3—
Geologic History of the White-Inyo Range
Clemens A. Nelson, Clarence A. Hall, Jr., and W. G. Ernst
Introduction and General History
The White-Inyo Range (Fig. 3.1), representing the westernmost range of the Basin and Range structural province, extends for 110 mi (175 km) from Montgomery Pass south-southeastward to Malpais Mesa opposite Owens Lake. Its maximum width, east of Bishop, is approximately 22 mi (35 km). The terminological separation of White from Inyo mountains is placed along the Westgard-Cedar Flat-Deep Springs Valley Road, a division that has no particular topographic or geologic significance. As is typical of the ranges of the province, it is bounded, generally on both sides, by normal faults of large-magnitude slip. The northern part of the range is mainly an easterly tilted block marked by an impressive escarpment from the Owens-Chalfant-Hamill valleys on the west, at 4,300 ft (1,310 m), to the crest of the range at White Mountain Peak, 14,246 ft (4,342 m). The southern, Inyo Mountains, part of the range has been tilted slightly to the west, with its maximum relief from Saline Valley at 1,100 ft (353 m) on the east to the range crest at Mt. Inyo, 11,107 ft (3,385 m).
Rocks in the White-Inyo Range span the time from the late Precambrian (700 Ma [Ma, millions of years ago]) to the Holocene, or Recent (i.e., last 10,000 years). Figure 3.2 is a simplified geologic timetable showing major time units and millions of years before the present (Ma) for the beginning of each, and the time spans of mountain building episodes (orogenies) referred to in the text. All periods of the Paleozoic (570–225 Ma), Mesozoic (225–65 Ma) and Cenozoic (65 Ma to the present) are represented, some incompletely. Rocks from the late Precambrian to the end of the Devonian Period (345 Ma) are entirely of sedimentary origin, having been deposited as sand, shale, dolomite, and limestone along the western edge of the North American continent by stream systems flowing westward into a marine basin called the Cordilleran geosyncline (Fig. 3.3). The total accumulation of uppermost Precambrian through Lower Jurassic surficial deposits in the geosyncline exceeded 4.5 mi (7 km) in thickness in the White-Inyo region. Beginning in Mississippian time, about 345 million years ago, the sedimentation pattern changed in response to elevated lands lying to the north and possibly west, resulting in the accumulation of coarse-grained sands and conglomerate, reflecting higher-energy stream systems. This pulse of uplift has been considered by some geologists as the result of the first of several collisions of the North American lithospheric plate and an ancient Pacific plate; the inferred plate collision is held responsible for the Antler orogeny, or time
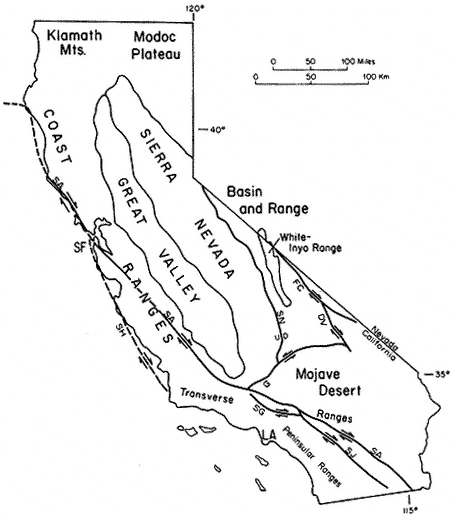
Figure 3.1
Relation of the White-Inyo Range to major physiographic and structural features of
California. Faults: SA — San Andreas, SN — Sierra Nevada, G — Garlock, SG — San
Gabriel, SJ — San Jacinto, FC — Furnace Creek, DV — Death Valley, SH — San
Gregorio-Hosgri; other abbreviations: SF — San Francisco, LA — Los Angeles. Arrows
show relative horizontal slip on faults. U, D indicate relative vertical slip on faults.
of mountain building. The Pennsylvanian and Permian (320–225 Ma) were times of renewed deposition of limestone, reflecting a return to conditions of quiet carbonate bank formation.
