Three Geothermal Systems in Iceland: Krafla, Surtsey, and Heimaey Volcanoes
Iceland is located over the Mid-Atlantic Ridge, which is an active spreading center. Regions of active extension and volcanism in Iceland are called the neovolcanic zones and cross the island generally from south-west to northeast. Most volcanic activity occurs along the eastern and western volcanic zones, where plate motion currently averages 1.6 cm/year in either direction and lavas and pyroclastic deposits are erupted at a rate of 0.04 km3 /yr. The largest single historic eruption of basaltic lavas (12 km3 ) occurred in 1783 from the 25-km-long Laki fissure (Sigvaldason, 1974). The active state of this island and the extent of its geothermal resources are reflected in the thermal gradients, which vary from ~160°C/km (heat flow = 300 mW/m2 ) in the fissure swarms to 40°C/km (heat flow = 80 mW/m2 ) in the oldest rocks along the island margins (Pálmason, 1973). Fissure swarms in the neovolcanic zones range from 5 to 10 km wide and are 30 to 100 km long. Each zone consists of nested grabens, where near-vertical normal faults are exposed at the surface. Figure 6.14 illustrates the active faulting, volcanism, and geothermal systems that occur along these fissure swarms.
The economy of Iceland is closely linked to geothermal energy. Half of the population lives and works in buildings heated by geothermal waters. Greenhouses, an important component of the island's agricultural effort, are also heated by hot springs and water from geothermal wells. Many hot water wells have been drilled for direct-use applications, especially around the capitol city of Reykjavik. These waters are pumped from reservoirs located in Tertiary-age interbedded flood basalts and hyaloclastite deposits at depths of 1 to 2 km and temperatures of 86 to 128°C. Hot water is conducted horizontally along the basal contacts
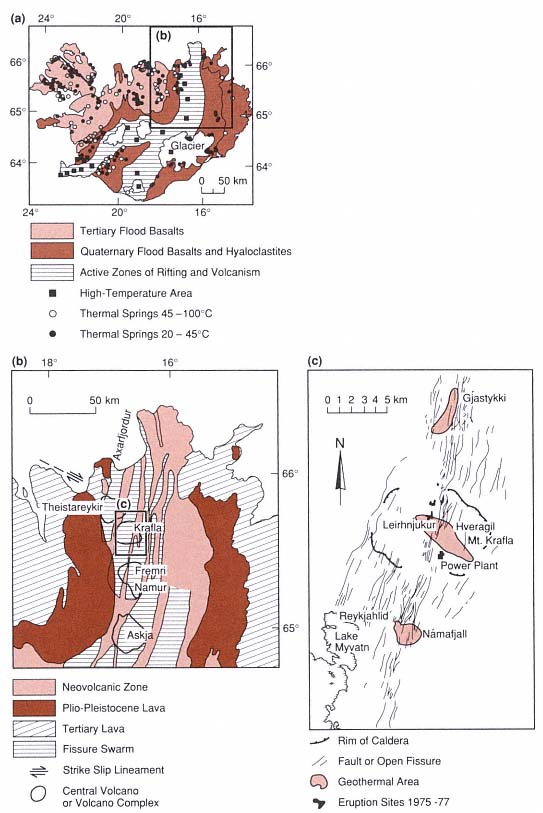
Fig. 6.14
(a) Simplified geologic map of Iceland shows the distribution of
geothermal manifestations and their relation to active volcanic zones.
(Adapted from Fridleifsson, 1979.)
(b) Fissure swarms and central volcanoes of the Northeast volcanic zone of Iceland
(Adapted from Stefánsson, 1981.)
(c) Structural map of Krafla caldera and its active fissure swarms
(Adapted from Stefánsson, 1981.)
of lava flows and vertically along dikes and faults; Bodvarsson (1961) presents hydrologic and chemical evidence that these waters can flow laterally for as far as 50 km.
High-temperature geothermal systems are associated with the young volcanic fields along the active tectonic rifts. Most have temperatures of 200 to 300°C at depths of 1 to 2 km. Bodvarsson (1976) estimated that nearly 400 km2 of Iceland, along the neovolcanic zones, is underlain by high-temperature geothermal systems. The Krafla field, which is representative of these hydrothermal systems, has been developed in and around Krafla caldera in northeastern Iceland, as described in the next section.
