel I. 1983a . New Pleistocene conifer records, coastal California. University of California Publications in Geological Sciences 127:1-108.
Axelrod, Daniel I. 1983b . Biogeography of oaks in the Arcto-Tertiary province. Missouri Botanical Garden Annals 70:629-657.
Axelrod, Daniel I. 1984. An interpretation of Cretaceous and Tertiary biota in polar regions. Paleogeography, Paleoclimatology, Paleoecology 45:105-147.
Axelrod, Daniel I. 1985a . Miocene floras from the Middlegate Basin, west-central Nevada. University of California Publications in Geological Sciences 129:1-279.
Axelrod, Daniel I., and P. H. Raven. 1978. Late Cretaceous and Tertiary vegetation history of Africa, pp. 77-130. In M. J. A. Werger, ed., Biogeography and Ecology of Southern Africa . W. Junk, The Hague.
Axelrod, Daniel I., and P. H. Raven. 1982. Paleobiogeography and origin of the New Guinea flora, pp. 919-941. In J. L. Gressitt, ed., Biogeography and ecology of New Guinea. Monographiae Biologicae . W. Junk, The Hague.
Axelrod, Daniel I., and P. H. Raven. 1985. Origins of the Cordilleran flora. Journal of Biogeography 12:21-47.
Baker, Herbert G. 1965a . Characteristics and modes of origin of weeds, pp. 147-172. In Herbert G. Baker and G. Ledyard Stebbins, eds., The Genetics of Colonizing Species . Academic Press, New York.
Baker, Herbert G. 1965b . The evolution of the cultivated kapok tree: a probable West African product, pp. 185-216. In David Brokensha, ed., Ecology and economic development in tropical Africa. University of California, Berkeley, Institute for International Studies, Research Series .
Baker, Herbert G. 1972. Migration of weeds, pp. 327-347. In D. H. Valentine, ed., Taxonomy, Phytogeography and Evolution . Academic Press, London.
Baker, Herbert G. 1983. Ceiba pentandra (Ceyba, Ceiba, Kapok Tree), pp. 212-215. In Daniel H. Janzen, ed., Costa Rican Natural History . University of Chicago Press, Chicago.
Baker, Richard G. 1983. Holocene vegetational history of the western United States, 2:109-128. In H. E. Wright, Jr., ed., Late Quaternary Environments of the United States . University of Minnesota Press, Minneapolis.
Bakker, D., and W. van der Zweep. 1957. Plant migration studies near the former island of Urk in the Netherlands. Acta Botanica Neerlandica 6:60-73.
Bambach, Richard K., Christoper R. Scotese, and Alfred M. Ziegler. 1980. Before Pangaea: the geographies of the Paleozoic world. American Scientist 68:26-38.
Bank, Theodore P., II. 1953. Ecology of prehistoric Aleutian village sites. Ecology 34:246-264.
Barbour, Michael G., and James E. Rodman. 1970. Saga of the west coast sea rockets: Cakile edentula ssp. californica and C. maritima. Rhodora 72:370-386.
Barlund, Ulla. 1940. Uber die Einwanderung der Pflanzen nach einem Archipelago von Kleininseln in den Scharen Alands. Memoranda Societatis pro Fauna et Flora Fennica 15:216-220.
Barnard, P. D. W. 1973. Mesozoic floras, pp. 175-187. In N. F. Hughes, ed., Organisms and continents through time. Paleontological Association, London, Special Papers .
Barnes, R. F. W. 1983. Effects of elephant browsing on woodlands in a Tanzanian National Park: measurements, models and management. Journal of Applied Ecology 20:521-540.
Barnes, William. 1972. Some aspects of the ecology of honeysuckle in the Arboretum. University of Wisconsin Arboretum News 21(2):1-6.
Barnes, William, and Grant Cottam. 1974. Some autecological studies of the Lonicera x bella complex. Ecology 55:40-50.
Barrau, Jacques. 1980. Indigenous and colonial land-use systems in Indo-Oceanian
savannas: the case of New Caledonia, pp. 253-265. In David R. Harris. Human Ecology in Savanna Environments . Academic Press, London.
Barrett, Spencer C. H. 1983. Crop mimicry in weeds. Economic Botany 37:255-282.
Bartolome, James. W., and Barbara Gemmill. 1981. The ecological status of Stipa pulchra (Poaceae) in California. Madroño 28:172-184.
Batchelor, B. C. 1979. Discontinuously rising late Cainozoic eustatic sea-levels, with special reference to Sundaland, Southeast Asia. Geologie en Mijnbouw 58:1-20.
Batten, David J. 1981. Stratigraphic, palaeogeographic and evolutionary significance of Late Cretaceous and early Tertiary Normapolles pollen. Review of Palaeobotany and Palynology 35:125-137.
Baumann, Robert H., John W. Day, Jr., and Carolyn A. Miller. 1984. Mississippi deltaic wetland survival: sedimentation versus coastal submergence. Science 224:1093-1095.
Beaman, John H. 1961. Vascular plants on the cinder cone of Paricutin volcano in 1960. Rhodora 63:340-344.
Beatley, Janice C. 1976. Vascular plants of the Nevada Test Site and central-southern Nevada: ecologic and geographic distributions. U.S. Energy Research and Development Administration TID-26881:1-308.
Beck, C. B., ed. 1976. Origin and Early Evolution of Angiosperms . 341 pp. Columbia University Press, New York.
Becker, Ronald E., ed. 1981. Land loss: coastal zone crisis. Louisiana State University, Sea Grant College Program Aquanotes 10(3):1-6.
Bell, Frances G. 1969. The occurrence of southern, steppe and halophyte elements in Weichselian (Last Glacial) floras from southern Britain. New Phytologist 68:913-922.
Bennett, K. D. 1985. The spread of Fagus grandifolia across eastern North America during the last 18,000 years. Journal of Biogeography 12:147-164.
Berthoud, Edward L. 1892. A peculiar case of plant dissemination. Botanical Gazette 17:321-326.
Bertsch, Karl. 1953. Geschichte des Deutschen Waldes . 4th ed. 124 pp. Gustav Fischer, Jena.
Beug, Hans-Jurgen. 1982. Vegetation history and climatic changes in central and southern Europe, pp. 85-102. In Anthony F. Harding, ed., Climatic Change in Later Prehistory . Edinburgh University Press, Edinburgh.
Billings, W. D. 1938. The structure and development of old-field shortleaf pine stands and certain associated physical properties of the soil. Ecological Monographs 8:437-499.
Birger, Selim. 1907. Die Vegetation einiger 1882-1886 entstandenen schwedischen Inseln. Botanische Jahrbücher für Systematik, Pflanzengeschichte und Pflanzengeographie 38:212-232.
Black, R. Alan, and L. C. Bliss. 1980. Reproductive ecology of Picea mariana (Mill.) BSP at treeline near Invik, Northwest Territories, Canada. Ecological Monographs 50:331-354.
Blake, S. F. 1956. Froehlichia gracilis in Maryland. Rhodora 58:35-38.
Blake, S. T. 1969. A revision of Carpobrotus and Sarcozoma in Australia, genera allied to Mesembryanthemum (Aizoaceae). Contributions from the Queensland Herbarium 7:1-65.
Bock, Jane H. 1968. The water hyacinth in California. Madroño 19:281-283.
Bohrer, Vorsila L. 1978. Plants that have become locally extinct in the Southwest. New Mexico Journal of Science 18:10-19.
Bond, Richard M., and Atwell M. Wallace. 1941. Invasion of a protected area by exotic plants. Scientific Monthly 53:470-472.
Borssum Waalkes, J. van. 1960. Botanical observations on the Krakatau Islands in 1951 and 1952. Annales Bogorienses 4:1-64.
Bradbury, J. Platt, Barbara W. Leyden, M. L. Salgado-Labouriau, W. M. Lewis, Jr., C. Schubert, M. W. Binford, D. G. Frey, D. R. Whitehead, and F. H. Weibezahn. 1981. Late Quaternary environmental history of Lake Valencia, Venezuela. Science 214:1299-1305.
Bradshaw, A. D. 1983. The rconstruction of ecosystems: presidential address to the British Ecological Society. Journal of Applied Ecology 20:1-17.
Braun-Blanquet, J., E. Wikus, R. Sutter, and G. Braun-Blanquet. 1958. Lagunenverlandung und Vegetationsentwicklung an der französischen Mittelmeerküste bei Palavas, ein Sukzessionexperiment. Station Internationale de Géobotanique Méditerraneene et Alpine, Montpellier, Communication 141:9-32.
Brenner, Gilbert J. 1976. Middle Cretaceous floral provinces and early migrations of angiosperms, pp. 23-47. In C. B. Beck, ed., Origin and Early Evolution of Angiosperms . Columbia University Press, New York.
Brokaw, Nicholas V. L. 1985. Gap-phase regeneration in a tropical forest. Ecology 66:682-687.
Brown, R. T., and J. T. Curtis. 1952. The upland conifer-hardwood forests of northern Wisconsin. Ecological Monographs 22:217-234.
Brown, William H., and Donald M. Mathews. 1914. Philippine dipterocarp forests. Philippine Journal of Science, Section A , 9:413-568.
Brumbaugh, Robert W. 1980. Recent geomorphic and vegetal dynamics on Santa Cruz Island, California, pp. 139-158. In Dennis M. Power, ed., The California Islands: Proceedings of a Multidisciplinary Symposium . Santa Barbara Museum of Natural History, Santa Barbara.
Buckley, R. 1981. Alien plants in central Australia. Botanical Journal of the Linnean Society of London 82(4):369-379.
Burdon, J. J., and G. A. Chivers. 1977. Preliminary studies on a native Australian eucalypt forest invaded by exotic pines. Oecologia 31:1-12.
Burkhardt, J. W., and E. W. Tisdale. 1976. Causes of juniper invasion in southern Idaho. Ecology 57:472-484.
Butzer, Karl. 1971. Environment and Archeology: An Ecological Approach to Prehistory . 2d ed. 703 pp. Aldine--Atherton, Chicago.
Butzer, Karl. 1974. Geological and ecological perspectives on the Middle Pleistocene. Quarternary Research 4:136-148.
Byrne, A. Roger. 1980. Man and the variable vulnerability of island life: a study of recent vegetation change in the Bahamas. Atoll Research Bulletin 240:1-200.
Byrne, A. Roger, Colin Busby, and R. F. Heizer. 1979. The Altithermal revisited: pollen evidence from the Leonard Rockshelter. Journal of California and Great Basin Anthropology 1:280-294.
Canham, Charles D., and Orie L. Loucks. 1984. Catastrophic windthrow in the presettlement forests of Wisconsin. Ecology 65:803-809.
Capeheart, B. L., J. J. Ewel, B. R. Sedlik, and R. L. Myers. 1977. Remote sensing survey of Melaleuca. Photogrammetric Engineering and Remote Sensing 43:197-206.
Carlquist, Sherwin. 1974. Island Biology . 660 pp. Columbia University Press, New York.
Carson, Hampton L. 1982. Speciation as a major reorganization of polygenic balance, pp. 411-431. In Claudio Barigozzi, ed., Mechanisms of Speciation . Liss, New York.
Chaloner, W. G., and W. S. Lacey. 1973. The distribution of late Palaeozoic floras, pp. 271-289. In N. F. Hughes, ed., Organisms and continents through time. Palaeontological Association, London, Special Papers .
Chaloner, W. G., and S. V. Meyen. 1973. Carboniferous and Permian floras of the northern continents, pp. 169-186. In A. Hallam, ed., Atlas of Palaeobiogeography . Elsevier, Amsterdam.
Chapman, V. J. 1959. Studies in salt marsh ecology IX. Changes in salt marsh vegetation at Scolt Head Island. Journal of Ecology 47:619-639.
Chapman, V. J. 1976. Coastal vegetation . 2d ed. 292 pp. Pergamon, Oxford.
Christensen, Earl M., and Hyrum B. Johnson. 1964. Presettlement vegetation and vegetational change in three valleys in central Utah. Brigham Young University Science Bulletin, Biology Series 4(4):1-16.
Christensen, Normal L., and Robert K. Peet. 1981. Secondary forest succession on the North Carolina Piedmont, pp. 230-245. In Darrell C. West, Herman H. Shugart, and Daniel B. Botkin, eds. Forest Succession: Concepts and Application . Springer--Verlag, New York.
Clapp, Roger B., and William O. Wirtz II. 1975. The natural history of Lisianski Island, northwestern Hawaiian Islands. Atoll Research Bulletin 186:1-196.
Coblentz, Bruce E. 1978. The effects of feral goats (Capra hircus ) on island ecosystems. Biological Conservation 13:279-286.
Cockayne, Leonard. 1927. New Zealand Plants and Their Story . 3d ed. 271 pp. Government Printer, Wellington.
Coleman, James M. 1966. Recent coastal sedimentation: central Louisiana coast. Louisiana State University, Coastal Studies Institute Technical Report 29:1-73.
Coleman, James M., and Sherwood M. Gagliano. 1964. Cyclic sedimentation in the Mississippi River deltaic plain. Gulf Coast Association of Geological Societies Transactions 14:67-80.
Conard, G. S., and S. R. Radosevich. 1979. Ecological fitness of Senecio vulgaris and Amaranthus retroflexus biotypes susceptible or resistant to atrazine. Journal of Applied Ecology 16:171-177.
Conklin, Harold C. 1957. Hanunoo agriculture: a report on an integral system of shifting cultivation in the Philippines. United Nations, Food and Agriculture Organization Forestry Development Paper 12:1-209.