This lull was again succeeded by extensive tectonic activity, apparently resulting from a second plate collision, the Sonoma orogeny (230–220 Ma). This event, as in
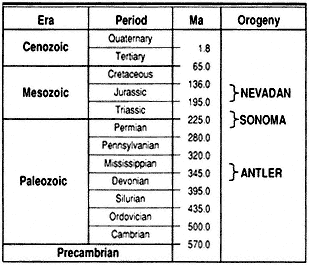
Figure 3.2
Geologic timetable showing millions of years before the
present (Ma) and the approximate time span of orogenies
mentioned in text.
the Sierra Nevada to the west, resulted in extensive volcanism in a marine environment, the products of which are exposed as interlayered lava flows, ash beds, and continental sedimentary rocks in the southern Inyo Mountains and in the northern White Mountains.
In both the White-Inyo Range and the Sierra Nevada, surficial volcanism and its deep-seated counterpart, igneous intrusion, continued intermittently until approximately 155 million years ago as a consequence of the most intense orogeny to affect the region, the Nevadan (see Fig. 3.2). This complex episode, the result of terrane
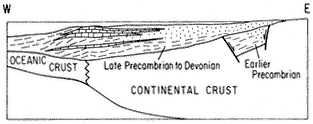
Figure 3.3
Schematic cross section of the western margin of North
America during late Precambrian (700 Ma) to Devonian
time (345 Ma) illustrating the mode of sediment
accumulation in the Cordilleran geosyncline. Sediment
transport direction was dominantly from east to west.
accretion (plate collision) and amalgamation of far-traveled subcontinental fragments outboard of the North American plate and large-scale subduction of an ancient Pacific plate beneath the North American plate, produced major compressional deformation, metamorphism, and the emplacement of numerous coarse-grained intrusive bodies, termed plutons. The latest Mesozoic (90–75 Ma) was a time of renewed plutonic activity, represented by cross-cutting granitic intrusive rocks that transect the earlier-formed deformational structures. The physical conditions inferred to have attended crystallization of the metamorphic minerals associated with the intrusive rocks document the presence of a volcanic-plutonic arc marking the western margin of the North American continent throughout Mesozoic time (Fig. 3.4).
The early and middle Cenozoic Era was a time of large-scale uplift and extensive erosion; no sedimentary record of this time has been left in the White-Inyo Range. A major erosion surface truncates all previously formed rocks, including the deep-seated granitic plutons, which had solidified at depths of perhaps 6 mi (10 km) or more. Beginning approximately 10 million years ago, the range experienced the outpourings of extensive fragmental volcanic ejecta (volcanic tuff) and basaltic lava flows. Remnants of this episode can be seen in the volcanic rocks that mantle the northeast corner of the White Mountains at Montgomery Pass and east, in the table-like flows extending from the Cottonwood Basin (SE of Mt. Barcroft) to the north end of Deep Springs Valley, in the large expanse of volcanic rocks covering the saddle area between Eureka and Saline valleys on the east flank of the Inyo Mountains, and in the Malpais Mesa at the south end of the Inyo Mountains.
The latest Cenozoic was a time of renewed uplift along Basin and Range normal faults flanking the range, and transcurrent motion along the Furnace Creek fault zone at the margin of the White Mountains and Fish Lake Valley. Both styles of faulting reflect a major episode of crustal extension that was initiated in the Basin and Range Province approximately 15 million years ago. That this style of deformation continues to the present in the White-Inyo region is attested to by such events as the Chalfant earthquakes of July 1986, which measured up to 5.5 on the Richter scale.
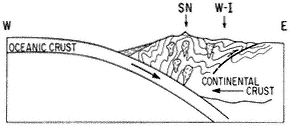
Figure 3.4
Schematic cross section of the western margin of North
America during the convergent episode of the Nevadan
orogeny, illustrating the development of compressional
fold and fault structures and the generation of granitic
bodies (SN — Sierra Nevada, W-I — White-Inyo Range).
An additional result of the uplift in latest Cenozoic time was the onset of Quaternary glaciation, which affected the area east and north of Mt. Barcroft and White Mountain Peak. This was followed by further uplift and the development of extensive aprons of alluvial deposits at the western and eastern margins of the range.
The Central White-Inyo Range
The area of principal interest for this guide extends from directly north of White Mountain Peak south to approximately 37°00¢ N. lat., a distance of 47 mi (75 km). All of this area has been mapped geologically at a scale of 1:62,500 or 1:24,000 (Fig. 3.5), and a more detailed discussion of its sedimentary, deformational, and igneous history is possible. This is also the area covered by the enclosed geologic map and by the principal and subsidiary road logs included at the end of this chapter.