Migration of Magma and Dike Formation
Information on the movement, shape, and size of magma bodies below fissure swarms is based on both geophysical measurements during recent eruptions and structural/volcanologic studies of historic eruptions. Magma overpressures result in the beginnings of extension, which in turn lead to magma rise and eruption. Sigvaldason (1987) inferred that during the 1975–1981 Krafla eruptions, magma moved through "holding chambers" at depths of 30, 25, 8, and 4 km. After the eruptions, refilling of these intermediate chambers took ~3 weeks. From the high-level chambers—at depths of 3 to 7 km—repeated lateral magma injections into fissure swarms north and south of the Krafla central volcano initiated a rifting event. [This rifting episode was activated by the subsequent release of tensional stress that accumulated over the plate boundary during the previous 250 years (Tryggvason, 1984)]. The fissure swarm was extended by an 80- to 90-km-long section during this period; the average widening for a fissure during the accumulated 20 discrete events was 5 to 6 m (Tryggvason, 1984). Each extensional event was accompanied by subsidence near the center of the Krafla caldera, which demonstrates the link between the fissure swarms and the high-level chamber below the central volcano.
Tryggvason (1984) determined that the accumulated area of fissure widening during the 1975–1981 Krafla event was ~377,000 m2 . Based on observed ground deformation, he suggested that most of the magma was injected into vertical fissures rather than into sills. Using gravity and elevation measurements, he determined that the volume of magma leaving the reservoir was ~1.75 times that of caldera subsidence. The dike volume (V) is equated with that of magma leaving the reservoir, minus the volume of material erupted. The estimated dike height (h) = ~1.75 V/A, where A = area of horizontal extension by the dike. For the best recorded events at Krafla, calculated dike heights are 2.4 to 2.8 km. The total volume of magma that flowed out of the reservoir into fissure swarms during this episode at Krafla is estimated at 1.08 km3 , of which 1.03 km3 remains in the dikes to become a renewed heat source for the associated geothermal fields. The measured volume of lavas erupted is 0.2 km3 , which is four times greater than the volume predicted. Tryggvason concludes that perhaps the volume estimates of magma leaving the caldera are too low.
Gudmundsson (1986) used his work on the Reykjanes Peninsula of Iceland to develop a method for estimating the volume of magma reservoirs below fissure swarms, as is depicted in Fig. 6.15; his method requires the measurements and assumptions listed here.
· The maximum length for a magma reservoir is taken to be equal to the length of vents in the fissure swarm.
· The width of the reservoir is estimated to be 1.72 times the width of the vents in the fissure swarm; this ratio is based on observations of older dike swarms that are exposed in outcrop.
· A reservoir is taken as an ellipsoid, the volume of which is calculated as V = 4/3 p ah bh ch and the area of which is calculated as A = p ah bh ,
where ah , bh , and ch = the half-width, half-length, and half-thickness, respectively, of the ellipsoid. The half-thickness of the reservoir is calculated by ch = 0.75 V/A, where V = total volume of the magma (both erupted and in dikes).
· The average volume of individual fissure lava flows on the peninsula is 0.11 km3 . Walker (1959) estimated the average volume of a corresponding feeder dike by using the average length of the volcanic fissures (2.2 km), a crustal thickness of 8 km, and an average dike width of 4 m. The estimated volume of a feeder dike here is 0.07 km3 and an average value for ch is 1.5 km (2ch = 3 km). It is likely that only the uppermost 3 km of the reservoir participates in an eruption.
The volume of feeder dikes (~0.07 km3 ) is small, but as a result of intrusions and eruptions every 10 years, the active fissure swarms contain excellent heat sources. Bodvarsson (1976) calculated that in Iceland, heat reaches the surface by conduction (~50%), as erupted magma (~30%), and as thermal waters (~20%).
South of Krafla caldera is Askja-Öskuvatn caldera; Sigurdsson and Sparks' (1978) documentation of the 1874–1875 eruption provides another view of fissuring and dike injection along the fissure swarms. The Askja central volcano straddles a 75-km-long fissure swarm. Magnitude 6 or 7 earthquakes in 1872 marked a new phase of rifting, and by the fall of 1874 the fissure swarm was rifted along a 70-km segment. A graben 1 to 2 km wide was formed, bounded by normal faults with throws of 40 to 60 m south of the central volcano and 10 m north of it. In early January 1975, a major injection of magma into a high-level reservoir was followed by phreatomagmatic eruptions of rhyolitic ash. Caldera inflation was relieved by periodic injections of magma out into the fissure swarms. At the surface, the central fissure is flanked by en echelon spatter ramparts. The Sveinagja lava field covers 30 km2 ; it is
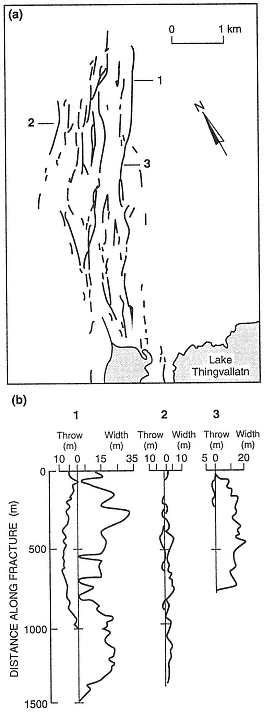
Fig. 6.15
(a) Fractures measured in the western part of the
Thingvellir swarm in Iceland. (b) The width and
throw of three fractures from the Thingvellir
swarm were measured along
the strike of the fissures.