Cook, Sheila C. A., Claude Lefevre, and Thomas McNeilly. 1972. Competition between metal tolerant and normal plant populations on normal soil. Evolution 26:366-372.
Cooper, William S. 1939. A fourth expedition to Glacier Bay, Alaska. Ecology 20:130-159.
Cooper, William S. 1958. Coastal sand dunes of Oregon and Washington. Geological Society of America Memoir 72:1-169.
Critchfield, William B., and Elbert L. Little, Jr. 1966. Geographic Distribution of the
Pines of the World . 97 pp. USDA Forest Service Miscellaneous Publication 991.
Crocker, Robert L., and B. A. Dickson. 1957. Soil development on the recessional moraines of the Herbert and Mendenhall glaciers, south-eastern Alaska. Journal of Ecology 45:169-185.
Crocker, Robert L., and Jack Major. 1955. Soil development in relation to vegetation and surface age at Glacier Bay, Alaska. Journal of Ecology 43:427-448.
Cross, Aurel T., and Ralph E. Taggart. 1982. Causes of short-term sequential changes in fossil plant assemblages: some considerations based on Miocene flora in the northwest United States. Missouri Botanical Garden Annals 69:676-734.
Cross, J. R. 1975. Biological flora of the British Isles: Rhododendron ponticum. Journal of Ecology 63:345-364.
Cross, J. R. 1981. The establishment of Rhododendron ponticum in the Killarney oak-woods, southwest Ireland. Journal of Ecology 69:807-824.
Cumming, David H. M. 1981. The management of elephants and other large mammals in Zimbabwe, pp. 91-118. In Peter A. Jewell and Sidney Holt, eds., Problems in Management of Locally Abundant Wild Animals . Academic Press, New York.
Curtis, John T. 1959. The Vegetation of Wisconsin: An Ordination of Plant Communities . 657 pp. University of Wisconsin Press, Madison.
Daghlian, Charles P. 1981. A review of the fossil record of monocotyledons. Botanical Review 47:517-555.
Dansereau, Pierre. 1964. Six problems in New Zealand vegetation. Torrey Botanical Club Bulletin 91:114-140.
D'Antoni, H. L., and O. T. Solbrig. 1977. Algarrobas in South American cultures, past and present, pp. 188-199. In Beryl B. Simpson, ed., Mesquite: Its Biology in Two Desert Scrub Ecosystems . Dowden, Hutchinson & Ross, Stroudsburg, Pennsylvania.
Davis, Margaret B. 1965. Phytogeography and palynology of northeastern United States, pp. 377-401. In H. E. Wright, Jr. and David G. Frey, eds. The Quaternary of the United States . Princeton University Press, Princeton.
Davis, Margaret B. 1978. Climatic interpretation of pollen in Quaternary sediments, pp. 35-51. In D. Walker and J. C. Guppy, eds., Biology and Quaternary Environments . Australian Academy of Science, Canberra.
Davis, Margaret B. 1981a . Quaternary history and the stability of forest communities, pp. 132-143. In Darrell C. West, Herman H. Shugart, and Daniel B. Botkin, eds., Forest Succession: Concepts and Application . Springer--Verlag, New York.
Davis, Margaret B. 1981b . Mid-Holocene hemlock decline: evidence for a pathogen or insect outbreak, p. 253. In Robert C. Romans, ed., Geobotany: Proceedings of Geobotany Conference, Bowling Green State University, Ohio . Plenum, New York.
Davis, Margaret B. 1983. Holocene vegetational history of the eastern United States, 2:166-181. In H. E. Wright, Jr., ed., Late Quaternary Environments of the United States . University of Minnesota Press, Minneapolis.
Dean, Walter E., J. Platt Bradbury, Roger Y. Anderson, and Cathy W. Barnosky. 1984. The variability of Holocene climatic change: evidence from varved lake sediments. Science 226:1191-1194.
Degener, Otto, and Isa Degener. 1974. Flotsam and jetsam of Canton Atoll, South Pacific. Phytologia 28:405-418.
Delcourt, Hazel R. 1979. Late Quaternary vegetation history of the eastern Highland Rim and adjacent Cumberland Plateau of Tennessee. Ecological Monographs 49:255-280.
Delcourt, Hazel R., and Paul A. Delcourt. 1984. Ice Age haven for hardwoods. Natural History 93(9):22-28.
Delcourt, Hazel R., Paul A. Delcourt, and E. C. Spiker. 1983. A 12,000-year record of forest history from Cahaba Pond, St. Clair County, Alabama. Ecology 64:874-887.
Delcourt, Paul A. 1980. Goshen Springs: late Quaternary vegetation record for southern Alabama. Ecology 61:371-386.
Delcourt, Paul A., and Hazel R. Delcourt. 1977. The Tunica Hills, Louisiana-Mississippi: Late Glacial locality for spruce and deciduous forest species. Quaternary Research 7:218-237.
Delcourt, Paul A., and Hazel R. Delcourt. 1981. Vegetation maps for eastern North America, 40,000 yrs. B.P. to the present, pp. 123-165. In Robert C. Romans. Geobotany: Proceedings of Geobotany Conference, Bowling Green State University, Ohio . Plenum, New York.
deVlaming, Victor, and Vernon W. Proctor. 1968. Dispersal of aquatic organisms: viability of seeds recovered from the droppings of captive killdeer and mallard ducks. American Journal of Botany 55:20-26.
Dicken, Samuel N., Carl L. Johannessen, and Bill Hanneson. 1961. Some recent physical changes of the Oregon coast. 151 pp. University of Oregon, Department of Geography, Final Report on Office of Naval Research Project NR 388-061.
Dietz, Robert A. 1952. The evolution of a gravel bar. Missouri Botanical Garden Annals 39:249-254.
Dilcher, D. L. 1973. A revision of the Eocene flora of southeastern North America. The Paleobotanist 20:7-18.
Dilcher, D. L., and Peter R. Crane. 1984. In pursuit of the first flower. Natural History 93(3):56-61.
Dilcher, D. L., and Peter R. Crane. 1985. Archaeanthus: an early angiosperm from the Cenomian of the western interior of North America. Missouri Botanical Garden Annals 71:351-383.
Docters van Leeuwen, W. M. 1936. Krakatau, 1883 to 1933. A. Botany. Annales du Jardin Botanique de Buitenzory 46-47:1-506.
Dolan, Robert, Bruce Hayden, and Jeffrey Heywood. 1977. Atlas of environmental dynamics, Assateague Island National Seashore. U.S. Department of Interior, National Park Service, Natural Resource Report 11:1-42.
Dolan, Robert, Bruce Hayden, and Harry Lins. 1980. Barrier islands. American Scientist 68:16-25.
Douglas, J. G., and G. E. Williams. 1982. Southern polar forests: the Early Cretaceous floras of Victoria and their palaeoclimatic significance. Palaeogeography, Palaeoclimatology, Palaeoecology 39:171-185.
Doyle, James A. 1973. Fossil evidence on early evolution of the monocotyledons. Quarterly Review of Biology 48:399-413.
Doyle, James A. 1977. Patterns of evolution in early angiosperms, pp. 501-546. In A. Hallam, ed., Patterns of Evolution as Illustrated by the Fossil Record . Elsevier, Amsterdam.
Doyle, James A., and Leo J. Hickey. 1976. Pollen and leaves from the mid-Cretaceous
Potomac Group and their bearing on early angiosperm evolution, pp. 139-206. In C. B. Beck, ed., Origin and Early Evolution of Angiosperms . Columbia University Press, New York.
Dunkle, Meryl Byron. 1950. Plant ecology of the Channel Islands of California. University of Southern California, Allan Hancock Pacific Expeditions 13:247-340.
Dwire, Kathleen A. 1984. What happens to native grasses when grazing stops? Fremontia 12(2):23-25.
Edgell, Michael C. R. 1984. Trans-hemispheric movements of Holarctic Anatidae: the Eurasian widgeon (Anas penelope L.) in North America. Journal of Biogeography 11:27-39.
Edwards, Kevin J., and Kenneth R. Hirons. 1984. Cereal pollen grains in pre-elm decline deposits: implications for the earliest agriculture in Britain and Ireland. Journal of Archaeological Science 11:71-80.
Eggler, Willis A. 1963. Plant life of Paricutin Volcano, Mexico, eight years after activity ceased. American Midland Naturalist 69:38-68.
Egler, Frank E. 1954. Vegetation science concepts. I. Initial floristic composition, a factor in old field vegetation development. Vegetatio 4:412-417.
Emboden, William A., Jr. 1972. Ginseng: wonder of the world. Terra 10(4):26-31.
Estrada, Alejandro, Rosamond Coates-Estrada, and Carlos Vasquez-Yanes. 1984. Observations on fruiting and dispersers of Cecropia obtusifolia at Los Tuxtlas, Mexico. Biotropica 16:315-318.
Everitt, Benjamin L. 1980. Ecology of saltcedar--a plea for research. Environmental Geology 3:77-84.
Eyde, Richard H., and Storrs L. Olson. 1983. The dead trees of Ilha da Trindade. Bartonia 49:32-51.
Fairbrothers, David E. 1959. Morphological variation of Setaria faberi and S. viridis. Brittonia 11:44-48.
Fassett, Norman C., and Jonathan D. Sauer. 1950. Studies of variation in the weed genus Phytolacca . I. Hybridizing species in northeastern Colombia. Evolution 4:332-333.
Ferguson, David K. 1971. The Miocene flora of Kreuzau, Western Germany. 1. The leaf-remains. Verhandelingen der Koninklijke Nederlandse Akademie van Wetenschappen, Afd. Natuurkunde . Series 2. 60(1):1-297.
Ferren, Wayne R., Jr., John Bleck, and Nancy Vivrette. 1981. Malephora crocea (Aizoaceae) naturalized in California. Madroño 28:80-85.
Finegan, Bryan. 1984. Forest succession. Nature 312:109-114.
Finley, Robert W. 1976. Original vegetation cover of Wisconsin Compiled from U.S. General Land Office Notes . 1:500,000 map, text on reverse. U.S. Department of Agriculture, North Central Forest Experiment Station, St. Paul.
Fisher, C. E. 1977. Mesquite and modern man in southwestern North America, pp. 177-188. In Beryl B. Simpson, ed., Mesquite: Its Biology in Two Desert Scrub Ecosystems . Dowden, Hutchinson & Ross, Stroudsburg, Pennsylvania.
Flaccus, Edward. 1959. Revegetation of landslides in the White Mountains of New Hampshire. Ecology 40:692-703.
Flader, Susan L., ed. 1983. The Great Lakes Forest: An Environmental and Social History . 336 pp. University of Minnesota Press, Minneapolis.
Flenley, John. 1979. The Equatorial Rain Forest: A Geological History . 162 pp. Butterworths, London.
Florin, Rudolf. 1963. The distribution of conifer and taxad genera in time and space. Acta Horti Bergiani 20(4):122-312.
Forcella, Ferank, and Stephen J. Harvey. 1983. Eurasian weed infestation in Western Montana in relation to vegetation and disturbance. Madroño 30:102-109.
Frakes, L. A. 1979. Climates Throughout Geologic Time . 310 pp. Elsevier, Amsterdam.
Franklin, Jerry F., and C. T. Dyrness. 1973. Natural vegetation of Oregon and Washington. U.S. Department of Agriculture, Forest Service General Technical Report PNW-8:1-417.
Frenkel, Robert E. 1977. Ruderal vegetation along some California roadsides. University of California Publications in Geography 20:1-163.
Frenzel, Burkhard. 1968. Grundzuge der pleistozanen Vegetationsgeschichte Nord-Eurasiens . 326 pp. Franz Steiner, Wiesbaden.
Fridriksson, Sturla. 1975. Surtsey . 198 pp. Halsted, New York.
Garwood, Nancy C., D. P. Janos, and N. Brokaw. 1979. Earthquake-caused landslides: a major disturbance to tropical forests. Science 205:997-999.
Germeraad, J. H., C. A. Hopping, and J. Muller. 1968. Palynology of Tertiary sediments from tropical areas. Review of Palaeobotany and Palynology 6:189-348.
Gibbens, Robert P., and Harold F. Heady. 1964. The influence of modern man on the vegetation of Yosemite Valley. University of California, Division of Agricultural Sciences Manual 36:1-44.
Gilbert, Bil, and Terrence Moore. 1985. A tree too tough to kill. Audubon 87:84-97.
Gill, Don. 1973a . Ecological modifications caused by the removal of tree and shrub canopies in the Mackenzie Delta. Arctic 26:95-111.
Gill, Don. 1973b . Modification of northern alluvial habitats by river development. Canadian Geographer 17:138-153.
Gill, Don. 1973c . Native induced secondary plant succession in the Mackenzie River Delta, Northwest Territories, Canada. Polar Record 16:805-808.
Gill, Don. 1974. Influence of waterfowl on the distribution of Beckmannia syzigachne in the Mackenzie River Delta, Northwest Territories. Journal of Biogeography 1:63-69.
Gleadow, Roslyn M. 1982. Invasion by Pittosporum undulatum of forests of central Victoria. II. Dispersal, germination and establishment. Australian Journal of Botany 30:185-198.
Gleadow, Roslyn M., and D. H. Ashton. 1981. Invasion by Pittosporum undulatum of forests of central Victoria. I. Invasion patterns and plant morphology. Australian Journal of Botany 29:705-720.
Godwin, Harry. 1956. The History of the British flora . 384 pp. Cambridge University Press, Cambridge.
