7.3.4—
Cell Division
At least two types of microtubule can be distinguished in most spindles, those that traverse the spindle from pole to pole (continuous fibres), and those that connect the kinetochores of the chromosomes to the poles (chromosomal or kinetochore fibres). During separation of chromatids in anaphase, the continuous fibres lengthen, increasing the distance between the poles, while the chromosomal fibres usually—but not always—shorten, further separating the chromatids. After telophase, the continuous spindle may persist and be added to in the interzonal region by numerous additional microtubules, leading to formation of the phragmoplast and eventually, the cell plate.
Considerable changes in microtubule organization (Fig. 7.5, A–F) take
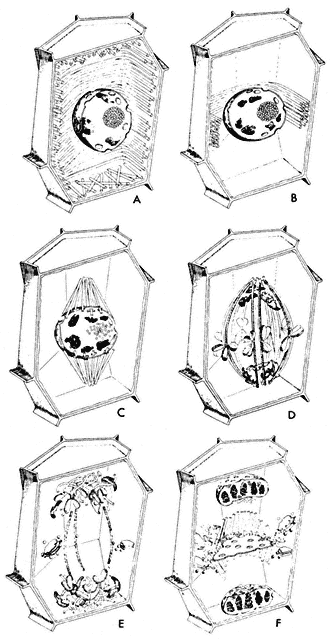
Figure 7.5
Diagram of changes in microtubule orientation and distribution during the phases
of mitosis in a higher plant cell. A—interphase; B—preprophase; C—prophase;
D—metaphase; E—anaphase; F—telophase. From Ledbetter & Porter (1970)
Introduction to the Fine Structure of Plant Cells, p. 44, Springer-Verlag, Berlin,
reproduced by permission of the authors and Springer-Verlag.
place during the events of a typical mitotic cycle (Fuge, 1974). With the onset of division, the peripheral cytoplasmic microtubules of the interphase cell disappear. Concomitantly, a band of microtubules is formed, several layers deep, lying close to the cell wall and girdling the nucleus perpendicularly to the prospective spindle axis. This preprophase band of microtubules appears to predict the plane of the future cell plate (Pickett-Heaps & Northcote, 1966). This has been demonstrated in divisions destined to give daughter cells of different size, for example in the asymmetric divisions of guard mother cells of wheat.
At the beginning of prophase, the number of microtubules in the preprophase band decreases, and new microtubules appear close to the nuclear membrane, this time running parallel to the prospective spindle axis. The microtubules in this so-called 'clear zone' in turn appear to furnish protein material for the spindle. It is possible that a total transformation of these extranuclear microtubules into spindle microtubules takes place.
Prior to spindle formation, the nuclear envelope either disintegrates or becomes perforated at the prospective poles, and cytoplasmic (clear zone) microtubules enter the karyoplasm at these sites. Some of these microtubules appear to establish connections with the chromosomal kinetochores. Since the number of kinetochore microtubules (kMts) increases as prometaphase proceeds, and since clear zone microtubules can be utilized only immediately after breakdown of the nuclear envelope, it is likely that de novo microtubule assembly also occurs at the kinetochores. Thus, it appears that the kinetochore, which varies in appearance in different organisms from amorphous to highly structured, acts as an initiating site or microtubule morganizing centre (MTOC).
In prometaphase, continuous fibres also begin to form and to increase in number. There is some controversy as to whether in higher organisms the continuous microtubules pass from pole to pole. A large proportion appear to overlap in the interzone between the half-spindles (Fig. 7.6), and have been termed non-kinetochore microtubules (nkMts). In lower plants, and all animal cells, with centrioles, the continuous or nkMts originate near the centrioles. These loci are represented by amorphous, electron-dense aggregates of material, and behave as another set of MTOCs. The essential feature of the centriole in mitosis seems to be in determining the spindle axis. In higher plants that lack centrioles, neither the location nor form of polar MTOCs is clear.