(Adapted from Gudmundsson, 1987.)
located 40 to 70 km north of the Askja-Öskjuvatn caldera and consists of 0.3 km3 of mostly tholeiitic aa and pahoehoe lavas. The estimated volume of intruded magma was 1.5 km3 , which could be accounted for by a single 100-km-long, 5-km-deep, 3-m-wide dike. Fissure widths range from 2.5 to 4 m. In the northern part of the lava field, activity was centralized at an offset in the rift and formed a line of cinder cones.
Brown et al . (1987) used gravity surveys to document the presence of a 20-mGal, north-south-trending anomaly that may correspond with a dense dike swarm below the Öskuvatn-Askja caldera. They also noted that caldera fill is most likely thin and that the caldera's collapse was primarily related to eruptions out along the fissure swarms.
Hydrothermal Reservoirs
Hydrothermal reservoirs in Iceland are usually bounded by lava flow contacts or clay-rich hyaloclastite deposits. Water in aquifers can also pond when dikes act as barriers. By measuring the deuterium content of thermal waters, Arnoson (1976) has shown that the waters are of meteoric origin, although a few systems are charged with seawater. In most cases, water from the highlands percolates into bedrock and flows laterally for distances of as much as 150 km but more usually several tens of km. The water then rises to the surface along dikes or faults. Tables 6.1 and 6.2 summarize potential reservoir rocks and the flow rates through these rocks as sampled by drilling (Friedleiffson, 1975; Tómasson et al ., 1975).
Pillow lavas have a higher effective permeability than any other rock type encountered by drilling in Icelandic geothermal areas (Friedliefsson, 1978/79). Subglacial fissure eruptions produce elongate ridges (mobergs), as shown in Fig. 6.16, that are 1 to 5 km wide, tens of kilometers long, and a few hundred meters thick. The cores of these ridges consist of permeable pillow lavas, but the flanking hyaloclastite deposits can serve as aquitards. Subglacial eruptions are remarkable in that they are able to create both the reservoir and the caprock in one volcano.
Krafla volcano has three high-temperature geothermal fields, which are located
· within the 8- by 10-km Krafla caldera, where the thermal area is outlined by explosion craters, surface manifestations, and altered ground (Fig. 6.17). [Further exploration to define the reservoir included Schlumberger resistivity soundings and analyses of fumarolic gases. In this 35-km2 thermal area, the temperature in a 2-km-deep drillhole reached 345°C (Stefánsson, 1981)];
· 6.2 km south of the caldera along the fissure swarm at Námafjall, where the geothermal area covers 4 km2 ; and
· 5 km north of the caldera, also along the fissure swarm at Gjástykki; surface manifestations encompass an area 1 by 4.5 km parallel to the swarm.
|
|
Drilling within the low-resistivity zone revealed three main rock units: hyaloclastite deposits, lava flows, and dikes, as shown in Fig. 6.17. Below a depth of 800 m, lava flows are dominant, but from a depth of 400 m to the bottoms of the deepest wells, dikes are more common. The transition depth from zeolite to greenschist facies metamorphism is at ~800 m (Stefánsson, 1981).
In his model of the Krafla field, Stefánsson (1981) identified two hydrothermal zones. The shallowest, extending to 1100-m depth, is water-dominated and has a maximum temperature of 205°C; the basal contact of this zone coincides with the base of a sequence of lava flows. Within the deeper zone—from 1100 to 2200 m (total depth for the deepest well)—the reservoir contains a mixture of
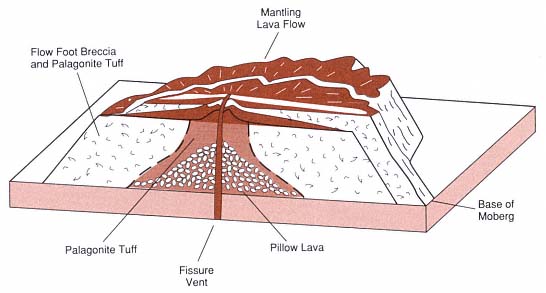
Fig. 6.16
Table mountain, or moberg, formed during eruption of basalt along a fissure under a glacier.