Goeden, Richard D., and Donald W. Ricker. 1981. Santa Cruz Island revisited: sequential photography records the causation, rates of progress, and lasting benefits
of successful biological weed control. Proceedings of the Fifth International Symposium on Biological Control of Weeks, Brisbane, Queensland pp. 355-365.
Goeden, Richard D., Charles A. Fleschner, and Donald W. Ricker. 1967. Biological control of prickly pear cacti on Santa Cruz Island, California. Hilgardia 38:579-606.
Good, Ronald L. 1936. On the distribution of the lizard orchid (Himantoglossum hircinum Koch). New Phytologist 35:142-170.
Gould, Stephen Jay. 1984. Toward the vindication of punctuational change, pp. 9-34. In W. A. Berggren and John A. Van Couvering, eds., Catastrophes and Earth History: The New Uniformitarianism . Princeton University Press, Princeton, New Jersey.
Gould, Stephen Jay, and Niles Eldredge. 1977. Punctuated equilibrium: the tempo and mode of evolution reconsidered. Paleobiology 3:115-151.
Graf, William. 1982. Tamarisk and river-channel management. Environmental Management 6:283-296.
Graham, Alan, ed. 1973. Vegetation and Vegetational History of Northern Latin America . 393 pp. Elsevier, Amsterdam.
Graham, Alan, ed. 1976. Studies in neotropical paleobotany. II. The Miocene communities of Veracruz, Mexico. Missouri Botanical Garden Annals 63:787-842.
Graham, Alan, ed. 1982. Diversification beyond the Amazon basin, pp. 78-90. In Ghillean T. Prance, ed., Biological Diversification in the Tropics: Proceedings of the Fifth International Symposium of the Association for Tropical Biology . Columbia University Press, New York.
Gray, M. 1976. Miscellaneous notes on Australian plants. 2. Chrysanthemoides (Compositae). Contributions from Herbarium Australiense 16:1-5.
Griffin, Lisa Carlson, and Ralph M. Rowlett. 1981. A "lost" Viking cereal grain. Journal of Ethnobiology 1:200-207.
Griggs, Tom. 1981. Life histories of vernal pool annual grasses. Fremontia 9(1):14-17.
Grimm, E. C. 1983. Chronology and dynamics of vegetation change in the prairie-woodland region of southern Minnesota. New Phytologist 93:311-350.
Groves, Eric W. 1981. Vascular plant collections from the Tristan da Cunha group of islands. British Museum (Natural History) Bulletin, Botany Series 8(4):333-420.
Grubb, P. J., and E. V. J. Tanner. 1976. The montane forests and soils of Jamaica: a re-assessment. Arnold Arboretum Journal 57:313-368.
Habeck, James R. 1976. Forests, fuels and fire in the Selway-Bitterroot Wilderness, Idaho. Proceedings Tall Timbers Fire Ecology Conference 14:305-353.
Hallam, A. 1977. Biogeographic evidence bearing on the creation of Atlantic seaways in the Jurassic, pp. 23-34. In Robert M. West, ed. Paleontology and plate tectonics with special reference to the history of the Atlantic Ocean. Milwaukee Public Museum Special Publications in Biology and Geology .
Hamilton, Alan. 1974. The history of the vegetation, pp. 188-209. In E. M. Lind and M. E. S. Morrison, eds., East Africa Vegetation . Longman, London.
Hamilton, Alan. 1982. Environmental History of East Africa: A Study of the Quaternary . 328 pp. Academic Press, New York.
Hammen, T. van der. 1974. The Pleistocene changes of vegetation and climate in tropical South America. Journal of Biogeography 1:3-26.
Hammen, T. van der. 1979. History of flora, vegetation and climate in the Colombian Cordillera Oriental during the last five million years, pp. 25-32. In Kai Larsen and Lauritz B. Holm-Nielsen, eds., Tropical Botany . Academic Press, London.
Hammen, T. van der, T. A. Wijmstra, and W. H. Zagwijn. 1971. The floral record of the late Cenozoic of Europe, pp. 391-424. In K. K. Turekian, ed., The Late Cenozoic Glacial Ages . Yale University Press, New Haven.
Harlan, Jack R. 1982a . Relationships between weeds and crops, pp. 91-96. In W. Holzner and Numata, eds., Biology and Ecology of Weeds . W. Junk, The Hague.
Harlan, Jack R. 1982b . Human interference with grass systematics, pp. 37-50. In James R. Estes, Ranald J. Tyrl, and Fere N. Brunken, eds., Grasses and Grasslands: Systematics and Ecology . University of Oklahoma Press, Norman.
Harris, David R. 1966. Recent plant invasions in the arid and semi-arid southwest of the United States. Association of American Geographers Annals 56:408-422.
Hartshorn, Gary S. 1980. Neotropical forest dynamics. Biotropica 12(2) Supplement: 23-30.
Hartshorn, Gary S. 1983. Pentaclethra macroloba (Gavilan), pp. 301-303. In Daniel H. Janzen, ed., Costa Rican Natural History . University of Chicago Press, Chicago.
Harvey, H. Thomas, Howard S. Shellhammer, and Ronald E. Stecker. 1980. Giant sequoia ecology: fire and reproduction. U.S. Department of the Interior, National Park Service Scientific Monograph Series 12:1-182.
Hegi, Gustav. 1954. Matricaria discoidea. Illustrierte Flora von Mittel-Europa 6(2):584-587. Carl Hansen, Munich.
Heinselman, Miron L. 1981. Fire intensity and frequency as factors in the distribution and structure of northern ecosystems, pp. 7-57. In Harold A. Mooney, R. M. Bonnicksen, N. L. Christensen, J. E. Lotan, and W. A. Reiners, coords. Proceedings of the conference: fire regimes and ecosystem properties. U.S. Department of Agriculture, Forest Service General Technical Report . WO-26.
Heiser, Charles B., Jr. 1978a . The totora (Scirpus californicus ) in Ecuador and Peru. Economic Botany 32:222-236.
Heiser, Charles B., Jr. 1978b . Taxonomy of Helianthus and origin of domesticated sunflower. American Society of Agronomy: Sunflower Science and Technology 2:31-53.
Herwitz, Stanley. 1981. Regeneration of selected tropical tree species in Corcovado National Park, Costa Rica. University of California Publications in Geography 24:1-149.
Heslop-Harrison, J. 1953. The North American and Lusitanian elements in the flora of the British Isles, pp. 105-122. In J. E. Lousley, ed., The Changing Flora of Britain . Botanical Society of the British Isles, Oxford.
Heusser, Calvin J. 1964. Some pollen profiles from the Laguna de San Rafael area, Chile, pp. 95-114. In Lucy M. Cranwell, ed., Ancient Pacific Floras . Tenth Pacific Science Congress Series, University of Hawaii, Honolulu.
Heyerdahl, Thor. 1971. The Ra Expeditions . [Translation by Patricia Crampton.] 341 pp. Doubleday, Garden City.
Hickey, Leo J. 1977. Early Creataceous fossil evidence for angiosperm evolution. Botanical Review 43:2-104.
Hickey, Leo J. 1984. Changes in the angiosperm flora across the Cretaceous-Tertiary boundary, pp. 279-313. In W. A. Berggren and John A. Van Couvering, eds., Catastrophes and Earth History: The New Uniformitarianism . Princeton University Press, Princeton, New Jersey.
Hobbs, Elizabeth. 1980. Effects of grazing on the northern populations of Pinus muricata on Santa Cruz Island, California, pp. 159-165. In Dennis M. Power, ed., The California Islands: Proceedings of a Multidisciplinary Symposium . Santa Barbara Museum of Natural History, Santa Barbara.
Hockley, J. 1974. And alligator weed spreads in Australia. Nature 250:704.
Holm, LeRoy G., Donald L. Plucknett, Juan V. Pancho, and James P. Herberger. 1977. The World's Worst Weeds: Distribution and Biology . 609 pp. University of Hawaii Press, Honolulu.
Hopley, David. 1982. The Geomorphology of the Great Barrier Reef: Quaternary Development of Coral Reefs . 452 pp. Wiley-Interscience, New York.
Hos, D. 1975. Preliminary investigation of the palynology of the upper Eocene Werillup Formation, Western Australia. Royal Society of Western Australia Journal 58:1-14.
Hosking, J. R., and P. J. Deighton. 1979. The distribution and control of Opuntia aurantiaca in New South Wales, pp. 195-220. In Seventh Asian-Pacific Weed Science Society Conference, Sydney, Proceedings .
Houston, Douglas B. 1973. Wildfires in northern Yellowstone National Park. Ecology 54:1111-1117.
Howe, Henry F., and Judith Smallwood. 1982. Ecology of seed dispersal. Annual Review of Ecology and Systematics 13:201-228.
Hughes, N. F. 1976. Palaeobiology of Angiosperm Origins: Problems of Mesozoic Seed-Plant Evolution . 242 pp. Cambridge University Press, Cambridge.
Huntley, B., and H. J. B. Birks. 1983. An Atlas of Past and Present Pollen Maps for Europe: 0-13,000 Years Ago . 667 pp. Cambridge University Press, Cambridge.
Ibrahim, Fouad N. 1980. Desertification in Nord-Darfur: Untersuchungen zur Gefährdung des Naturpotentials durch nicht angepasste Landnutzungsmethoden in der Sahelzone der Republik Sudan. Hamburger Geographische Studien 35:1-175.
Iversen, J. 1949. The influence of prehistoric man on vegetation. Danmarks Geologiske Undersøgelse , Series IV. 3(6):1-25.
Iversen, J. 1960. Problems of early Post-Glacial forest development in Denmark. Danmarks Geologiske Undersøgelse , Series IV. 4(3):1-32.
Iversen, J. 1964. Retrogressive vegetational succession in the Post-Glacial. Journal of Ecology 52 Supplement: 59.
Jain, Subodh K., ed. 1976. Vernal pools: their ecology and conservation. University of California, Davis, Institute of Ecology Publication 9:1-93.
Jain, Subodh K., ed. 1983. Genetic characteristics of populations, pp. 240-258. In Harold A. Mooney and M. Godron, eds., Disturbance and Ecosystems: Components of Response . Springer-Verlag, Berlin.
Jain, Subodh K., and A. D. Bradshaw. 1966. Evolutionary divergence among adjacent plant populations. I. The evidence and its theoretical analysis. Heredity 21:407-444.
Jain, Subodh K., and P. S. Martins. 1979. Ecological genetics of the colonizing ability of rose clover (Trifolium hirtum All.). American Journal of Botany 66:361-366.
Jefferson, Timothy H. 1982. Fossil forests from the Lower Cretaceous of Alexander Island, Antarctica. Palaeontology 25:681-708.
Johannessen, Carl L., William A. Davenport, Artimus Millet, and Steven McWilliams. 1971. The vegetation of the Willamette Valley. Association of American Geographers Annals 61:286-302.
Johnson, Donald Lee. 1980. Episodic vegetation stripping, soil erosion, and landscape modification in prehistoric and recent historic time, San Miguel Island, California, pp. 103-121. In Dennis M. Power, ed., The California Islands: Proceedings of a Multidisciplinary Symposium . Santa Barbara Museum of Natural History, Santa Barbara.
Johnson, W. Carter, Robert L. Burgess, and Warren R. Keammerer. 1976. Forest overstory vegetation and environment on the Missouri River floodplain in North Dakota. Ecological Monographs 46:59-84.
Keay, R. W. J. 1959. Lowland vegetation on the 1922 lava flow, Cameroons Mountain. Journal of Ecology 47:25-29.
Keeley, Jon E. 1981. Reproductive cycles and fire regimes, pp. 231-277. In Harold A. Mooney, R. M. Bonnicksen, N. L. Christensen, J. E. Lotan and W. A. Reiners, coords., Proceedings of the conference: fire regimes and ecosystem properties. U.S. Department of Agriculture, Forest Service General Technical Report WO-26.
Keeley, Jon E., and Sterling C. Keeley. 1979. Aponogeton distachyus and Stanleya pinnata. Madroño 26:188-189.
Keeley, Sterling C., Jon E. Keeley, Steve M. Hutchinson, and Albert W. Johnson. 1981. Postfire succession of the herbaceous flora in southern California chapparal. Ecology 62:1608-1621.
Keever, Catherine. 1950. Causes of succession on old fields of the Piedmont, North Carolina. Ecological Monographs 20:229-250.
Kellman, Martin C. 1970. Secondary plant succession in tropical montane Mindanao. Australian National University, Department of Biogeography and Geomorphology Publication BG/2:1-174.
Kellman, Martin C. 1979. Soil enrichment by Neotropical savanna trees. Journal of Ecology 67:565-577.
Kellman, Martin C., and Koyoko Miyanashi. 1982. Forest seedling establishment in Neotropical savannas: observations and experiments in the Mountain Pine Ridge savanna, Belize. Journal of Biogeography 9:193-206.
Kemp, Elizabeth M., and Wayne K. Harris. 1975. The vegetation of Tertiary islands on the Ninetyeast Ridge. Nature 258:303-307.
Kerner von Marilaun, Anton. 1863. Das Pflanzenleben der Donaulaender . 348 pp. Wagner'schen Universität, Innsbruck.
Kerner von Marilaun, Anton. 1896. The Natural History of Plants . [Translation by F. W. Oliver.] 2 vols. Blackie & Son, London.