During metaphase, engagement of sister chromatids to opposite poles takes place via microtubules, followed by movement of the chromatids to the equatorial plate. At this stage, the maximum number of spindle microtubules is attained, the numbers often trebling between prometaphase and metaphase. Estimates from microtubule counts in serial sections of the metaphase spindle of the sea urchin, Arbacia, indicate a total microtubule length of 50,000 µm. The very large
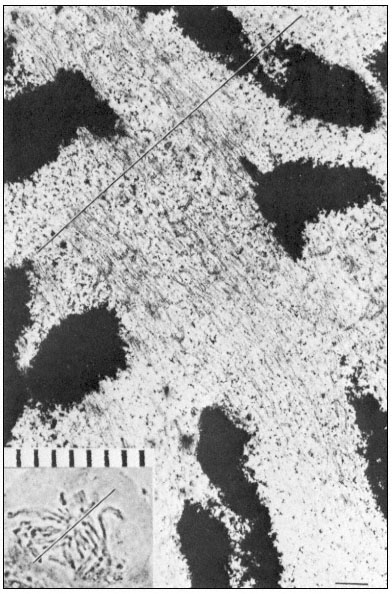
Figure 7.6
Electron and light micrographs of a dividing cell in prometaphase showing
kinetochore and nonkinetochore microtubules. The line indicates the equatorial
plate. Scale marker 1 µm. From Bajer (1968) Symp. Soc. exp. Biol. 22, 285–310,
reproduced by permission of the author and University Press, Cambridge.
spindles of Haemanthus endosperm cells contain considerably more microtubules than those of Arbacia.
Anaphase separation of the chromatids involves direct translation of the kinetochores at almost uniform velocity (0.2–4 µm min–1 ) towards their respective poles. Two experimentally separable processes are involved: first, a movement of the chromatids towards their poles, during which the chromosomal fibres shorten to as little as 20% of their metaphase length; secondly, an elongation of the spindle itself, during which the distance between the poles increases, thereby further separating the two groups of chromatids.
Time-lapse films of dividing Haemanthus endosperm cells show that many other objects, such as vesicles and granules, are commonly transported polewards during prometaphase-anaphase. Conversely, in onion root tip cells, the poleward movement of the chromosomes is accompanied by a reciprocal movement of other materials from the poles towards the mid-plate. Elements of the endoplasmic reticulum accumulate at the poles during metaphase, enter the spindle at anaphase, pass between the chromosomes moving in the opposite direction, and eventually aggregate and coalesce at the mid-plate to give rise to the transverse cell wall at telophase.
Dissolution of kMts and nkMts during telophase is accompanied by the formation of new microtubules in the interzone, leading to the formation of the phragmoplast in higher plant cells (Fig. 7.7). Phragmoplast microtubules appear to be involved in the movement, alignment and fusion of ER- and
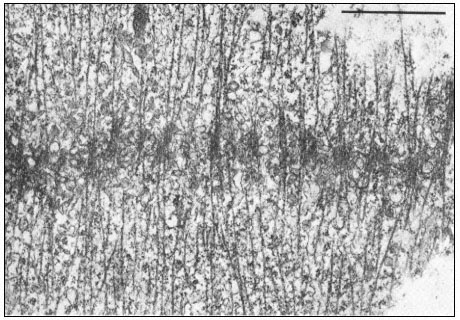
Figure 7.7
Phragmoplast microtubules at the mid-plate during telophase.
Vesicles contributing to the forming cell plate can be seen aligned among the
microtubules. Scale marker 1 µm. From Hepler & Jackson (1974) J. Cell. Biol. 38, 437–46,
reproduced by permission of the authors and The Rockefeller University Press.
dictyosome-derived vesicles to form the cell plate—yet another example of microtubule-associated intracellular transport.
Thus, distinct changes in the polarity and distribution of spindle microtubules take place during the various phases of the mitotic cycle. It should be borne strongly in mind that such changes occur under conditions of very low protein synthesis. Consequently, both assembly and functioning of the mitotic apparatus must involve regulation of microtubule polymerization at a physico-chemical level. Interaction of microtubules with other components of the mitotic apparatus must also be regulated at this level. Several hypotheses have been proposed to explain the mechanism of mitotic movements, although little direct experimental evidence to support the models has yet been obtained.