The central White-Inyo Range exposes the best stratigraphic sections of the uppermost Precambrian to middle Paleozoic strata in the range. The basal part of the section (Fig. 3.6) contains the Precambrian-Cambrian transition. The Lower Cambrian portion of this section, the Waucoban Series, is regarded as the North American-type succession for rocks of this age. It contains the oldest trilobite faunas in the Americas, abundant archeocyathans (primitive reef-forming animals), numerous criss-crossing tracks and trails of primitive molluscs and arthropods, and molluscan body fossils (Wyattia and others), now regarded as marking the beginning of the Paleozoic Era. This section of strata and its fossils illustrate a remarkable explosion of life at the Precambrian-Cambrian boundary, in which "explosion" all invertebrate phyla are represented. These fossil representatives occur in the limited span extending from the upper Wyman Formation (animal tracks and trails), through the Reed and Deep Spring formations (primitive molluscs and fossil animal trails), to the Campito and Poleta formations (trilobites). This succession represents a group of rocks deposited in largely tidal and subtidal environments, as well as reefal and off-reef carbonate bank and shoal environments. The terrigenous strata are largely shale-siltstone and quartzite deposited on the shallow continental shelf of the time. It has been speculated that one could have walked across the early Paleozoic sea in the White-Inyo region in water only chest-deep. The strata contain abundant shallow water indicators, such as sedimentary rocks with cross-bedding, current and wave ripple marks, mud cracks, and very highly bioturbated beds.
The geologic structure of the area from 37°00¢ N. lat. north to just south of Mt. Barcroft is relatively simple: the range is largely anticlinal. North of Westgard Pass, the White Mountains are principally a gently south-plunging, asymmetrical anticlinorium (east flank nearly vertical) exposing the oldest stratigraphic unit, the Wyman Formation, in the core. The central, relatively simple structure (Fig. 3.7), is modified on both the west and east sides by more complex, closely appressed sets of compressional structures. The Inyo Mountains are dominated by a more open and nearly horizontal anticline with a southeast trend, also exposing the Wyman Formation at its core and similarly modified on its flanks by folds as well as faults.
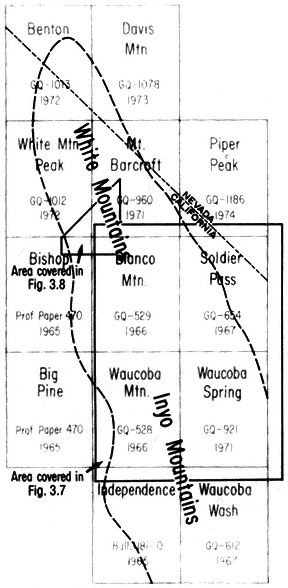
Figure 3.5
Index map of published geologic maps of the U.S. Geological Survey,
scale 1:62,500 (see references).
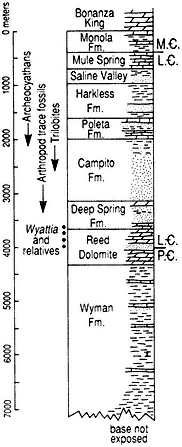
Figure 3.6
White-Inyo Range latest Precambrian
to Middle Cambrian stratigraphic
section, showing distribution of key
faunal elements of the
Precambrian-Cambrian transition.