Kilgore, Bruce M. 1981. Fire in ecosystem distribution and structure: western forests and scrublands, pp. 58-59. In Harold A. Mooney, R. M. Bonnicksen, N. L. Christensen,
J. E. Lotan, and W. A. Reiners, coords., Proceedings of the conference: fire regimes and ecosystem properties. U.S. Department of Agriculture, Forest Service General Technical Report WO-26.
Kilgore, Bruce M., and Dan Taylor. 1979. Fire history of a sequoia-mixed conifer forest. Ecology 60:129-142.
King, James E. 1981. Late Quaternary vegetational history of Illinois. Ecological Monographs 51:43-62.
Kloot, P. M. 1984. The introduced elements of the flora of southern Australia. Journal of Biogeography 11:63-78.
Kral, Friedrich. 1972. Grundlagen zur Entstehung der Waldgesellshaften in Ostalpenraum, pp. 173-186. In Burkhard Frenzel, ed., Vegetationsgeschichte der Alpen: Studien zur Entwicklung von Klima und Vegetation im Postglacial. Berichte der Deutschen Botanischen Gesellschaft .
Lakhanpal, Rajendra N. 1970. Tertiary floras of India and their bearing on the historical geology of the region. Taxon 19:675-694.
Lambert, J. D. H., and J. T. Aranson. 1982. Ramon and Maya ruins: an ecological, not an economic, relation. Science 216:298-299.
Landolt, Elias. 1979. Lemna minuscula Herter (= L. minima Phil.), eine in Europa neue eingebürgerte amerikanische Wasserpflanze. Geobotanisches Institut, Stiftung Rübel, Eidgenössishe Technische Hochschule, Zürich, Berichte 46:86-89.
Lang, Gerald E., and Dennis H. Knight. 1983. Tree growth, mortality, recruitment, and canopy gap formation during a 10-year period in a tropical moist forest. Ecology 64:1075-1080.
Langenheim, Jean H. 1956. Plant succession on a subalpine earthflow in Colorado. Ecology 37:301-317.
Lanner, Ronald M. 1981. The Piñon Pine: A Natural and Cultural History . 208 pp. University of Nevada Press, Reno.
Lawrence, Donald B. 1958. Glaciers and vegetation in southeastern Alaska. American Scientist 46:89-122.
Lawrence, Donald B., and Elizabeth G. Lawrence. 1959. Recent glacier variations in southern South America. American Geographical Society, Southern Chile Expedition Technical Report 1:1-39.
Lawrence, Donald B., R. E. Schoenike, A. Quispel, and G. Bond. 1967. The role of Dryas drummondii in vegetation development following ice recession at Glacier Bay, Alaska. Journal of Ecology 55:793-813.
Leatherman, Stephen P., ed. 1979. Barrier Islands from the Gulf of St. Lawrence to the Gulf of Mexico . 325 pp. Academic Press, New York.
Leopold, Estella B. 1969. Late Cenozoic palynology, pp. 377-438. In Robert H. Tschudy and R. A. Scott, eds., Aspects of Palynology . Wiley-Interscience, New York.
Lesko, G. L., and R. B. Walker. 1969. Effect of sea water on germination in two Pacific atoll beach species. Ecology 50:730-734.
Leyden, Barbara W. 1985. Late Quaternary aridity and Holocene moisture fluctuations in the Lake Valencia Basin, Venezuela, Ecology 66:1279-1295.
Livingstone, Daniel A. 1967. Postglacial vegetation of the Ruwenzori Mountains in equatorial Africa. Ecological Monographs 37:25-52.
Livingstone, Daniel A. 1971. A 22,000-year pollen record from the plateau of Zambia. Limnology and Oceanography 16:349-356.
Livingstone, Daniel A. 1975. Late Quaternary climatic change in Africa. Annual Review of Ecology and Systematics 6:249-280.
Lockmann, Ronald F. 1981. Guarding the Forests of Southern California: Evolving Attitudes Toward Conservation of Watershed, Woodlands , and Wilderness . 184 pp. Arthur H. Clark, Glendale.
Lorence, David H., and Robert W. Sussman. 1986. Exotic species invasion into Mauritius wet forest remnants. Journal of Tropical Ecology 2:147-162.
Loucks, Orie L. 1983. New light on the changing forest, pp. 17-32. In Susan L. Flader, ed., The Great Lakes Forest: An Environmental and Social History . University of Minnesota Press, Minneapolis.
Lüdi, Werner. 1958. Beobachtungen über die Besiedlung von Gletschervorfeldern in den Schweizeralpen. Flora 146:386-407.
Lunan, J. S., and J. R. Habeck. 1973. The effects of fire exclusion on ponderosa pine communities in Glacier National Park, Montana. Canadian Journal of Forest Research 3:574-579.
Luther, Hans. 1961. Veränderungen in der Gefässpflanzenflora der Meeresfelsen von Tvärminne. Societas pro Fauna et Flora Fennica, Acta Botanica Fennica 62:1-100.
Mack, Richard N. 1984. Invaders at home on the range. Natural History 93(2):40-47.
Macnair, M. R. 1981. Tolerance of higher plants to toxic materials, pp. 177-208. In M. A. Bishop and L. M. Cook, eds., Genetic Consequence of Man-Made Change . Academic Press, New York.
Madany, Michael H., and Neil E. West. 1983. Livestock grazing-fire regime interactions within montane forests of Zion National Park, Utah. Ecology 64:661-667.
Maddox, D. M., L. A. Andres, R. D. Hennessey, R. D. Blackburn, and N. R. Spencer. 1971. Insects to control alligatorweed: an invader of aquatic ecosystems in the United States. BioScience 21:985-991.
Mädler, Karl. 1939. Die Pliozäne Flora von Frankfurt am Main. Abhandlungen der Senckenbergischen Naturforschenden Gesellschaft 446:1-202.
Mamay, S. H. 1976. Paleozoic origin of cycads. U.S. Geological Survey Professional Paper 934:1-148.
Mann, John. 1970. Cacti Naturalised in Australia and Their Control . 128 pp. Queensland Department of Lands, Brisbane.
Marchant, C. J. 1967. Evolution in Spartina (Gramineae). I. The history and morphology of the genus in Britain. Botanical Journal of the Linnean Society of London 60:1-24.
Marchant, C. J. 1968. Evolution in Spartina (Gramineae). II. Chromosomes, basic relationships and problems of S . × townsendii Agg. Botanical Journal of the Linnean Society of London 60:381-409.
Marchant, C. J. 1977. Hybrid characteristics in Spartina × neyrautii Fouc., a taxon rediscovered in northern Spain. Botanical Journal of the Linnean Society of London 74:289-296.
Markgraf, Vera. 1972. Die Ausbreitungsgeschichte der Fichte (Picea abies H. Karst) in der Schweiz, pp. 165-172. In Burkhard Frenzel, ed., Vegetationsgeschichte der
Alpen: Studien zur Entwicklung von Klima und Vegetation im Postglacial. Berichte der Deutschen Botanischen Gesellschraft .
Martin, William E. 1959. The vegetation of Island Beach State Park, New Jersey. Ecological Monographs 29:1-46.
Matthews, P., and A. W. Davidson. 1976. Maritime species on roadside verges. Watsonia 11:146-147.
McBride, Joe R., and Diana F. Jacobs. 1980. Land use and fire history in the mountains of southern California, pp. 85-88. In Marvin A. Stokes and John H. Dieterich, coords. Proceedings of the fire history workshop. U.S. Department of Agriculture, Forest Service General Technical Report RM-81.
McCormick, J. Frank, and Robert B. Platt. 1980. Recovery of an Appalachian forest following the chestnut blight, or Catherine Keever--you were right! American Midland Naturalist 104:264-273.
McIntire, William G., and Harley J. Walker. 1964. Tropical cyclones and coastal morphology of Mauritius. Association of American Geographers Annals 54:582-596.
McIntosh, Robert P. 1980. The relationship between succession and the recovery process in ecosystems, pp. 11-62. In John Cairns, Jr., ed., The Recovery Process in Damaged Ecosystems . Ann Arbor Science, Ann Arbor.
McLaughlin, Willard T., and Robert L. Brown. 1942. Controlling coastal sand dunes in the Pacific Northwest. U.S. Department of Agriculture Circular 660:1-46.
Meadley, G. R. W. 1954. Mexican poppy. Western Australia Department of Agriculture Journal , 3d series 3:401-403.
Miller, Charles N., Jr. 1977. Mesozoic conifers. Botanical Review 43:217-280.
Minnich, Richard A. 1977. The geography of fire and big-cone Douglas fir, Coulter pine, and western conifer forests in the east Transverse Ranges, southern California, pp. 443-450. In Harold A. Mooney and Eugene Conrad, coords., Proceedings of the Symposium on Environmental Consequences of Fire and Fuel Management in Mediterranean Ecosystems. U.S. Department of Agriculture, Forest Service General Technical Report WO-3.
Minnich, Richard A. 1980. Vegetation of Santa Cruz and Santa Catalina Islands, pp. 123-137. In Dennis M. Power, ed., The California Islands: Proceedings of a Multidisciplinary Symposium . Santa Barbara Museum of Natural History, Santa Barbara.
Minnich, Richard A. 1982. Grazing, fire, and the management of vegetation on Santa Catalina Island, California, pp. 444-449. In C. Eugene Conrad and Walter C. Oechel, coords., Proceedings of Symposium on Dynamics and Management of Mediterranean-Type Ecosystems. U.S. Department of Agriculture, Forest Service General Technical Report PSW-58.
Minnich, Richard A. In press. The biogeography of fire in the San Bernardino Mountains of California, University of California Publications in Geography .
Mirsky, Arthur, ed., 1966. Soil development and ecological succession in a deglaciated area of Muir Inlet, southeast Alaska. Ohio State University, Institute of Polar Studies Report 20:1-167.
Mitchell, D. S. 1977. Aquatic Weeds in Australian Inland Waters . 189 pp. Australia Department of Environment, Housing and Community Development, Canberra.
Moran, Reid. 1950. Mesembryanthemum in California. Madroño 10:161-163.
Moran, Reid. 1980. Noteworthy collections: Avicennia marina var. resinifera. Madroño 27:143.
Mühlenbach, Viktor. 1979. Contributions to the synanthropic (adventive) flora of the railroads of St. Louis, Missouri, U.S.A. Missouri Botanical Garden Annals 66:1-108.
Mueller-Dombois, Dieter. 1980. The 'ohi'a dieback phenomenon in the Hawaiian rainforest, pp. 153-161. In John Cairns, Jr., ed., The Recovery Process in Damaged Ecosystems . Ann Arbor Science, Ann Arbor.
Mueller-Schneider, Paul. 1977. Verbreitungsbiologie (Diasporologie) der Blutenpflanzen. 2d ed. Gebotanisches Institut, Stiftung Rübel, Eidenössische Technische Hochschule, Zürich, Veroffentlichungen 61:1-226.
Muller, Jan. 1970. Palynological evidence on early differentiation of angiosperms. Cambridge Philosophical Society Biological Reviews 45:417-450.
Muller, Jan. 1981. Fossil pollen records of extant angiosperms. Botanical Review 47:1-142.
Mussett, René. 1952. La dissémination recente d'une graminee, la Spartina townsendii , sur le littoral français. Annales de Geographie 61:53-54.
Myers, R. L. 1983. Site susceptibility to invasion by the exotic tree Melaleuca quinquinervia in southern Florida. Journal of Applied Ecology 20:645-658.
Niklas, Karl J., and E. E. Ginnasi. 1978. Angiosperm paleobiochemistry of the Succor Creek flora (Miocene), Oregon, U.S.A. American Journal of Botany 65:943-952.
Norman, F. I., and M. P. Harris. 1981. Some recent changes in the flora and avifauna of Rabbit Island, Wilson's Promontory, Victoria. Royal Society of Victoria Proceedings 92:209-213.
Numata, Makoro, ed. 1974. The Flora and Vegetation of Japan . 294 pp. Kodasha, Tokyo.
Ogle, Douglas W. 1981. Long-distance dispersal of vascular halophytes: the marshes of Saltville, Virginia. Castanea 46:8-15.
Oka, H. I., and Hiroko Morishima. 1982. Ecological genetics and the evolution of weeds, pp. 73-89. In W. Holzner and M. Numata, eds., Biology and Ecology of Weeds . W. Junk, The Hague.
Osmond, C. B., and J. Monro. 1981. Prickly pear, pp. 194-222. In D. J. Carr and S. G. M. Carr, eds., Plants and Man in Australia . Academic Press, Sydney.
Owen-Smith, Norman. 1981. The white rhino overpopulation problem and a proposed solution, pp. 129-150. In Peter A. Jewell and Sidney Holt, eds., Problems in Management of Locally Abundant Wild Animals . Academic Press, New York.
Ownbey, Marion. 1950. Natural hybridization and amphiploidy in the genus Tragopogon. American Journal of Botany 37:487-499.
Parsons, James J. 1955. The Miskito pine savanna of Nicaragua and Honduras. Association of American Geographers Annals 45:36-63.
Parsons, James J. 1972. Spread of African pasture grasses to the American tropics. Journal of Range Management 25:12-17.
Pennington, Winifred. 1969. The History of British Vegetation . 152 pp. English Universities Press, London.
Penny, John S. 1969. Late Cretaceous and early Tertiary palynology, pp. 331-376. In Robert H. Tschudy and R. A. Scott, eds., Aspects of Palynology . Wiley-Interscience, New York.
Perring, F. H. 1974. Changes in our native vascular plant flora, pp. 7-25. In D. L. Hawksworth, ed., The changing flora and fauna of Britain. Systematics Association Special Volume .