(Adapted from Jones, 1969.)
steam, water, and CO2 . The rocks, consisting of mostly lavas, a granophyre unit, and a dolerite sill, are intruded by multiple dikes. The two reservoirs are connected by a fault and/or a dike. Permeability in the upper zone is 10-11 m2 ; such permeabilities are reflected in the pressure increases and rises in water level during dike injection and eruption (Fig. 6.18). Pressure transients are not observed in the lower zone because the change is absorbed in the two-phase hydrothermal system.
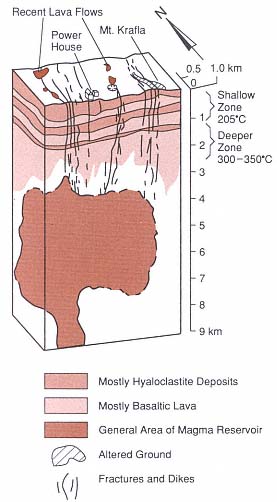
Fig. 6.17
Schematic cross-section of the Krafla geothermal
field and the underlying magma body
(Adapted from Stefánsson, 1981.)
Stefánsson (1981) noted that a severe mistake was made in constructing a power plant before the drilling was completed and the field was tested. As a result, the proposed maximum capacity of 35 MWe was not achieved. An eruption within the caldera also slowed construction work on the facility.
In Iceland, exploration techniques begin with detailed geologic mapping and dating of potential reservoir rocks. Determination of the eruption type is crucial; for example, by locating the vents and mapping facies within rocks erupted from a subglacial volcano, it is possible to evaluate not only the potential heat source, but also the location and extent of reservoir and caprocks. As noted earlier, pillow lavas are excellent reservoir rocks and the associated hyaloclastic carapaces are effective caprocks. All dikes and faults must be mapped for identification (or interpretation) of both thermal sources and potential aquicludes. After potential reservoir rocks have been evaluated at the surface, interpretative cross-sections can be prepared and evaluated by both geologist and hydrologist. An exploration drillhole can then be sited for further evaluation and temperature measurements. The primary targets in Iceland for direct-use purposes are highly permeable rocks that contain fluids with temperatures of > 100°C (Fridleifsson, 1978/79).
Geothermal Potential of Several Small Basaltic Islands
Surtsey
Much of what has been learned during the last 20 years concerning phreatomagmatic volcanism began with the mid-1960s submarine eruptions south of Iceland that eventually formed Surtsey, one of the Westmann Islands (Thorarinsson, 1965; 1966; 1967). The eruption, first noted on November 14, 1963, lasted more than 4 years and ended on June 5, 1967; this eruption formed an island of 2.8 km2 and an elevation of 174 m, which
is shown in Fig. 6.19. The early phases of activity were phreatomagmatic; much of the heat was lost in magma/sea water interactions that generated very energetic steam eruptions. The tephra deposited by these eruptions was barely warm to touch when was deposited as fallout and surges.
In later phases of activity, after a growing tuff ring denied the sea access to the vent, the main activity was lava fountaining, and lava flows that moved across the edges of the cone toward the sea. After activity ceased entirely, Stefánsson et al . (1985) drilled a 181-m-deep borehole on the cone at an elevation of 58 m. The tuff above sea level has a permeability of 1.2 × 10-10 m2 and the system within it is vapor-dominated. Below sea level, the estimated permeability of the basaltic tuffs is 4.1 × 10-13 m2 , and the tuffs are altered to palagonite (a mixture of smectite clays, zeolites, and iron oxides). No pillow lavas were encountered in this borehole, and the maximum temperature was 140°C at a depth of 104 m. Below this depth, near the contact between the sea floor and the base of the Surtsey cone, temperatures dropped to 40°C within altered tuffs that were cooled by sea water.
During the drilling operation, the borehole crossed a 13-m-thick dike at a depth of 80 m (Stefánsson et al ., 1985; Fig. 6.20). Most likely emplaced during the lava fountaining episodes late in the history of the cone, the dike can account for high heat flow within the cone. Fluids and heat from a tuff ring such as this one could be used for heating water but not for producing electricity; therefore, it is a limited, short-term resource unless the heat source were to be replenished by a new eruption.