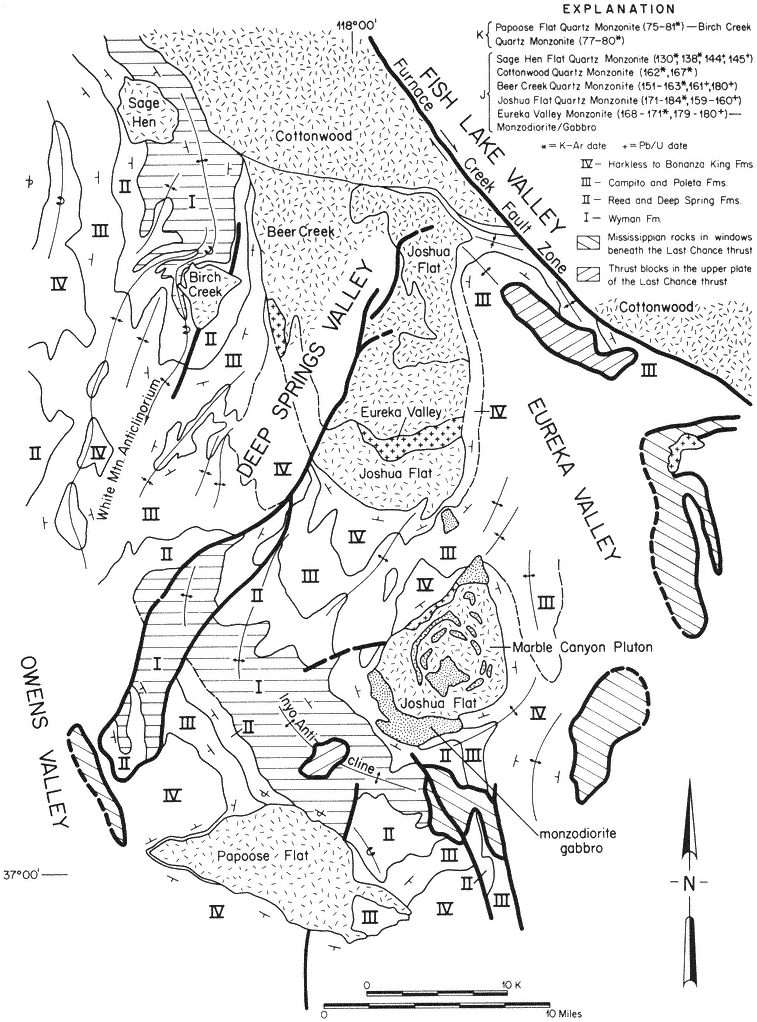
Figure 3.7
Generalized geologic map of the central portion of the White-Inyo Range showing
distribution, structural position, and radiometric dates of Jurassic (J) and Cretaceous
(K) plutons.
Lying between the Inyo anticline and the White Mountain anticlinorium is a structural downwarp containing many closely spaced anticlinal and synclinal folds and associated faults, along the western side of Deep Springs Valley. These are the famed Poleta folds, an area used for instructional purposes by many academic departments of geology.
The Inyo Mountains, and areas to the southeast, expose structures interpreted as the consequence of extreme compression: a system of low-angle reverse faults — extensive, gently inclined surfaces across which old rocks have been thrust from beneath, onto and over younger rocks. In the Inyo Mountains, this fault system has been termed the Last Chance thrust, along which Precambrian and lower Paleozoic rocks have been juxtaposed above rocks of middle and late Paleozoic age. In its northernmost exposures, the fault is inclined to the north, suggesting that the northern Inyo Range and a part of the White Mountains may lie entirely above the Last Chance thrust.
A roughly similar interpretation can be made for the area of the White Mountains north of Mt. Barcroft. Even though this part of the range has been invaded by many Mesozoic intrusive bodies (to be discussed later), the gross distribution of the pre-intrusive strata suggests that the lower to middle Mesozoic core of the range has been overthrust by Paleozoic and Precambrian strata, which appear to lie structurally above, along the northwest, north, and northeast flanks of the range.
Dating the many structural features of the White-Inyo Range is difficult because of the large temporal gap between the deformed and undeformed strata. Many of the structures are truncated by one or more of the Mesozoic intrusive bodies. Consequently, the major folding and thrust faulting can be regarded as pre-intrusive (pre-Nevadan), generally before 180 Ma, possibly consequences of the Antler (» 350–340 Ma) and/or the Sonoma (» 230–220 Ma) mountain building episodes (see Fig. 3.2).
The uppermost Precambrian to middle Mesozoic strata of the central White-Inyo Range are essentially "swimming" in a sea of middle to late Mesozoic intrusive rocks. The plutons have disrupted pre-intrusive structures, have metamorphosed the sedimentary strata to slate, schist, and quartzite, and in some cases have strongly deformed and stretched sedimentary layers. The plutons have been radiometrically dated and range in age from 180 to 75 Ma.
The plutons of the range exhibit a variety of emplacement mechanisms. This is well illustrated by the several bodies in the southern White Mountains and the northern Inyo Mountains (see Fig. 3.7). The oldest, the Marble Canyon composite pluton and the Eureka Valley and Joshua Flat plutons, are Jurassic in age. They have invaded the axial portion of major synclinal (downwarp) and basinal structures to the east and northeast of the White Mountain and Inyo anticlines, respectively. They are in part discordant to the original sedimentary layering but have principally shouldered aside their host rocks and locally thinned them to approximately 40% of the initial thicknesses.