Pestrong, Raymond. 1965. The development of drainage patterns on tidal marshes. Stanford University Publications, Geological Sciences 10(2):1-87.
Philbrick, Ralph N. 1972. The plants of Santa Barbara Island, California. Madroño 21:329-393.
Philbrick, Ralph N. 1980. Distribution and evolution of endemic plants of the California islands, pp. 173-187. In Dennis M. Power, ed., The California Islands: Proceedings of a Multidisciplinary Symposium . Santa Barbara Museum of Natural History, Santa Barbara.
Phillips, Tom L., Russel A. Peppers, and William A. Dimichele. 1985. Stratigraphic and interregional changes in Pennsylvanian coal-swamp vegetation environmental inferences. International Journal of Coal Geology 5:43-109.
Pickard, John. 1984. Exotic plants on Lord Howe Island: distribution in space and time, 1853-1981. Journal of Biogeography 11:181-208.
Pijl, L. van der. 1982. Principles of Dispersal in Higher Plants . 3d ed. 215 pp. Springer-Verlag, New York.
Pillmore, C. L., R. H. Tschudy, C. J. Orth, J. S. Gilmore, and J. D. Knight. 1984. Geologic framework of nonmarine Cretaceous-Tertiary boundary sites, Raton Basin, New Mexico and Colorado. Science 223:1180-1183.
Plumstead, Edna P. 1973. The late Palaeozoic Glossopteris flora, pp. 187-205. In A. Hallam, ed., Atlas of Palaeobiogeography . Elsevier, Amsterdam.
Pohl, Richard W. 1951. The genus Setaria in Iowa. Iowa State College Journal of Science 25:501-508.
Prentice, I. C. 1983. Postglacial climatic change: vegetation dynamics and the pollen record. Progress in Physical Geography 7:273-286.
Price, Edward T. 1960. Root digging in the Appalachians: the geography of botanical drugs. Geographical Review 50:1-20.
Rabinowitz, Philip D., Millard F. Coffin, and David Falvey. 1983. The separation of Madagascar and Africa. Science 220:67-69.
Ralska-Jasiewiczowa, Magdalena. 1983. Isopollen maps for Poland 0-11,000 years. New Phytologist 94:133-175.
Ranwell, D. S. 1967. World Resources of Spartina townsendii (sensu lato) and economic use of Spartina marshland. Journal of Applied Ecology 4:239-256.
Ranwell, D. S. 1972. Ecology of Salt Marshes and Sand Dunes . 258 pp. Chapman & Hall, London.
Raven, Peter H. 1963. A flora of San Clemente Island, California. Aliso 5:289-347
Raven, Peter H., and Daniel I. Axelrod. 1974. Angiosperm biogeography and past continental movements. Missouri Botanical Garden Annals 61:539-673.
Rees, John D. 1979. Effects of the eruption of Paricutin Volcano on landforms, vegetation, and human occupancy, pp. 249-292. In Payson D. Sheets and Donald K. Grayson, eds., Volcanic Activity and Human Ecology . Academic Press, New York.
Retallack, Greg, and David L. Dilcher. 1981a . Arguments for a glossopterid ancestry of angiosperms. Paleobiology 7:54-67.
Retallack, Greg, and David L. Dilcher. 1981b . A coastal hypothesis for the dispersal and rise to dominance of flowering plants, 2:27-77. In Karl J. Niklas, ed., Palaeobotany, Palaeoecology and Evolution . Praeger, New York.
Richards, Paul W. 1964. The Tropical Rain Forest: An Ecological Study . 450 pp. Cambridge University Press, Cambridge.
Ridley, H. N. 1930. The Dispersal of Plants Throughout the World . 744 pp. L. Reeve, Ashford, Kent.
Ritchie, J. C. 1976. The late Quaternary vegetational history of the western interior of Canada. Canadian Journal of Botany 54:1793-1818.
Robbins, W. W. 1940. Alien plants growing without cultivation in California. University of California, Berkeley, Agricultural Experiment Station Bulletin 637:1-128.
Robertson, J. H. 1971. Changes on a sagebrush-grass range in Nevada ungrazed for 30 years. Journal of Range Management 24:397-400.
Robinson, T. W. 1965. Introduction, spread and areal extent of saltcedar (Tamarix ) in the western states. U.S. Geological Survey Professional Paper 491-A:1-12.
Roche, Emile. 1974. Paléobotanique, Paléoclimatologie et derive des continents. Université Louis Pasteur de Strasbourg, Sciences Géologiques Bulletin 27:9-24.
Rodman, James E. 1974. Systematics and evolution of the genus Cakile (Cruciferae). Gray Herbarium of Harvard University Contributions 205:3-146.
Rogers, Garry F. 1982. Then and Now: A Photographic History of Vegetation Change in the Central Great Basin Desert . 152 pp. University of Utah Press, Salt Lake City.
Roose, M. L., and L. D. Gottlieb. 1976. Genetic and biochemical consequences of polyploidy in Tragopogon. Evolution 30:818-830.
Rosengren, Neville. 1981. Dune systems on cuspate forelands, East Gippsland, Victoria. Royal Society of Victoria Proceedings 92:137-147.
Rosser, Effie M. 1955. A new British species of Senecio. Watsonia 3:228-232.
Rundel, Philip W. 1972. Habitat restriction in giant sequoia: the environmental control of grove boundaries. American Midland Naturalist 87:81-99.
Russell, Richard J. 1942. Flotant. Geographical Review 32:74-98.
Russell, Richard J. 1967. River Plains and Sea Coasts . 173 pp. University of California Press, Berkeley.
Sachet, Marie-Helene. 1962. Geography and land ecology of Clipperton Island. Atoll Research Bulletin 86:1-115.
Sagado-Labouriau, Maria Lea. 1982. Climatic change at the Pleistocene-Holocene boundary, pp. 74-77. In Ghillean T. Prance, ed., Biological Diversification in the Tropics: Proceedings of the Fifth International Symposium of the Association for Tropical Biology . Columbia University Press, New York.
Salisbury, Edward J. 1943. The flora of bombed areas. Nature 151:462-466.
Salisbury, Edward J. 1961. Weeds and Aliens . 384 pp. Collins, London.
Sauer, Jonathan D. 1952. A geography of pokeweed. Missouri Botanical Garden Annals 39:113-125.
Sauer, Jonathan D. 1957. Recent migration and evolution of the dioecious amaranths. Evolution 11:11-31.
Sauer, Jonathan D. 1961. Coastal plant geography of Mauritius. Louisiana State University Studies, Coastal Studies Series 5:1-153.
Sauer, Jonathan D. 1962. Effects of recent tropical cyclones on the coastal vegetation of Mauritius. Journal of Ecology 50:275-290.
Sauer, Jonathan D. 1967a . The grain amaranths and their relatives: a revised taxonomic and geographic survey. Missouri Botanical Garden Annals 54:103-137.
Sauer, Jonathan D. 1967b . Plants and Man on the Seychelles Coast: A Study in Historical Biogeography. 132 pp. University of Wisconsin Press, Madison.
Sauer, Jonathan D. 1972. The dioecious amaranths: a new species name and major range extensions. Madroño 21:426-434.
Sauer, Jonathan D. 1982. Cayman Islands seashore vegetation: a study in comparative biogeography. University of California Publications in Geography 25:1-161.
Sawatzky, Harry Leonard. 1971. They Sought a Country: Mennonite Colonization in Mexico . 387 pp. University of California Press, Berkeley.
Schroeder, F. G. 1969. Zur Klassifizierung der Anthropochoren. Vegetatio 16:225-238.
Schuster, Rudolf M. 1976. Plate tectonics and its bearing on the geographical origin and dispersal of angiosperms, pp. 48-138. In C. B. Beck, ed., Origin and Early Evolution of Angiosperms . Columbia University Press, New York.
Scott, Geoffrey A. J. 1978. Grassland development in the Gran Pajonal of eastern Peru. University of Hawaii at Manoa Monographs in Geography 1:1-187.
Sculthorpe, C. D. 1967. The Biology of Aquatic Vascular Plants . 610 pp. Edward Arnold, London.
Searcy, Karen B. 1969. Variation in Cercoarpus in southern California. New Phytologist 68:829-839.
Semple, John C. 1983. Range expansion of Heterotheca camporum (Compositae Astereae) in the southeastern United States. Brittonia 35:140-146.
Shea, S. R. 1979. An ecological approach to the control of jarrah dieback. Western Australia Forest Department, Forest Focus 29:7-18.
Shea, S. R. 1982. Multiple use management in a Mediterranean ecosystem: the jarrah forest, a case study, pp. 49-55. In C. Eugene Conrad and Walter C. Oechel, coords., Proceedings of Symposium on Dynamics and Management of Mediterranean-Type Ecosystems. U.S. Department of Agriculture, Forest Service General Technical Report PSW-58.
Simon, Charles. 1958. Eine Halophytenflora am Oberrhein. Bauhinia 1:144-150.
Smathers, Garrett A., and Dieter Mueller-Dombois. 1974. Invasion and recovery of vegetation after a volcanic eruption in Hawaii. U.S. Department of the Interior, National Park Service Scientific Monograph 5:1-129.
Smit, J., and S. van der Kaars. 1984. Terminal Cretaceous extinctions in the Hell Creek area, Montana: compatible with catastrophic extinctions. Science 223:1177-1179.
Spaulding, W. Geoffrey. 1983. Late Wisconsin macrofossil records of desert vegetation in the American Southwest. Quaternary Research 19:256-264.
Spaulding, W. Geoffrey, Estella B. Leopold, and Thomas R. Van Devender. 1983. Late Wisconsin paleoecology of the American Southwest, 1:259-293. In H. E. Wright, Jr., ed., Late Quaternary Environments of the United States . University of Minnesota Press, Minneapolis.
Spencer, Neal R. 1984. Velvetleaf, Abutilon theophrasti (Malvacea): history and economic impact in the United States. Economic Botany 38:407-416.
Spurr, Stephen H. 1956. Natural restocking of forests following the 1938 hurricane in central New England. Ecology 37:443-451.
Srivastava, Satish K. 1981. Evolution of Upper Cretaceous phytogoprovinces and their pollen flora. Review of Paleobotany and Palynology 35:155-173.
Stanley, Steven M. 1979. Macroevolution: Pattern and Process . 332 pp. Freeman, San Francisco.
Stebbins, G. Ledyard. 1981. Coevolution of grasses and herbivores. Missouri Botanical Garden Annals 68:75-86.
Stevens, Neil E. 1917. Some factors influencing the prevalence of Endothia gyrosa. Bulletin of the Torrey Botanical Club . 44:127-144.
Stewart, Wilson N. 1983. Paleobotany and the Evolution of Plants . 405 pp. Cambridge University Press, Cambridge.
Stoddart, David R. 1962. Three Caribbean atolls: Turneffe Islands, Lighthouse Reef, and Glover's Reef, British Honduras. Atoll Research Bulletin 87:1-151.
Stoddart, David R. 1963. Effects of Hurricane Hattie on the British Honduras reefs and cays, October 30-31, 1961. Atoll Research Bulletin 95:1-142.
Stoddart, David R. 1969. Post-hurricane changes on the British Honduras reefs and cays: resurvey of 1965. Atoll Research Bulletin 131:1-36.
Stoddart, David R. 1980. Mangroves as successional stages, inner reefs of the northern Great Barrier Reef. Journal of Biogeography 7:269-284.
Stoddart, David R. 1981. Biogeography: dispersal and drift. Progress in Physical Geography 5: 575-590.
Stoddart, David R., F. R. Fosberg, and D. I. Spellman. 1982. Cays of the Belize barrier reef and lagoon. Atoll Research Bulletin 256:1-75.
Stoddart, David R., R. F. McLean, T. P. Scoffin, and P. E. Gibbs. 1978. Forty-five years of change on low wooded islands, Great Barrier Reef. Royal Society, London, Philosophical Transcactions Series B 284:63-80.
Stott, Philip. 1981. Historical Plant Geography . 151 pp. Allen & Unwin, London.
Strobel, Gary A., and Gerald N. Lanier. 1981. Dutch elm disease. Scientific American 245(2):56-66.
Stuesy, Tod F., J. K. Foland, John F. Sutter, Roger W. Sanders, and Mario O. Silva. 1984. Botanical and geological significance of potassium-argon dates from the Juan Fernandez Islands. Science 225:49-51.
Suominen, Juha. 1979. The grain immigrant flora of Finland. Acta Botanica Fennica 111:1-108.
Swain, Albert M., and Marjorie J. Winkler. 1983. Environmental history of southern Wisconsin during the Holocene: changes in vegetation, climate, and lake levels. Ecological Society of America Bulletin 64:132-133.
Sweet, Sue. 1981. Plants of the Lanphere-Christensen dunes. Fremontia 9(2):3-8.
Sykes, William R. 1969. The effect of goats on vegetation of the Kermadec Islands. New Zealand Ecological Society Proceedings 16:13-16.
Szafer, Wladyslaw. 1946. The Pliocene flora of Kroscienko in Poland. Polska Akademia Umiejetnosci[*], Krakow, Wydzial Matematyczno-Przyrodniczy Rozprawy, Series 3B 32: 1-162.
Szafer, Wladyslaw. ed. 1966. The Vegetation of Poland . 738 pp. Pergamon, Oxford.
Tallantire, P. A. 1972. Spread of spruce (Picea abies ) in Fennoscandia and possible climatic implications. Nature 236:64-65.