One prevailing hypothesis to account for spindle elongation (Brinkley & Cartwright, 1971) is that the poles are pushed apart by tip growth of continuous microtubules in the course of which subunits from disassembled kMTs may be utilized. Shortening of the chromosomal fibres is thought to occur by depolymerization at the poles.
Another hypothesis based on the sliding of adjacent spindle microtubules was proposed by Mcintosh et al., (1969). Earlier, several workers had reported the existence of arms or cross-bridges between spindle microtubules. The basis of the sliding tubule hypothesis is that the intertubular bridges in the spindle serve as mechano-chemical elements. Sliding of two microtubules against each other is thought to be generated by the successive breaking and reforming of cross-bridge linkages, a process that is energy-consuming and mediated via ATPase activity of the bridges. It has been estimated that the energy released through the hydrolysis of approximately 20 ATP molecules would suffice to move an average chromosome from the metaphase plate to the pole.
A prerequisite for the McIntosh model is an ordered system of long microtubules, emanating from the poles and reaching far into the opposite half-spindles in metaphase. Microtubules from opposite poles are suggested to have antiparallel polarity with regard to the direction in which their lateral arms could produce force. The kMts in each half-spindle would also show polarity; their lateral arms would only produce force directed towards the spindle equator. If one of the long interpolar microtubules and a kMt of antiparallel polarity came into contact at anaphase, the two tubules, due to the directed activity of their arms, would push themselves in opposite directions by sliding. The model is analogous to the generally accepted model for the interaction of actin and myosin in skeletal muscle. The mechanism could cause chromosome separation, as well as spindle elongation when anti-parallel nkMts interact where they overlap.
A third hypothesis involving actin-microtubule interactions (Forer & Behnke, 1972) has derived from observations of actin-like thin filaments in the mitotic or meiotic spindles of both animal and plant cells. Fluorescein-labelled heavy meromyosin binds to isolated sea urchin spindles. At the ultrastructural level, microfilaments within the spindle have been decorated with heavy
meromyosin, a test for actin-like proteins (Gawadi, 1971; Forer & Behnke, 1972). Several other recent publications have confirmed the presence of actinlike filaments in dividing cells. In kangaroo rat cells, actin was only demonstrable in association with chromosomal fibres (Sanger, 1975), suggesting an actinmyosin type interaction as the force-producing mechanism for chromosome movements, that is, for the autonomous movements of chromosomes in anaphase, as opposed to spindle elongation which may be controlled solely by microtubule assembly-disassembly.
Myosin-like proteins have not yet been located in spindles. Several workers have suggested that microtubules might serve as rigid structures against which the actin filaments could exert a contractile force by the formation and breakage of cross-links. A Ca2+ -dependent ATPase (similar in this respect to both myosin and dynein) is present in the isolated spindle in a concentration three times that in the cytoplasm (Mazia et al., 1972).
The theories on the mechanisms involved in chromosome separation have arisen from observations of living or fixed, intact cells. Such studies are hampered by the inability to experiment directly with the mitotic apparatus, except as it exists in the complexity of its cellular environment. Exciting possibilities have been raised by recent successful attempts to isolate and experiment with the mitotic apparatus in vitro. Intact spindles from the eggs of the surf clam show normal sensitivity to temperature and colchicine. These isolated spindles can incorporate brain tubulin during their reassembly and such hybrid spindles are also cold labile, Ca2+ -sensitive and capable of considerable increase in overall length (Rebhun et al., 1974). Moreover, early anaphase spindles isolated from kangaroo rat cells will continue chromosome motion, in the absence of exogenous spindle subunits, when ATP is added (Cande et al., 1974). These results already suggest that while spindle growth requires microtubule polymerization, anaphase movements do not.