The slightly younger Jurassic Beer Creek and Cottonwood plutons occupy the same synclinal downwarp but are more broadly discordant, as they cut across the eastern limb of the White Mountain anticlinorium. The youngest Jurassic pluton, the Sage
Hen Flat (145 Ma), is unique among the White-Inyo intrusive rocks in that it has not significantly deformed its host rocks. It has passively invaded the west flank of the White Mountain anticlinorium, apparently by a process of magmatic stoping: blocks of the overlying host rocks were fractured and, because of their greater density, sank into the magma chamber, allowing the molten granitic column to rise buoyantly.
Cretaceous intrusive activity in this area is represented by the Birch Creek and Papoose Flat plutons, each of which attained its present structural position by a process of forcible injection. Both appear to have initially invaded their host rocks along pre-intrusive faults (see Fig. 3.7). The Birch Creek Pluton occupies the east flank of the central portion of the White Mountain anticlinorium. During emplacement, it deflected to the northwest and overturned the central anticlinal structure and the strata of the east flank. The Papoose Flat Pluton was emplaced within the southwest limb of the Inyo anticline, drastically disrupting the southeast trend of the structure, producing a pronounced westward bulge and overturning the strata along its northeast border. Accompanying the bulging, the sedimentary succession at the contact with the pluton was in places stretched and thinned to less than 10% of initial thicknesses, an extreme example of forcible emplacement and accompanying attenuation of wall rocks.
North of the area of Fig. 3.7, Jurassic plutonism is represented by the Barcroft Pluton (see enclosed geologic map). The pluton transects the White Mountain anti-clinorium, diverting the western limb of the structure from its regional northward trend to the northeast, parallel to the southeast border of the intrusive (Fig. 3.8). Alternatively, this change in trend could be related to reverse movement along the Marmot thrust, a south-dipping fault within the Precambrian-Cambrian rocks directly south of the Barcroft Pluton. It has also been suggested that the pluton was emplaced along a steeply inclined structural discontinuity along which the Paleozoic rocks of the southern block had been uplifted or overthrust into contact with the Mesozoic rocks of the northern block.
The White Mountain anticlinorium with its N-S trend is thought by some to have developed during the Sonoma orogeny of latest Paleozoic to early Mesozoic age. The Cottonwood Pluton (Fig. 3.8) intruded preexisting folds in the Precambrian and Cambrian sequence and is apparently responsible for the overturning of the east limb of the anticlinorium.
The dominant minor fold axes in the Wyman and Deep Spring formations are approximately N-S and are nearly horizontal. Exposure of the Wyman and Deep Spring formations in the northeast part of Fig. 3.8 and south of the Marmot thrust are more highly deformed than in the southern part of the area depicted in Fig. 3.8. The dominant trends of minor fold axes in this more deformed area are N30°E. These folds are interpreted to be a result of deformation associated with the Marmot thrust and postdate the folds with N-S trends. An alternative interpretation is that the more highly deformed Wyman and Deep Spring formations in this area are related to emplacement of the Barcroft Pluton, and that the Marmot thrust follows the preexisting structural grain or was reactivitated during intrusion of the granodiorite pluton.
Folds with NE-SW or E-W trends in the Poleta Formation (see Fig. 3.8) north of the Marmot thrust are associated with the emplacement of the Barcroft Pluton. Metamorphic minerals (tremolite, scapolite, and diopside) in the Wyman Formation of this area and south of the surface trace of the Marmot thrust could reflect either the close proximity of the Barcroft Pluton at depth or, less likely, metamorphism that occurred during movement along the Marmot thrust. Thinning of the Reed Dolomite and the Deep Spring Formation in the northeast part of the area and above the Wyman-cored anticline or dome, as depicted in Fig. 3.8, suggests either: regional upwarping during deposition of the Reed and Deep Spring formations; thinning related to deformation associated with the White Mountain anticlinorium, along whose trend the dome in the Wyman Formation lies; or attenuation owing to the proximity of the Barcroft and Cottonwood plutons in a manner similar to that proposed for the Papoose Flat Pluton. Hence, folding or upwarping within the study area could have first occurred during the Cambrian or late Precambrian, and again during the Antler and/or Sonoma orogenies, or perhaps partly during the Nevadan tectonic events.