Tanai, Toshimasa. 1972. Tertiary history of vegetation in Japan, pp. 235-255. In Alan Graham, ed., Floristics and Paleofloristics of Asia and Eastern North America . Elsevier, Amsterdam.
Tansley, A. G. 1947. Introduction to Plant Ecology 260 pp. Allen & Unwin, London.
Taylor, H. C. 1978. Capensis, 1:171-229. In M. J. A. Werger, ed., Biogeography and Ecology of Southern Africa . W. Junk, The Hague.
Taylor, Thomas N. 1981. An Introduction to Fossil Plant Biology . 589 pp. McGraw-Hill, New York.
Temple, Stanley A. 1977. Plant-animal mutualism: coevolution with dodo leads to near extinction of plant. Science 197:885-886.
Thellung, Albert. 1912. La flore adventice de Montpellier. Societe Nationale des Sciences Naturelles et Mathématiques, Cherbourg, Memoires 38:57-728.
Thom, Bruce G. 1967. Mangrove ecology and deltaic geomorphology, Tabasco, Mexico. Journal of Ecology 55:301-343.
Thompson, R. S., and J. I. Mead. 1982. Late Quaternary environments and biogeography in the Great Basin. Quaternary Research 17:34-55.
Thorne, Robert F. 1969. The California islands. Missouri Botanical Garden Annals 56:391-408.
Thorne, Robert F. 1978. Plate tectonics and angiosperm distribution. Notes from the Royal Botanical Garden, Edinburgh 36:297-315.
Thorne, Robert F., and Earl W. Lathrop. 1969. A vernal marsh on the Santa Rosa Plateau of Riverside County, California. Aliso 7:85-95.
Tiffney, Bruce H. 1985. The Eocene North Atlantic land bridge: its importance in Tertiary and modern phytogeography of the Northern Hemisphere. Journal of the Arnold Arboretum 66:243-273.
Tisdale, E. W., M. A. Fosberg, and C. E. Poulton. 1963. Vegetation and soil development on a recently glaciated area near Mount Robson, British Columbia. Ecology 47:517-523.
Tralau, Hans. 1973. Some Quaternary plants, pp. 499-503. In A. Hallam, ed., Atlas of Palaeobiogeography . Elsevier, Amsterdam.
Tryon, Rolla M. 1970. Development and evolution of fern floras of oceanic islands. Biotropica 2:76-84.
Tschudy, Robert H. 1984. Palynological evidence for change in continental flora at the Cretaceous-Tertiary boundary, pp. 315-337. In W. A. Berggren and John A. Van Couvering, eds., Catastrophes and Earth History: The New Uniformitarianism . Princeton University Press, Princeton, New Jersey.
Tschudy, Robert H., and R. A. Scott, eds. 1969. Aspects of Palynology . 510 pp. Wiley-Interscience, New York.
Tschudy, Robert H., and Bernardine D. Tschudy. 1986. Extinction and survival of plant life following the Cretaceous/Tertiary boundary event, Western Interior, North America. Geology 14:667-670.
Tsukada, Matsuo. 1982a . Cryptomeria japonica: Glacial refugia and Late-Glacial and Postglacial migration. Ecology 63:1091-1105.
Tsukada, Matsuo. 1982b . Late-Quaternary development of the Fagus forest in the Japanese Archipelago. Japanese Journal of Ecology 32:113-118.
Tsukada, Matsuo. 1982c . Late-Quaternary shift of Fagus distribution. Botanical Magazine, Tokyo 95:203-217.
Tsukada, Matsuo. 1983a . Vegetation and climate during the last glacial maximum in Japan. Quaternary Research 19:212-235.
Tsukada, Matsuo. 1983b . Late-Quaternary spruce decline and rise in Japan and Sakhalin. Botanical Magazine, Tokyo 96:127-133.
Tsukada, Matsuo. 1983c . Man and vegetation in prehistoric Japan. Encyclopedia of Japan 5:92-96. Kodansha, New York.
Tsukada, Matsuo. 1985. Map of vegetation during the last glacial maximum in Japan. Quaternary Research 23:369-381.
Turner, Charles. 1975. The correlation and duration of middle Pleistocene interglacial periods in northwest Europe, pp. 259-308. In Karl Butzer and G. L. Isaac, eds., After the Australopithecines . Mouton, The Hague.
Turner, Raymond M. 1974. Quantitative and historical evidence of vegetation changes along the Upper Gila River, Arizona. U.S. Geological Survey Professional Paper 655-H:1-119.
Turner, Raymond M., and Martin M. Karpiscak. 1980. Recent vegetation changes along the Colorado River between Glen Canyon Dam and Lake Mead, Arizona. U.S. Geological Survey Professional Paper 1132:1-125.
Twisselmann, Ernest C. 1967. A flora of Kern County, California. University of San Francisco, Wasmann Journal of Biology 25:1-395.
Vale, Thomas R. 1974. Sagebrush conversion projects: an element of contemporary environmental change in the western United States. Biological Conservation 6:274-284.
Vale, Thomas R. 1975. Presettlement vegetation in the sagebrush-grass area of the Intermountain West. Journal of Range Management 28:32-36.
Van Cleve, Keith, and Leslie A. Viereck. 1981. Forest succession in relation to nutrient cycling in the boreal forest of Alaska, pp. 185-211. In Darrell C. West, Herman H. Shugart, and Daniel B. Botkin, eds., Forest Succession: Concepts and Application . Springer-Verlag, New York.
Van Devender, Thomas R., and W. Geoffrey Spaulding. 1979. Development of vegetation and climate in the southwestern United States. Science 204:701-710.
Van Devender, Thomas R., Julio L. Betancourt, and Mark Wimberley, 1984. Biogeographic implications of a packrat midden sequence from the Sacramento Mountains, south-central New Mexico. Quaternary Research 22:344-360.
Vankat, John L. 1977. Fire and man in Sequoia National Park. Association of American Geographers Annals 67:17-27.
Varshney, C. L., and J. Rzoska, eds. 1976. Aquatic Weeds in Southeast Asia . 396 pp. W. Junk, The Hague.
Vartianen, Terttu. 1980. Succession of island vegetation in the land uplift area of the northernmost Gulf of Bothnia, Finland. Acta Botanica Fennica 115:1-105.
Vasek, Frank C. 1980. Creosote bush: long-lived clones in the Mojave Desert. American Journal of Botany 67:246-255.
Vaughan, R. E., and P. O. Wiehe. 1937. Studies on the vegetation of Mauritius. I. A preliminary survey of the plant communities. Journal of Ecology 25:298-343.
Vaughan, R. E., and P. O. Wiehe. 1941. Studies on the vegetation of Mauritius. III. The structure and development of the upland climax forest. Journal of Ecology 29:127-160.
Veblen, Thomas T., and David H. Ashton. 1978. Catastrophic influences on the vegetation of the Valdivian Andes, Chile. Vegetatio 36:149-167.
Veblen, Thomas T., Claudio Donoso Z., Federico M. Schlegel, and Bernardo Escobar R. 1981. Forest dynamics in south-central Chile. Journal of Biogeography 8:211-247.
Vesper, Stephen J., and Ronald L. Stuckey. 1977. The return of aquatic vascular plants into the Great Lakes region after Late-Wisconsin glaciation, pp. 283-299. In Robert C. Romans, ed., Geobotany: Proceedings of Geobotany Conference, Bowling Green State University, Ohio . Plenum, New York.
Vietmeyer, Noel D. 1975. The beautiful blue devil. Natural History 84(11):65-73.
Vivrette, Nancy J., and Cornelius H. Muller. 1977. Mechanism of invasion and dominance of coastal grassland by Mesembryanthemum crystallinum. Ecological Monographs 47:301-318.
Vogl, Richard J. 1967. Fire adaptations of some southern California plants. Tall Timbers Fire Ecology Conference 7:79-110.
Vogl, Richard J. 1980. The ecological factors that produce perturbation-dependent ecosystems, pp. 63-94. In John Cairns, Jr., ed., The Recovery Process in Damaged Ecosystems . Ann Arbor Science, Ann Arbor.
Vogts, Marie. 1982. South Africa's Proteaceae: Know Them and Grow Them . 240 pp. C. Struik, Cape Town.
Wace, N. M., and M. W. Holdgate. 1976. Man and nature in the Tristan da Cunha Islands. International Union for Conservation of Nature and Natural Resources, Morges, Switzerland, Monograph 6:1-114.
Walker, James W., and James A. Doyle. 1975. The bases of angiosperm phylogeny: palynology. Missouri Botanical Garden Annals 62:664-723.
Walker, James W., Gilbert J. Brenner, and Audrey G. Walker. 1983. Winteraceous pollen in the Lower Cretaceous of Israel: early evidence of a Magnolialean angiosperm family. Science 220:1273-1275.
Warme, John E. 1971. Paleoecological aspects of a modern coastal lagoon. University of California Publications in Geological Sciences 87:1-131.
Watts, W. A. 1978. Plant macrofossils and Quaternary palaeoecology, pp. 53-67. In D. Walker and J. C. Guppy, eds., Biology and Quaternary Environments . Australian Academy of Science, Canberra.
Watts, W. A. 1979. Late Quaternary vegetation of central Appalachia and the New Jersey coastal plain. Ecological Monographs 49:427-469.
Watts, W. A. 1983. Vegetational history of the eastern United States 25,000 to 10,000 years ago, 2:294-310. In H. E. Wright, Jr., ed., Late Quaternary Environments of the United States . University of Minnesota Press, Minneapolis.
Wells, Philip V. 1977. Post-Glacial origin of the present Chihuahuan Desert less than 11,500 years ago, pp. 67-83. In Roland H. Wauer and David H. Riskind, eds., Transactions of the symposium on the biological resources of the Chihuahuan
Desert Region. U.S. Department of the Interior, National Park Service Transactions and Proceedings Series .
Wells, Philip V. 1983. Paleobiogeography of montane islands in the Great Basin since the last glaciopluvial. Ecological Monographs 53:341-382.
Wells, Philip V., and J. H. Hunziker. 1976. Origin of the creosote-bush (Larrea ) deserts of southwestern North America. Missouri Botanical Garden Annals 63:843-861.
Wells, Philip V., and Deborah Woodcock. 1985. Full-Glacial vegetation of Death Valley, California: juniper woodland opening to Yucca semidesert. Madroño 32:11-23.
West, Neil E., ed. 1983. Temperate deserts and semideserts. Ecosystems of the World 5:1-522. Elsevier, Amsterdam.
West, R. G. 1970. Pleistocene history of the British flora, pp. 1-11. In D. Walker and R. G. West, eds., Studies in the Vegetational History of the British Isles: Essays in Honor of Harry Godwin . Cambridge University Press, Cambridge.
West, Robert C., Norbert P. Psuty, and Bruce G. Thom. 1969. The Tabasco lowlands of southeastern Mexico. Louisiana State University, Coastal Studies Institute Technical Report 70:1-193.
Wester, Lyndon. 1975. Changing Patterns of Vegetation on the West Side and South Side of the San Joaquin Valley during Historic Time . 186 pp. Ph.D. Thesis, University of California, Los Angeles.
Wester, Lyndon. 1981. Composition of native grasslands in the San Joaquin Valley, California. Madroño 28:231-241.
Wester, Lyndon. 1982. Introduction and spread of mangroves in the Hawaiian Islands. Association of Pacific Coast Geographers Yearbook 43:125-137.
Westman, Walter E. 1983. Island biogeography: studies on the xeric shrublands of the inner Channel Islands, California. Journal of Biogeography 10:97-118.
Westman, Walter E., F. D. Panetta, and T. D. Stanley. 1975. Ecological studies on reproduction and establishment of the woody weed, grounsel bush (Baccharis halimifolia L.: Asteraceae). Australian Journal of Agricultural Research 26:855-870.
Whitmore, J. L. 1983. Ochroma lagopus (Balsa), pp. 281-283. In Daniel H. Janzen, ed., Costa Rican Natural History . University of Chicago Press, Chicago.
Whitmore, T. C. 1982. On pattern and process in forests, pp. 45-59. In E. I. Newman, ed., The plant community as a working mechanism. British Ecological Society Special Publication .
Whittaker, R. J., K. Richards, H. Wiriadinata, and J. R. Flenley. 1984. Krakatau 1883 to 1983: biogeographical assessment. Progress in Physical Geography 8:61-81.
Widrlechner, Mark P. 1983. Historical and phenological observations on the spread of Chaenorrhinum minus across North America. Canadian Journal of Botany 61:179-187.
Williams, Garnett P. 1978. The case of the shrinking channels--the North Platte and Platte rivers in Nebraska. U.S. Geological Survey Circular 781:1-48.
Williams, Garnett P., and M. Gordon Wolman. 1984. Downstream effects of dams on alluvial rivers. U.S. Geological Survey Professional Paper 1286:1-83.
Williamson, G. B. 1983. Crysophila guagara (Guagra, Fan Palm), pp. 224-225. In
Daniel H. Janzen, ed., Costa Rican Natural History . University of Chicago Press, Chicago.
Wolfe, Jack A. 1972. An interpretation of Alaskan Tertiary floras, pp. 201-233. In Alan Graham, ed., Floristics and Paleofloristics of Asia and Eastern North America . Elsevier, Amsterdam.
Wolfe, Jack A. 1973. Fossil forms of Amentiferae. Brittonia 25:334-355.
Wolfe, Jack A., and Toshimasa Tanai. 1980. The Miocene Seldovia Point Flora from the Kenai Group, Alaska . U.S. Geological Survey Paper 1105.