Heimaey
Within sight of the new island of Surtsey is the small, populated island of Heimaey, which is an important Icelandic fishing community. On January 23, 1973, a north-north-east-trending fissure, located only 1 km east
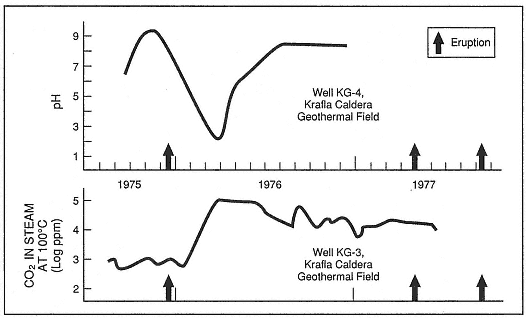
Fig. 6.18
Interaction of the Krafla hydrothermal system with rising magma. Changes in the CO2
concentration and pH within wells KG-3 and KG-4 correspond to renewed eruptive activity.
(Adapted from Stefánsson, 1981.)
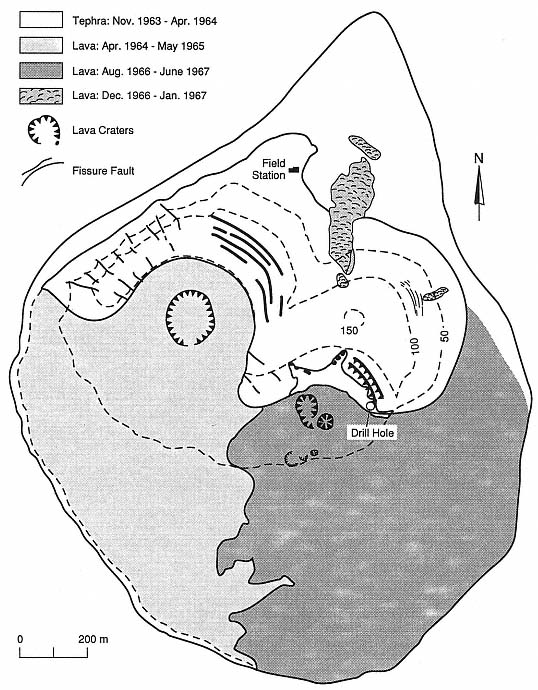
Fig. 6.19
Map of Surtsey volcano indicates the location of the 181-m-deep borehole.
(Adapted from Jakobsson and Moore, 1982.)
of the town center, opened over a length of 1200 m. Lava fountains occurred along the length of the fissure but were soon concentrated at one point (Williams and Moore, 1983). Within 2 days the lava fountaining had covered the island with ash and had constructed a 120-m-high scoria and spatter cone. Over the subsequent 2 weeks, lava fountaining decreased in intensity and a thick lava flow moved toward the edge of town. The 43- to 120-m-thick basaltic lava flow, at temperatures of 1030 to 1055°C, moved into the town and also threatened the harbor entrance.
To save the town and harbor, Icelandic officials were determined to stop the lava flow. Their method was to increase the lava's viscosity by spraying it with cold seawater and to construct a barrier along the flow margin. Seawater was sprayed onto the flow front and distributed across the flow surface at a rate of 1.7 m3 /s, cooling the flow to well below its solidus temperature. Barriers within the flow, which were formed by cooling, caused the flow to thicken. Over a 6-month period, the ~10 × 106 m3 of water sprayed onto the lava flows converted ~6.5 × 106 m3 of molten lava into hot, but solid rock (Jonsson and Matthiasson, 1974; Williams and Moore, 1983).
The eruption ceased June 23, 1973, leaving a lava flow ~1.5 by 1.5 km and ~100 m thick that was overlain by ~5 m of scoria. The residents of Heimaey immediately began to examine ways to take advantage of this heat source. A district heating system was created and after a successful prototype system was tested, construction of a geothermal heating system began. Four 100- by 100-m areas were developed, each consisting of steamwells in the unconsolidated scoria overlying the lava flow and an overlying network of pipes that spray water onto the ground surface. The water seeps into the scoria and the lava flow, is converted to steam, and rises to the steamwell collectors (Fig. 6.21). Each well produces 2.5 MWthermal during normal operations (Björnsson, 1980; Williams and Moore, 1983). By 1982, the entire town was heated by steam from the lava flow.