Plutons of Cretaceous age (90–75 Ma) in the region to the east and northeast of Mt. Barcroft and White Mountain Peak are represented by the Indian Garden Creek and McAfee Creek granitic bodies, which occupy large areas on the east slope of the White Mountains (see enclosed geologic map). The Indian Garden Creek Pluton is entirely within and discordant to the Cottonwood Pluton. The McAfee Creek Pluton intrudes Precambrian strata, the Cottonwood Pluton, and the Barcroft Pluton.
Recrystallization (metamorphism) of the Precambrian, Paleozoic, and Mesozoic strata of the White-Inyo Range took place repeatedly in response to the periods of heating and deformation just described. The early metamorphic mineral assemblages were overprinted and were partly to completely replaced by other assemblages produced by the younger thermal events. The rocks are typified by a regional development of greenschist facies minerals; common phases in recrystallized, stratified rocks include quartz, albite, microcline, white mica, chlorite, biotite, epidote, and magnetite. Thermal annealing reflects more intense baking occasioned by the emplacement of plutons of various ages; many, but not all, have produced distinct metamorphic aureoles or halos in the surrounding strata, indicated by the localized formation of new, higher-temperature minerals such as garnet, cordierite, tremolite, tourmaline, fluorite, scapolite, andalusite, sillimanite, and calcic plagioclase.
In the central White-Inyo Range, the record of the early to middle Cenozoic (65-15 Ma) is missing. During this time, broad regional uplift resulted in the unroofing of the range, exposing the deep-seated granitic bodies. Erosional products of the uplift and resulting denudation were probably shed chiefly westward, across what is now the Sierra Nevada (then low-lying), to the Cenozoic seaway in the region of the present San Joaquin Valley. With the development of crustal extension beginning about 15 million years ago, erosional debris was transported to adjacent down-dropped basins such as the Owens, Fish Lake, Eureka, and Saline valleys.
The character of the erosional surface produced by this long period of denudation is well illustrated in the area of Cottonwood Basin, southeast of Mt. Barcroft. From there to east of the north end of Deep Springs Valley, Miocene volcanic tuff and
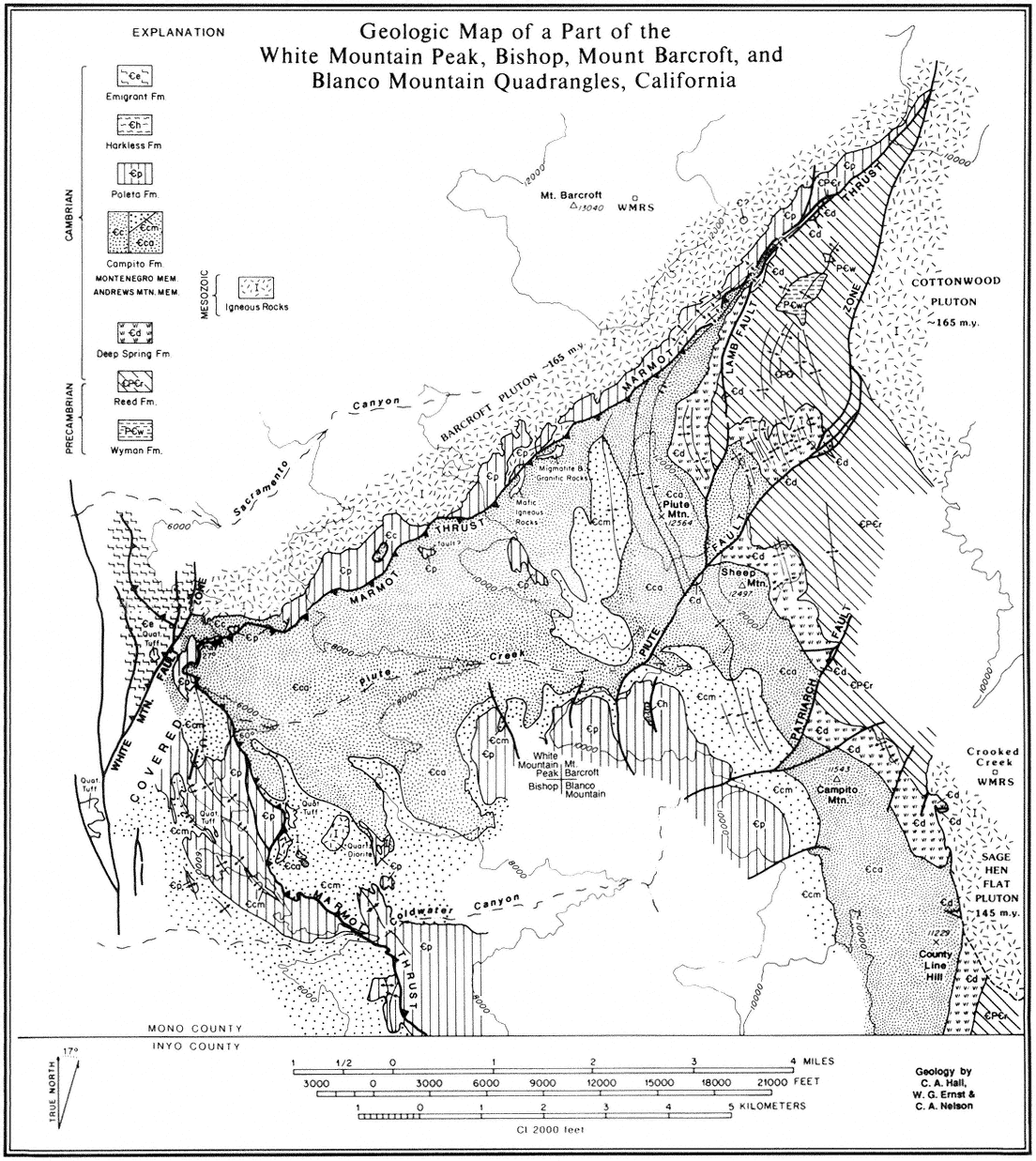
Figure 3.8
Geologic map of the southern part of the Barcroft Pluton and associated structures.
overlying basaltic flows (10 Ma) lie upon a generally smooth surface planed across the Jurassic plutonic rocks of the range. This erosional surface and its basaltic cover was uplifted and tilted, mainly to the east, as shown by its elevation of 11,800 ft (3,595 m), 2.5 mi east of Mt. Barcroft on the northwest, to 5,500 ft (1,675 m) in Deep Springs Valley, from where it has been uplifted along a marginal fault to an elevation of 7,700 ft (2,350 m) east of the valley at Piper Mountain.