Wolfe, Jack A., and Garland R. Upchurch, Jr., In press. Vegetational, climatic, and floral changes at the Cretaceous-Tertiary boundary. Nature .
Wright, H. E., Jr. 1971. Late Quaternary vegetational history of North America, pp. 425-464. In K. K. Turekian, ed., The Late Cenozoic Glacial Ages . Yale University Press, New Haven.
Wright, H. E., Jr. 1976. The dynamic nature of Holocene vegetation: a problem in paleoclimatology, biogeography, and stratigraphic nomenclature. Quaternary Research 6:581-596.
Wulff, E. V. 1943. An Introduction to Historical Plant Geography . [Translation by Elizabeth Brissenden.] 223 pp. Chronica Botanica Company, Waltham, Massachusetts.
Yensen, D. L. 1981. The 1900 invasion of alien plants into southern Idaho. Great Basin Naturalist 41:176-183.
Yeo, Richard R., and W. B. McHenry. 1977. Hydrilla , a new noxious aquatic weed in California. California Agriculture 31(10):4-5.
Young, James A., and Jerry D. Budy. 1979. Historical use of Nevada's pinyon-juniper woodlands. Journal of Forest History 23:112-121.
Young, James A., R. A. Evans, and J. Major. 1972. Alien plants in the Great Basin. Journal of Range Management 25:194-201.
Young, James A., R. A. Evans, and P. T. Tueller. 1976. Great Basin plant communities, pp. 187-215. In Robert Elston, ed., Holocene environmental change in the Great Basin. Nevada Archeological Survey Research Paper .
Zedler, Paul H. 1977. Life history attributes of plants and the fire cycle: a case study in chaparral dominated by Cupressus forbesii , pp. 451-458. In Harold A. Mooney and C. Eugene Conrad, coords., Proceedings of the symposium on environmental consequences of fire and fuel management in Mediterranean ecosystems. U.S. Department of Agriculture, Forest Service General Technical Report WO-3.
Zimmerman, R. C. 1969. Plant ecology of an arid basin: Tres Alamos-Reddington area, southeastern Arizona. U.S. Geological Survey Professional Paper 485-D:1-51.
Zumph, F., and O. Rebmann. 1932. Ökologische Studien in Sperenberger Salzgebiet. Zeitschrift für Morphologie und Ökologie der Tiere 24:768-801.
Index To Genera
A
Abies,54 , 64 -65, 69 , 73 , 74 , 126 , 129 -131, 152 , 153 , 157 , 160 , 166 , 169 , 181 , 183 , 189 , 190 -191, 194 , 239
Abutilon, 100 , 104 , 220 , 241
Acacia, 30 , 86 , 89 , 120 , 121 , 123 , 133 , 136 , 167 , 190 , 193 , 213 , 243
Acalypha, 175 , 244
Acanthospermum,120 , 246
Acer,49 , 52 , 69 , 79 -81, 106 , 126 -127, 134 -135, 155 , 160 , 162 , 184 , 187 , 189 , 190 -191, 244
Achillea, 53 , 246
Actinidia, 189 , 241
Adansonia, 129 , 241
Aegiciras, 16 , 242
Aesculus, 120 , 244
Agave, 167 , 247
Agropyron, 85 , 100 , 247
Agrostis, 60 , 220 , 247
Ailanthus, 189 , 190 , 244
Albizzia, 190 , 243
Alhagi, 136 , 243
Allium, 60 , 247
Alnus,48 , 52 , 53 , 57 , 58 , 60 , 62 , 63 , 69 , 152 -153, 154 -155, 157 , 160 , 163 , 175 , 185 , 187 , 189 , 190 -192, 194 , 240
Alternanthera,44 -45, 175 , 241
Amaranthus, 26 , 28 , 47 , 49 , 50 , 99 , 101 -103, 174 -175, 224 -226, 241
Ambrosia, 28 , 49 , 50 , 100 , 105 , 175 , 246
Ammophila, 26 -27, 29 , 247
Anacampsis, 98 , 247
Anacardium, 24 -25, 244
Anaphalis, 68 , 246
Andropogon, 27 , 67 , 105 , 108 , 247
Anemone, 67 , 240
Annona, 190 , 240
Anthoxanthum,84 , 220 , 247
Aponogeton, 42 , 246
Araucaria, 202 , 211 , 233 , 239
Arbutus, 190 , 192 , 242
Archaeosperma,198 , 239
Arctostaphylos,48 , 56 -57, 131 , 167 , 190 , 193 , 242
Argemone, 20 , 52 , 240
Aristida, 120 , 247
Artemisia, 36 , 48 , 52 , 54 , 110 , 112 -113, 128 , 165 -168, 171 , 246
Arthrocnemum,37 , 38 -39, 241
Arthrotaxis,203 , 239
Asimina, 189 , 240
Aster,5 , 36 , 38 , 98 , 105 , 246
Asystsasia, 66 , 246
Atriplex, 40 , 41 , 85 , 110 -112, 166 -167, 171 , 241
Avena,83 , 115 , 220 , 221 , 222 , 247
Avicennia, 11 , 12 , 13 , 15 , 16 , 176 , 210 , 245
B
Baccharis, 50 , 51 -52, 59 , 65 , 76 , 107 -108, 116 , 136 , 246
Banksia, 133 , 244
Barringtonia,24 , 118 , 210 , 242
Bassia, 40 , 85 , 241
Batis,12 , 241
Beckmannia, 47 -48, 247
Berchemia, 189 , 244
Betula, 53 , 58 , 60 , 63 , 68 , 69 , 79 -81, 126 , 128 , 148 , 149 , 151 , 152 , 153 , 154 -155, 157 , 160 , 162 , 176 , 180 -181, 187 , 189 , 190 , 240
Bidens, 63 , 246
Blackstonia, 98 , 245
Borreria, 66 , 246
Borrichia, 18 -19, 246
Bothriochloa,226 , 247
Brachystegia,121 , 243
Brahea, 190 , 247
Brassica, 103 , 242
Brickellia, 136 , 246
Bromus, 54 , 83 -84, 85 , 87 , 112 -113, 115 , 125 , 220 , 247
Brosimum, 95 , 175 , 242
Bruguiera, 16 , 243
Buddleia, 55 , 65 , 67 , 245
Bursera, 18 -19, 175 , 190 , 193 , 244
Buxus, 181 , 244
Byrsonima, 76 , 108 , 175 , 245
C
Caesalpinia,210 , 243
Cakile,4 , 26 , 28 , 31 -35, 60 , 72 , 233 , 242
Callitriche, 42 , 245
Calluna, 60 , 242
Calophyllum, 21 , 22 , 242
Calvaria, 140 , 242
Calystegia, 114 , 116 , 245
Canavalia, 2 , 20 , 21 , 25 , 70 , 243
Capparis, 120 , 242
Carduus, 84 , 246
Carex,36 , 72 , 188 , 247
Carica, 70 , 242
Carpinus, 152 , 153 , 154 -155, 160 , 180 , 189 , 190 , 240
Carpobrotus, 30 -31, 241
Carya, 106 , 157 , 160 , 162 , 181 , 189 , 190 -191, 245
Castanea, 68 , 86 , 89 -91, 161 , 162 , 163 , 177 , 189 , 190 , 240
Castanopsis,190 , 240
Casuarina, 20 -21, 22 , 24 , 71 , 72 , 76 , 118 , 123 , 211 , 241
Cathaya, 189 , 239
Ceanothus, 65 , 72 , 131 , 184 , 190 , 192 -193, 244
Cecropia, 78 -79, 107 -108, 242
Cedrela, 190 , 192 -193, 244
Cedrus, 189 , 202 , 239
Ceiba,66 , 78 -79, 81 , 213 , 241
Celtis, 49 , 135 , 189 , 190 , 242
Cenchrus, 19 , 120 , 247
Centaurea, 148 , 155 , 246
Cephalotaxus,189 , 240
Ceratoides, 110 , 112 , 241
Ceratonia, 82 , 243
Ceratophyllum,156 , 188 , 240
Cercidiphyllum,190 , 240
Cercidium, 168 , 243
Cercocarpus,166 -167, 184 , 190 , 192 -193, 230 , 243
Cereus, 168 , 241
Ceriops, 16 , 243
Chaenactis, 54 , 246
Chaenorrhinum,96 , 245
Chamaecyparis,189 , 190 , 240
Chamomilla, 97 -98, 246
Chenopodium, 40 , 66 , 118 , 148 , 155 , 241
Chilopsis, 50 , 190 , 193 , 246
Choisya, 169 , 244
Chrysanthemoides,30 , 246
Chrysobalanus,24 , 243
Chrysophila, 78 , 247
Chrysothamnus,110 , 168 , 246
Chusquea, 55 , 58 -59, 247
Cinchona, 86 -87, 246
Cinnamomum, 88 , 189 , 240
Cirsium, 85 , 246
Cladium, 151 , 156 , 247
Clethra, 65 , 242
Coccoloba, 18 -19, 107 , 241
Cocculus, 189 , 240
Cochlearia, 60 , 242
Cocos,18 , 20 , 21 , 22 , 24 , 210 , 247
Coleogyne, 166 , 243
Colubrina, 190 , 193 , 244
Commiphora, 121 , 244
Conocarpus, 13 , 16 , 18 -19, 243
Conyza, 65 , 105 , 246
Cordia, 18 -19, 245
Coreopsis, 116 , 246
Coriaria, 55 , 66 , 72 , 244
Cornus, 60 , 189 , 227 , 244
Corylopsis, 189 , 240
Corylus, 151 -152, 155 , 177 , 180 -181, 183 , 189 , 240
Cotula, 40 , 42 , 246
Crassula, 42 , 243
Crataegus, 190 , 243
Crotalaria, 65 , 243
Croton, 28 , 244
Cryptomeria,177 -178, 203 , 239
Cunninghamiana,189 , 203 , 239
Cupressus, 131 -132, 190 , 193 , 240
Curatella, 175 , 241
Cynodon, 65 , 247
Cyperus, 20 -21, 46 , 247
Cyrtandra, 70 , 245
Cytisus, 29 , 243
D
Dacrydium, 202 , 239
Dalea,53 , 65 , 243
Datura, 100 , 245
Daucus, 148 , 245
Dendromecon,190 , 240
Dendrosenecio,178 -179, 246
Dendroseris,223 , 246
Deschampsia, 42 , 84 , 247
Desmodium, 22 , 65 , 243
Digitalis, 55 , 245
Dioclea,3 , 243
Diospyros, 189 , 190 , 242
Distichlis, 85 , 247
Downingia, 42 , 246
Drimys, 58 -59, 185 , 240
Dryas,56 -57, 148 , 149 , 157 , 160 , 163 , 243
Dubautia, 67 -68, 246
Dudleya, 116 , 243
Dysoxylum, 71 , 244
E
Eichhornia, 44 -46, 247
Elatine, 42 , 241
Eleagnus, 51 , 136 , 244
Eleocharis, 40 , 42 , 47 , 247
Eleutheranthera,71 , 246
Elodea, 43 , 246
Elymus, 29 , 60 , 72 , 84 , 94 -95, 110 -112, 247
Emilia, 66 , 246
Empetrum, 59 , 60 -61, 69 , 242
Engelhardtia,189 , 245
Entada,3 , 243
Ephedra, 148 , 166 -167, 239
Epilobium, 53 , 54 , 57 , 60 , 68 , 75 , 98 , 151 , 243
Eragrostis, 118 , 120 , 247
Eremocarpus,115 , 244
Eriogonum, 117 , 241
Erophorum, 72 , 153 , 247
Erodium, 83 -84, 85 , 97 , 115 , 245
Erythea, 193 , 247
Eucalyptus, 89 , 133 , 211 , 243
Eucommia, 189 , 190 , 240
Eucryphia, 54 , 243
Eupatorium, 65 , 246
Euphorbia, 18 , 26 , 244
Euryale, 188 , 240
F
Fagus,68 , 69 , 79 , 81 , 85 , 126 -127, 146 , 150 , 153 , 154 -156, 159 , 161 , 162 , 177 , 181 , 183 , 189 , 190 , 194 , 240
Fallugia, 166 , 243
Festuca, 54 , 60 , 85 , 220 , 247
Ficus,66 , 70 -71, 141 , 242
Foeniculum, 115 , 245
Fouquieria, 168 , 171 , 190 , 242
Fragaria, 67 , 243
Franseria, 166 -168, 169 , 246
Fraxinus, 134 -135, 152 , 160 , 166 , 180 , 189 , 190 -191, 193 , 245
Fremontia, 190 , 193 , 241
Froehlichia, 95 -96, 241
Fuchsia, 55 , 59 , 243
G
Galium, 39 , 60 , 246
Garrya, 184 , 190 , 244
Gaultheria, 65 , 242
Gentiana, 185 , 245
Ginko, 189 , 190 , 200 , 231 , 234 , 239
Glaux,39 , 183 , 242
Glossopteris,199 -201, 239
Glyptostrobus,189 , 190 -191, 203 , 239