The development of Deep Springs Valley is typical of the late Cenozoic history of the region. The valley lies wholly within the range and trends northeastward across the regional structural grain. It is marked by a major fault system along its eastern margin, as illustrated by the steep escarpment, with a relief of 2,400 ft (730 m), east of the valley, and numerous small fault scarps, which attest to the recency of uplift. Geophysical data suggest that structural relief between the granitic rocks on the east side of the valley to the granite surface below the valley fill is as much as 5,000 ft (1,520 m) and that the alluvial fill beneath Deep Springs Lake on the east side of the valley is approximately 2,600 ft (790 m) thick.
An additional aspect of the crustal extension that the region has experienced is illustrated by the fault system that marks the margin between the northeast flank of the White Mountains and Fish Lake Valley. This is the Furnace Creek fault, which extends from Death Valley to its termination at the northwest end of Fish Lake Valley. It is a major transcurrent fault, along which the White Mountain block has been moved, principally horizontally, northwestward perhaps as much as 13 mi (20 km) relative to rocks on the northeast side of the fault.
As discussed previously, uplift during the late Cenozoic resulted in elevations sufficiently high to support small valley glaciers during Quaternary time. Small cirque basins and glacial moraines occupy the upper reaches of Leidy, Perry Aiken, McAfee, and Cottonwood creeks on the east slope of the White Mountains.
A major, relatively recent geological event, the extrusion of the Bishop Tuff from the Long Valley caldera east of Mammoth Lakes, had a marginal effect on the White Mountains. The event, which took place 700,000 years ago, was a huge volcanic explosion that resulted in the outpouring of pumice and ash amounting to more than 144 cu mi (600 cu km) and the collapse of the base of the caldera to below sea level. The volcanic tuff resulting from this eruption mantles or underlies much of northern Owens Valley and most of Chalfant and Hamill valleys, and it occurs interbedded with alluvial materials flanking the west slope of the White Mountains and in several canyons, notably Black Canyon, southeast of Bishop.
That uplift and associated volcanism has continued to the present is suggested by the presence of the very recent scarps along the range margins, especially marked along the east side of Deep Springs Valley (Road Log C), by a series of young volcanic cinder cones on the west side of the Inyo Mountains southeast of Big Pine, and by the historic earthquakes of the general eastern Sierra Nevada-Owens Valley region. This region has had a long and diverse geologic history, and geologic activity continues to the present day.