Gossypium, 229 , 241
Guaiacum, 93 , 244
Gundlachia, 107 , 246
Gunnera, 55 , 59 , 185 , 243
Gutierrezia,168 , 246
H
Hagenia, 179 , 243
Halimione, 38 -39, 241
Halogeton, 96 , 112 , 241
Haplopappus,167 , 246
Harungana, 66 , 241
Hedera, 181 , 245
Heydysarum, 48 , 56 , 243
Helianthemum,148 , 242
Helianthus, 95 , 246
Hemizonia, 115 , 246
Heracleum, 94 , 245
Heteromeles, 74 , 117 , 190 , 192 , 243
Heteropogon,192 , 247
Heterotheca, 28 , 95 , 246
Himantoglossum,5 , 247
Hippophaë,62 , 148 , 244
Holcus, 55 , 84 , 87 , 247
Homalanthus,108 , 244
Honkenya, 26 , 241
Hudsonia, 27 , 242
Hydrangea, 69 , 243
Hydrilla, 43 -44, 246
Hymenocallis,18 , 24 , 247
Hymenodictyon,66 , 246
Hyoscyamus, 100 , 245
Hypochoeris, 55 , 115 , 246
I
Ilex,85 -86, 181 , 185 , 189 , 244
Imperata, 70 , 107 -108, 109 , 119 , 247
Intsia, 93 , 243
Ipomoea, 18 -19, 20 , 21 , 25 , 28 , 70 , 118 -119, 245
Iris, 223 , 225 , 247
Iva,100 , 246
J
Juanea, 222 -223, 247
Juglans, 52 , 177 , 189 , 190 , 194 , 245
Juncus, 38 -39, 42 , 60 , 247
Juniperus, 27 , 60 -61, 110 -111, 113 , 148 , 149 , 165 -167, 168 -169, 171 , 173 , 190 , 240
K
Keteleeria, 189 , 190 , 239
Koelreuteria,189 , 244
Koenigia, 148 , 241
L
Lactoris, 222 -223, 240
Lagenostoma,195 , 239
Laguncularia,12 , 13 , 16 , 243
Lantana, 70 , 107 , 108 , 120 , 245
Larix,58 , 69 , 148 , 157 , 160 , 189 , 190 , 202 , 239
Larrea, 84 , 125 , 167 , 169 , 171 , 172 , 244
Lathyrus, 26 , 60 , 243
Lemna,42 , 44 , 60 , 247
Lepidopteris,200 , 239
Leptospermum,211 , 243
Leucaena, 106 , 243
Libocedrus, 184 , 190 , 240
Lilaea, 42 , 246
Limnanthes, 42 , 245
Limonium, 38 -39, 241
Lindera, 189 , 190 , 240
Liquidambar,186 , 189 , 190 , 194 , 240
Liriodendron,106 , 189 , 190 , 240
Lithocarpus,190 , 192 -193, 240
Lobelia, 178 , 246
Lolium, 97 , 247
Lonicera, 227 , 246
Lupinus, 53 , 66 , 243
Lycium, 166 , 245
Lyginopteris,195 , 239
Lyonothamnus,114 , 180 , 192 -194, 231 , 243
Lysiloma, 190 , 193 , 243
Lythrum, 42 , 67 , 243
M
Macaranga, 70 -71, 108 , 244
Magnolia, 184 , 189 , 190 , 206 , 210 , 234 , 240
Mallotus, 108 , 244
Mamillaria, 171 , 241
Matricaria, 60 , 97 -98, 246
Medicago, 83 , 97 , 115 , 243
Melaleuca, 76 , 77 , 119 , 243
Melilotus, 65 , 85 , 243
Mesembryanthemum,30 -31, 114 , 116 , 241
Metasequoia,190 -191, 203 , 229
Metopium, 107 , 244
Metrosideros,67 -68, 72 , 243
Mimosa, 52 , 243
Mimulus, 116 -117, 245
Miscanthus, 68 , 247
Monerma, 40 , 247
Morinda, 21 , 22 , 24 , 246
Morus, 189 , 242
Mucuna,3 , 243
Myosurus, 42 , 240
Myrica, 27 , 240
Myriophyllum,59 , 149 , 161 , 243
Myrtus, 82 , 243
N
Najas, 118 , 156 , 188 , 246
Nama, 118 , 245
Neonauclea, 70 -71, 246
Nicotiana, 21 , 52 , 117 , 226 , 245
Nolina, 167 , 247
Nothofagus, 54 -55, 58 -59, 207 , 211 , 213 , 240
Nuphar, 161 , 240
Nymphaea, 161 , 240
Nypa, 210 , 247
Nyssa, 189 , 190 , 244
O
Ochroma, 78 -79, 241
Oenothera, 28 , 68 , 243
Olea, 179 , 245
Ononis, 98 , 243
Opuntia, 115 -117, 121 -124, 125 , 166 -167, 168 , 241
Orchis,5 , 247
Orcuttia, 42 , 247
Orothamnus , 132 , 244
Ostrya , 160 , 189 , 190 , 240
P
Panax , 92 -93, 245
Panicum , 49 , 50 , 247
Papaver , 103 , 240
Parapholis , 40 , 247
Parkinsonia , 168 , 243
Paspalum , 66 , 247
Passiflora , 107 , 242
Paulownia , 189 , 190 , 245
Pennisetum , 65 , 68 , 247
Pentaclethra , 78 , 243
Pernettya , 59 , 242
Persea , 55 , 189 , 190 , 192 -193, 240
Phormium , 87 , 247
Phragmites , 5 , 12 , 38 , 40 , 46 , 47 , 60 , 136 , 247
Physalis , 28 , 245
Phytolacca , 6 , 49 -50, 65 , 99 , 107 , 226 , 241
Picea , 48 , 54 , 56 -57, 58 , 60 , 63 , 73 , 126 -127, 129 , 148 , 150 -151, 152 , 153 , 154 , 157 , 159 , 160 , 161 , 163 , 166 -167, 169 , 181 , 183 , 189 , 190 , 191 , 194 , 239
Pinus , 58 , 60 , 62 , 63 , 64 -65, 69 , 73 , 74 , 75 , 76 , 80 -81, 82 , 85 , 89 , 105 -106, 110 -111, 113 , 115 -116, 126 -127, 129 -131, 148 , 149 -152, 153 , 154 , 157 , 159 -160, 161 , 163 , 165 -171, 173 , 176 , 178 , 180 -181, 184 , 187 , 189 , 190 -191, 193 , 194 , 202 , 239
Pithecellobium , 190 , 193 , 243
Pittosporum , 86 , 243
Plantago , 39 , 97 , 98 , 128 , 148 , 155 , 164 , 185 , 220 , 245
Platanus , 49 , 50 , 52 , 189 , 190 -191, 206 , 210 , 240
Pluchea , 136 , 246
Plumeria , 24 , 245
Poa , 54 , 55 , 60 , 68 , 97 , 110 , 128 , 247
Podocarpus , 58 -59, 175 , 179 , 185 , 202 , 210 -211, 233 , 234 , 239
Polygonum , 39 , 47 , 72 , 97 , 183 , 185 , 188 , 241
Pongamia , 21 , 22 , 243
Populus , 48 , 49 , 50 , 51 -52, 53 , 54 , 56 , 57 , 60 , 63 , 68 , 80 -81, 126 , 128 , 129 , 131 , 134 -135, 136 , 148 , 149 , 151 , 152 , 157 , 160 , 184 , 187 , 189 , 190 -191, 194 , 242
Portulaca , 19 , 21 , 118 , 241
Potamogeton , 42 , 47 , 59 , 118 , 161 , 246
Prosopis , 125 , 136 , 169 , 190 , 193 , 243
Protium , 95 , 244
Prunella , 55 , 226 , 245
Prunus , 27 , 68 -69, 74 , 80 -81, 86 , 93 , 131 , 166 , 168 , 184 , 189 , 190 , 193 -194, 243
Pseudolarix , 189 , 239
Pseudotsuga , 129 -131, 166 -167, 168 -169, 184 , 190 -191, 193 , 239
Psidium , 66 , 88 , 108 , 120 , 243
Pterocarya , 181 , 187 , 189 , 190 -191, 245
Puccinellia , 60 , 247
Purshia , 166 , 168 , 243
Pyrus , 206 , 243
Q
Quercus , 52 , 63 , 64 -65, 68 , 69 , 74 , 82 , 85 , 93 , 105 -106, 114 -115, 117 , 127 -128, 131 , 134 -135, 140 , 150 , 152 , 154 -155, 157 , 159 , 160 , 162 , 163 , 165 , 167 , 168 -169, 171 , 175 , 177 , 180 -181, 185 , 187 , 189 , 190 , 192 -193, 240
R
Ranunculus , 185 , 240
Rhamnus , 184 , 190 , 193 , 244
Rhizophora , 12 , 13 , 14 -15, 16 , 176 , 210 , 243
Rhododendron , 85 -86, 181 , 190 , 242
Rhus , 27 , 69 , 117 , 167 , 184 , 190 , 192 -193, 244
Rhynchosia , 28 , 243
Ricinus , 21 , 52 , 244
Robinia , 189 , 243
Rosmarinus , 82 , 245
Rubus , 68 , 108 , 243
Rumex , 36 , 53 , 55 , 60 , 65 , 68 , 87 , 148 , 155 , 183 , 241
Ruppia , 37 , 38 , 118 , 175 , 246
S
Sabal , 189 , 190 , 193 , 247
Saccharum , 70 -71, 72 , 109 , 247
Sagina , 60 , 241
Sagittaria , 161 , 188 , 246
Salicornia , 36 , 37 , 38 , 40 , 41 , 137 , 241
Salix , 48 , 49 , 50 , 52 , 53 , 56 -57, 58 , 60 , 68 , 134 -135, 136 , 148 , 157 , 190 -192, 242
Salsola , 38 , 85 , 96 , 112 , 241
Salvia , 117 , 166 , 245
Sapindus , 189 , 190 , 193 , 244
Sarcobatus , 85 , 110 , 241
Sassafras , 189 , 190 -191, 240
Saxifraga , 58 , 243
Scaevola , 3 , 20 -21, 24 , 25 , 118 , 246
Schismus , 83 -84, 85 , 247
Sciadopitys , 189 , 203 , 239
Scirpus , 5 , 40 , 46 -47, 247
Secale , 220 , 247
Sedum , 60 , 243
Senecio , 5 , 39 , 47 , 52 , 54 , 65 , 72 , 98 -99, 116 , 228 , 246
Sequoia , 181 , 189 , 190 -191, 203 , 229 , 239
Sequoiadendron , 74 , 129 -131, 196 , 203 , 210 , 229 , 239
Serruria , 132 , 244
Susuvium , 18 -19, 28 , 118 , 241
Setaria , 100 , 104 , 247
Silybum , 115 , 246
Sisymbrium , 112 , 242
Sitanion , 54 , 110 , 112 , 247
Solanum , 100 , 245
Solidago , 27 , 128 , 246
Sonchus , 39 , 52 , 65 , 87 , 100 , 246
Sonneratia , 210 , 243
Sophora , 18 -19, 243
Sorbus , 60 , 68 , 243
Sorghum , 104 -105, 221 , 247
Spartina , 12 , 16 , 27 , 28 , 36 , 37 , 39 , 137 , 227 -228, 247
Spartium , 82 , 243
Spergularia , 97 , 98 , 241
Spinifex , 29 , 247
Spiranthes , 5 , 7 , 140 , 247
Spondias , 175 , 244
Sporobolus , 18 -19, 21 , 28 , 247
Stellaria , 39 , 183 , 241
Stipa , 84 , 85 , 110 , 247
Styrax , 189 , 190 , 242
Suaeda , 38 , 98 , 148 , 183 , 241
Suriana , 18 -19, 21 , 244
Symphoricarpos , 116 , 246
T
Tabebuia , 24 , 246
Tamarix , 50 -51, 136 , 242
Taraxacum , 54 , 72 , 84 , 128 , 246
Taxodium , 137 -138, 189 , 190 , 239
Taxus , 189 , 202 , 234 , 240
Tecoma , 24 , 246
Terminalia , 18 -19, 21 , 22 , 24 , 210 , 243
Tetraclinis , 189 , 240
Themeda , 121 , 247
Thrinax , 18 -19, 247
Thuja , 129 , 189 , 190 , 240
Tilia , 63 , 79 , 86 , 152 , 154 , 181 , 189 , 190 , 241
Torreya , 189 , 190 , 240
Tournefortia , 3 , 18 -19, 20 , 21 , 25 , 245
Tragopogon , 228 , 246
Trapa , 156 , 188 , 243
Trema , 78 -79, 108 , 175 , 179 , 242
Trifolium , 221 , 243
Triglochin , 39 , 60 , 148 , 246
Tsuga , 57 , 69 , 79 -81, 126 -127, 129 , 160 , 161 -162, 164 , 181 , 187 , 189 , 190 , 239
Typha , 12 , 46 , 47 , 60 , 136 , 161 , 247
U
Ulex , 53 , 243
Ulmus , 49 , 51 , 79 , 89 , 91 -92, 134 -135, 152 , 154 -156, 160 , 162 , 180 -181, 189 , 190 -191, 194 , 242
Umbellularia , 52 , 190 , 240
Uniola , 26 , 28 , 247
V
Vaccinium , 60 -61, 67 -68, 157 , 242
Verbascum , 85 , 245
Vitis , 181 , 244
W
Weinmannia , 58 -59, 185 , 243
Wigandia , 65 , 245
X
Xanthium , 52 , 246
Y
Yucca , 166 -167, 168 , 247
Z
Zea , 221 , 247
Zelkova , 189 , 190 , 242
Zizyphus , 120 , 244
Zostera , 36 , 37 , 38 , 246
Preferred Citation: Sauer, Jonathan D. Plant Migration: The Dynamics of Geographic Patterning in Seed Plant Species. Berkeley: University of California Press, c1988 1988. http://ark.cdlib.org/ark:/13030/ft196n99v8/